5. From Correlates to Theories of Consciousness
© David Gamez, CC BY 4.0 https://doi.org/10.11647/OBP.0107.05
5.1 Measurement of the Physical World
Randy is an elephant who lives at the bottom of my garden. Six blind men often come round to feel Randy. Sometimes I like to measure Randy. To measure his height, I compare my conscious experience of Randy with my conscious experience of a stick that has been calibrated against the distance light travels in a vacuum during 1⁄299,792,458 seconds.1 The ratio between Randy and the stick is his height in metres. Randy is three sticks (three metres) high (see Figure 5.1).2
The science of consciousness studies the relationship between consciousness and the physical world. It measures consciousness, measures spatiotemporal structures in the physical world and attempts to identify minimal sets of spatiotemporal structures (CC sets) that are linked to conscious states.
Physical objects do not directly appear in our bubbles of experience. We do not directly perceive their mass, chemical composition or size. To measure a property of a physical object I cause it to interact with another physical object that has been calibrated in some way and observe this interaction in my bubble of experience. This typically results in a number. Eddington describes this process:
Let us then examine the kind of knowledge which is handled by exact science. If we search the examination papers in physics and natural philosophy for the more intelligible questions we may come across one beginning something like this: “An elephant slides down a grassy hillside…” The experienced candidate knows that he need not pay much attention to this; it is only put in to give an impression of realism. He reads on: “The mass of the elephant is two tons.” Now we are getting down to business; the elephant fades out of the problem and a mass of two tons takes its place. What exactly is this two tons, the real subject matter of the problem? It refers to some property or condition which we vaguely describe as “ponderosity” occurring in a particular region of the external world. But we shall not get much further that way; the nature of the external world is inscrutable, and we shall only plunge into a quagmire of indescribable. Never mind what two tons refers to; what is it? How has it actually entered in so definite a way into our experience? Two tons is the reading of the pointer when the elephant was placed on a weighing-machine. Let us pass on. “The slope of the hill is 60°.” Now the hillside fades out of the problem and an angle of 60° takes its place. What is 60°? There is no need to struggle with mystical conceptions of direction; 60° is the reading of the plumb-line against the divisions of a protractor. Similarly for the other data of the problem.3
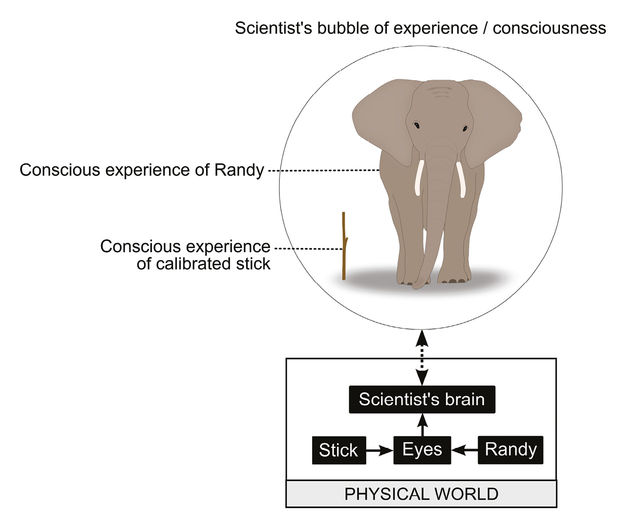
Figure 5.1. The measurement of an elephant’s height in a scientist’s bubble of experience. The scientist compares Randy with a stick that has been calibrated against the distance light travels in a vacuum during 1⁄299,792,458 seconds. The ratio between Randy and the calibrated stick is his height in metres. Randy is three sticks (three metres) high. Image © David Gamez, CC BY 4.0.
When I monitor Randy’s brain activity using electrodes, my equipment compares the effect of his brain on each electrode with the effect of a standard voltage: the ratio between these effects is the electrode’s voltage. A computer converts the electrode voltages into an attractive image of brain activity. Randy’s colourless physical brain does not directly appear to me when I am measuring it. Within my bubble of experience I am conscious of black wires emerging from a pinkish-grey brain and a 3D display of brain activity on a computer screen.
The physical world can be measured automatically without the instruments or measured objects appearing in a bubble of experience. A robot could measure Randy with a stick and write down the result on a piece of paper.
Measurements can be processed into numbers that correspond to different properties of an object. Electrode voltages can be processed into neuron firing events, which can be processed into firing frequencies, synchronization patterns, and so on.
Measurement assigns numbers to aspects of objects, properties or events.4 Objects, properties and events are typically described in natural language. When I measured Randy, 3 metres was the height of an elephant; 30 mV was the membrane potential of a neuron.
Objects, properties and events are tightly defined in physics and chemistry. For example, we have clear definitions of quarks and carbon. Physicists and chemists can state exactly what it means for a physical object to contain quarks or carbon; their instruments can reliably detect whether quarks or carbon are present in a physical object.
Context plays an important role in the description of biological objects, properties and events. Suppose I want to measure the membrane potential of a neuron. I do not use an abstract definition of a neuron to identify physical objects that are neurons—I look for a particular type of cell in the brain of an animal. The definition of a neuron only has to be precise enough to distinguish neurons from other cells in the brain. The context of a neuron (in the brain of an animal) is part of its definition.
A neuron is well defined inside a brain—but what exactly is a neuron? Does a neuron continue to be a neuron if I remove its nucleus, give it a chrome cytoskeleton and change its resting potential to 100 V? Synthetic biologists could construct a series of intermediate cases between neurons and liver cells—it would be difficult to classify the intermediate cases. Neurons are defined in a specific biological context; no formal definition exists that can unambiguously decide whether an arbitrary physical object is a neuron.
This vague definition of biological structures is a problem for consciousness science. We want to use what we know about consciousness in the brain to make inferences about the consciousness of non-biological systems. Suppose we identify neural correlates of consciousness and want to make inferences about the consciousness of synthetic neurons. This cannot be done without an unambiguous context-free definition of a neuron.
To address this problem we need formal ways of describing the spatiotemporal structures that form CC sets. These will be referred to as p-descriptions:
D7. A p-description is a formal description of a spatiotemporal structure in the physical world. A p-description unambiguously determines whether a spatiotemporal structure is present in a sequence of physical states.
When a spatiotemporal structure can be completely described by physics or chemistry (for example, an electromagnetic field or a molecule), the p-description is identical to the standard scientific description. We will have to find more formal context-free ways of describing biological structures that can resolve ambiguous cases. For example, we need a p-description that can determine whether an arbitrary part of the physical world contains neurons. This should not rely on the fact that neurons are found in biological creatures, and it should provide definite classifications of synthetic neurons, which lack some of the attributes of biological neurons. If the members of a CC set cannot be captured in a p-description, then we will only be able to make inferences about the consciousness of systems that are similar to platinum standards.5
5.2 Constraints on CC Sets
There are constraints on the spatiotemporal structures that can form CC sets. These derive from the assumptions that were introduced to measure consciousness (A1-A6) and from the requirements of scientific methodology:
C1. The spatiotemporal structures in a CC set are independent of the observer. My consciousness is a real phenomenon that does not depend on someone else’s subjective interpretation. CC sets must be formed from objective spatiotemporal structures, such as electromagnetic waves and neuron firing patterns.
C2. The members of CC sets are intrinsic properties.6 A conscious state supervenes on a CC set (A3a), so each duplicate of a CC set must be associated with an identical conscious state, regardless of the spatial and temporal context in which the duplicate appears.
C3. A non-conscious system does not contain a CC set that is 100% correlated with a conscious state.7 If A and B are 100% correlated, then A cannot occur without B. If a CC set is 100% correlated with a conscious state, then all brains that contain that CC set will be conscious.
C4. CC sets e-cause c-reports during consciousness experiments (A6).8 It is not necessary for every member of a CC set to e-cause c-reports. But some parts or aspects of the CC set must e-cause them. So when I say ‘I am conscious of a green tomato’, this c-report can be traced back to the CC set that e-caused it, which is functionally connected to a bubble of experience in which there is a green tomato.
A set of spatiotemporal structures that does not conform to these constraints cannot be a correlate of a conscious state.
5.3 Pilot Studies on the Correlates of Consciousness
We are in a beastly state of ignorance about the relationship between consciousness and the physical world. We have no idea which spatiotemporal structures form CC sets. Our intuitions are useless. We have to start with the assumption that everything in a platinum standard system that conforms to the constraints is a potential member of a CC set.
How can we reduce our ignorance? We can carry out pilot studies. We can attempt to identify the CC set that is associated with a particular conscious state.9
Briony is an adult human with a normally functioning brain. I strap her into a chair and connect electrodes to her temples. At intervals I display a red square at the centre of her visual field, play a loud 500Hz tone and deliver an electric shock. Briony’s attention is completely consumed by these stimuli. They are so compelling that the same conscious state can be induced on multiple occasions. This is conscious state c3.
Each time c3 is induced I ask Briony to c-report one aspect of it. On subsequent occasions she c-reports the size of the square, the colour of the tone and the shocking sensations in her body. Over time I build up a c-description of c3 that has a tolerable amount of detail.
I want to identify the minimal set of spatiotemporal structures that is correlated with c3. When c3 is induced I measure the neuron activity in Briony’s brain as well as the electromagnetic waves, blood movements, glia activity, and so on.10 Some of these spatiotemporal structures could form the CC set by themselves—a pattern of neuron activity might be the sole correlate of c3. Or a combination of spatiotemporal structures might form the CC set that is correlated with c3. For example, a pattern of neuron activity might only be associated with consciousness when it is immersed in blood—the same neuron activity without blood would not be linked to consciousness.
I must systematically consider all possible combinations of spatiotemporal structures that could form the CC set. This will enable me to identify the spatiotemporal structures that only occur when c3 is present. Suppose I want to demonstrate that a pattern of neuron activity, p2, is the sole member of the CC set. I will need to measure c3 when p2 is present and blood is present, measure c3 when p2 is present and blood is absent, measure c3 when just blood is present, and measure c3 when neither p2 nor blood are present. Further experiments will be required to distinguish p2 from glia activity, cerebrospinal fluid, and so on. This methodology is illustrated in Table 5.1.11
Spatiotemporal Structures |
Conscious States |
||||
A |
B |
C |
D |
c1 |
c2 |
0 |
0 |
0 |
0 |
0 |
0 |
0 |
0 |
0 |
1 |
0 |
0 |
0 |
0 |
1 |
0 |
0 |
1 |
0 |
0 |
1 |
1 |
0 |
1 |
0 |
1 |
0 |
0 |
0 |
0 |
0 |
1 |
0 |
1 |
0 |
0 |
0 |
1 |
1 |
0 |
0 |
1 |
0 |
1 |
1 |
1 |
0 |
1 |
1 |
0 |
0 |
0 |
0 |
0 |
1 |
0 |
0 |
1 |
0 |
0 |
1 |
0 |
1 |
0 |
0 |
1 |
1 |
0 |
1 |
1 |
0 |
1 |
1 |
1 |
0 |
0 |
1 |
0 |
1 |
1 |
0 |
1 |
1 |
0 |
1 |
1 |
1 |
0 |
1 |
1 |
1 |
1 |
1 |
1 |
1 |
1 |
Table 5.1. Simple example of correlations that could exist between spatiotemporal structures in a physical system and two conscious states. It is assumed that conscious states c1 and c2 can occur simultaneously. The physical structures A, B, C and D could be dopamine, haemoglobin, neural synchronization, electromagnetic waves, etc. These are assumed to be the only possible features of the system. ‘1’ indicates that a feature is present; ‘0’ indicates that it is absent. In this example D is not a correlate of consciousness because it does not systematically co-vary with either of the conscious states. {A,B} is a set of spatiotemporal structures that correlates with conscious state c1. {C} is a set of spatiotemporal structures that correlates with conscious state c2.
Many pilot studies have been carried out on the correlates of consciousness. They have identified areas of the brain and features of neuron activity (for example, recurrent connections) that are potential members of CC sets.12 Most of these pilot studies have focused on neural patterns that might form CC sets. No attempt has been made to show that neuron activity patterns form CC sets by themselves, or to demonstrate that glia, electromagnetic waves and haemoglobin are not members of CC sets.
5.4 Natural and Unnatural Experiments
Consciousness experiments are carried out on platinum standard systems. Assumptions A1-A6 enable us to measure the consciousness of platinum standard systems during these experiments.
Normally functioning adult human brains are our only platinum standard systems (A4). They change as they interact with the world and learn from their experiences. Most of these changes are part of their normal behaviour—they do not affect their status as platinum standards.
Brian’s skull contains a normally functioning adult human brain (a platinum standard system). I ask him to raise his right arm. He raises his right arm. I shave off his hair and slowly smear chocolate sauce on his face. These modifications do not affect the normal functioning of his adult human brain.
I inject Brian with 5 mg of LSD. After a brief spell of bliss he goes wild, bangs his head against the wall, yells in an uncontrollable manner and claws at his face. His brain is not functioning normally. I shoot him in the head. He lies still on the laboratory floor. Blood pours out of his head. His brain is no longer a platinum standard system.
A platinum standard system must remain a platinum standard system throughout an experiment on consciousness. If it ceases to be a platinum standard system, then assumptions A1-A6 no longer hold and it becomes an open question whether we can interpret its external behaviour as a c-report of its consciousness.
Some experiments preserve a system’s status as a platinum standard; other experiments transform a system into something that is not a platinum standard. This distinction will be expressed as follows:
D8. In a natural experiment the test system preserves its status as a platinum standard. Assumptions A1-A6 remain valid and consciousness can be measured throughout the experiment.
D9. In an unnatural experiment the test system is transformed into something that is not a platinum standard. A1-A6 cease to apply and we lose our ability to measure the system’s consciousness.
Natural experiments preserve a system’s physical integrity and normal behaviour. The system can be monitored using passive techniques, such as fMRI, EEG and electrodes.13 These manipulations do not affect our belief that it can c-report its consciousness.
Unnatural experiments alter the physical constitution of a platinum standard system. They remove material, add unusual chemicals or replace brain parts with functionally equivalent chips. Unnatural experiments undermine our ability to measure a system’s consciousness. They cannot be used to identify CC sets or to test theories of consciousness.
Suppose we replace part of a subject’s brain with a functionally equivalent chip. This would not affect their behaviour—they would continue to make the same reports as before. This experiment has been put forward as a way of testing the hypothesis that functions or computations in the brain are linked to consciousness, rather than patterns in biological materials.14
Prior to the experiment the subject’s brain was a platinum standard system and we interpreted its speech as a c-report of its conscious states. The implantation of the chip transforms the subject’s brain into a freak neuro-silicon hybrid that is not a platinum standard system. We have no idea whether brains with implanted chips are associated with consciousness. Assumptions A1-A6 do not apply—we have not assumed that the external behaviour of this type of system is a c-report that can be used to measure consciousness. Similar problems occur with other unnatural experiments, such as the replacement of haemoglobin with an artificial blood substitute, the removal of glia, and so on.15,16
We could add brains with implanted chips to our list of platinum standard systems. This would transform an unnatural chip implantation experiment into a natural experiment. Both the original system and the transformed system would be platinum standards, so we could measure consciousness throughout the experiment.
New assumptions about platinum standard systems should not be made lightly. Pilot studies look for CC sets in the systems that are assumed to be platinum standards. A science of consciousness that studied brains with implanted chips would be very different from our current science of consciousness.17
It will be difficult or impossible to identify all the members of a CC set using natural experiments. The members of a CC set can only be identified by systematically varying the physical world to test the link between each combination of candidate structures and consciousness (see Table 5.1). When a combination does not occur naturally it is impossible to test its link with consciousness. So natural experiments cannot test the connection between consciousness and biological neurons, because we cannot remove biological neurons from the brain without compromising its status as a platinum standard system.
5.5 Theories of Consciousness (C-Theories)
It is possible to interpret the ways of science more prosaically. One might say that progress can ‘… come about in only two ways: by gathering new perceptual experiences, and by better organizing those which are available already’. But this description of scientific progress, although not actually wrong, seems to miss the point. It is too reminiscent of Bacon’s induction: too suggestive of his industrious gathering of the ‘countless grapes, ripe and in season’, from which he expects the wine of science to flow: of his myth of a scientific method that starts from observation and experiment and then proceeds to theories […] The advance of science is not due to the fact that more and more perceptual experiences accumulate in the course of time. […] Bold ideas, unjustified anticipations, and speculative thought, are our only means for interpreting nature: our only organon, our only instrument, for grasping her.
Karl Popper, The Logic of Scientific Discovery18
What’s the matter with consciousness, then, and how should we proceed? Early on, I came to the conclusion that a genuine understanding of consciousness is possible only if empirical studies are complemented by a theoretical analysis. […] This state of affairs is not unlike the one faced by biologists when, knowing a great deal about similarities and differences between species, fossil remains, and breeding practices, they still lacked a theory of how evolution might occur. What was needed, then as now, were not just more facts, but a theoretical framework that could make sense of them.
Giulio Tononi, Consciousness as
Integrated Information: A Provisional Manifesto19
When people studied the heavens they were not seeking an infinitely long list of the planets’ positions. They wanted a compact theory that could calculate the positions of the planets at an arbitrary point in time. Ptolemy developed a model based on deferents and epicycles. This was superseded by Newton’s and Einstein’s equations.
Pilot studies might identify the correlates of some conscious states. This would be a major scientific achievement. It would help us to find the correlates of other conscious states. It would tell us something about the consciousness of non-platinum standard systems, such as coma patients, bats and robots.
The wine of a science of consciousness will not flow from industrious gathering of data about the correlates of individual conscious states. There are an effectively infinite number of conscious states—we cannot identify the CC sets associated with each one. Instead we need a compact mathematical theory that can map physical states onto conscious states and vice versa. This will be referred to as a c-theory:20
D10. A c-theory is a compact expression of the relationship between consciousness and the physical world. A c-theory can generate a c-description from a p-description, and generate a p-description from a c-description.21
The role of c-theories is illustrated in Figure 5.2.
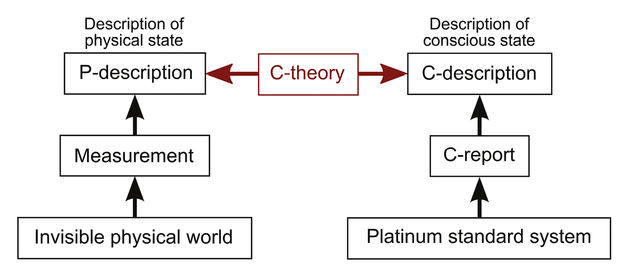
Figure 5.2. Theory of consciousness (c-theory). On the left, a measurement of the invisible physical world is converted into a formal p-description of a physical state. On the right, consciousness is measured with a c-report, which is converted into a formal c-description of a conscious state. The c-theory maps between the p-description and the c-description. Image © David Gamez, CC BY 4.0.
C-theories specify which types of spatiotemporal structures form CC sets. For example, neuron activity patterns, information patterns or computations might be members of CC sets. The most popular types of c-theory are covered in the next three chapters.
C-theories should be based on mathematics.22 This is the most compact way of linking p-descriptions to c-descriptions. Philosophical theories of consciousness might inspire c-theories. But the relationship between c-descriptions and p-descriptions cannot be expressed in natural language. Natural language is too weak and vague—it cannot make strong testable predictions.
Suppose we discover a neuron whose firing rate is correlated with a bubble of experience in which there is a single point of red light. We develop a c-theory that uses the equation ln(r)=2i to connect the neuron’s firing rate, r, with the intensity of the conscious red light, i. This theory predicts that when the neuron fires at 7 Hz it will be associated with conscious red light that has intensity 0.97. It also predicts that the neuron will fire at 20 Hz when conscious red light occurs with intensity 1.5.23
C-theories map between conscious states and sets of spatiotemporal structures in the physical world. These spatiotemporal structures must be valid members of CC sets. So the constraints on the members of CC sets (Section 5.2) are constraints on c-theories. C-theories must generate p-descriptions of valid CC sets from c-descriptions, and they must generate c-descriptions from p-descriptions of valid CC sets. C-theories that do not conform to the constraints should be excluded from the science of consciousness.
C-theories become scientifically credible when their predictions pass experimental tests. It is not enough for c-theories to match data gathered during pilot studies—they have to generate strong predictions that can be experimentally confirmed. The most compact and accurate c-theory will be considered to be a correct description of the relationship between consciousness and the physical world.24
Our final c-theories will describe brute regularities in the relationship between consciousness and the physical world (see Section 3.5). As the science of consciousness progresses we are likely to develop c-theories that describe regularities which can be further decomposed into more basic relationships. It might be impossible to tell whether a c-theory describes a genuine brute regularity.25
Some people base c-theories on their conscious experiences.26 But the source of inspiration of a c-theory is irrelevant to its success. We cannot directly imagine the relationship between consciousness and the invisible physical world (see Section 3.3), so the intuitive plausibility of a c-theory has no bearing on whether it is correct. C-theories stand or fall on their ability to make falsifiable predictions that pass experimental tests.
C-theories are not likely to provide intuitively satisfying explanations of the relationship between consciousness and the physical world. Since we cannot imagine the physical world, a mathematical c-theory cannot help us to make an imaginative transition from the invisible physical world to consciousness. At most a c-theory could help us to make an imaginative transition from a conscious experience of brain activity to another conscious experience (see Section 3.4).
People might use particles, forces or novel aspects of the physical world to explain why a particular relationship between c-descriptions and p-descriptions holds (see Section 6.3). Such explanations might be scientifically fruitful—they might help us to develop new mathematical c-theories. But they are unlikely to make a c-theory more intuitively plausible. Newton could not imagine how gravity acted at a distance. We cannot explain why brute regularities exist between consciousness and the physical world.
5.6 The Computational Discovery of Theories of Consciousness
[…] the era of simple mathematics effectively modelling parts of the world is drawing to a close. It is possible that new areas of investigation will lend themselves to simple models, but the evidence is that within existing areas of investigation, the domain of simple models has been extensively mined to the point where the rewards are slim.
Paul Humphreys, Extending Ourselves27
Traditional science is based on the idea that people identify regularities in the physical world. It is a working assumption that physical regularities are simple enough to be found by humans. When a human finds a regularity s/he might use a novel property to explain it. A mathematical description of the regularity can be experimentally tested. Humans find this satisfying—they like solving puzzles. But it is not necessarily the most effective approach.
Humans are biased and stupid. They have small working memories and little imagination. They cannot process large data sets. These limitations will prove fatal to consciousness science if there are complex relationships between c-descriptions and p-descriptions.28
We have little or no idea about the spatiotemporal structures that form CC sets. The mathematical relationships between c-descriptions and p-descriptions are unknown. They might be simple—a few differential equations. Or the mathematical complexity of these regularities could be way out of reach of human capabilities. Macro-scale laws of the brain could extend to thousands of pages of differential equations.
We should drop the assumption that there are simple relationships between p-descriptions and c-descriptions. We have no reason to believe that this is the case. If we persist with the assumption that there are simple relationships, we could spend large amounts of time and money on a fruitless quest for something that does not exist. It is better to assume that the relationships between c-descriptions and p-descriptions are potentially complex, and develop a methodology that can identify simple and complex relationships (or prove that no relationships exist).
We could use computers to identify the relationships between p-descriptions and c-descriptions. This would require a large amount of data, spanning multiple levels of the brain. C-descriptions and p-descriptions would have to be recorded for many different conscious states. This data could be gathered by human scientists. Or robots could capture it automatically.29
Machine-learning techniques could be applied to this data. The patterns that were found could be used to make predictions, which could be tested in further experiments. C-theories could be automatically tested against new data as it came in.
C-theories that are discovered by computers should be expressed in a format that can be read by both humans and machines (they should not be stored as weights in a complex neural network). This would enable them to be partly viewed and verified by humans—but there would be no expectation that an individual human scientist could check or comprehend them in their entirety. Sets of differential equations would be a good choice of output format—there is a long tradition of using differential equations to describe complex relationships in the physical world. Or perhaps we could use graph theory to describe the relationships between c-descriptions and p-descriptions.30
This computational approach to the science of consciousness could identify simple relationships between c-descriptions and p-descriptions. It could find regularities that are too complex to be identified by humans. Or it could prove that no simple or complex laws exist in the current data.
This approach could be prototyped on a simulated human brain.31 This would generate reports ‘about consciousness’ that are similar to c-reports and could be converted into c-descriptions.32 The computer could search for relationships between these c-descriptions and different aspects of the neural model. It could control the simulation (rewinding it, rewiring it, changing its parameters) to robustly test its hypotheses.33 The relationships between c-descriptions and p-descriptions that were identified by this method could be tested on platinum standard systems.34
5.7 Summary
This chapter has described how we measure the physical world. Physical measurements have to be expressed in a formal way (a p-description), so that we can use our knowledge about CC sets in humans to make inferences about the consciousness of non-biological systems.
Pilot studies could identify the CC sets that are linked to individual conscious states. These must use natural experimental methods, which preserve our ability to measure consciousness in platinum standard systems.
In the longer term we need to develop mathematical c-theories that map between p-descriptions and c-descriptions (see Figure 5.2). These c-theories must conform to the constraints on CC sets (C1-C4).
Humans might be incapable of discovering complex mathematical relationships between p-descriptions and c-descriptions. To avoid this potential problem, computers should be used to discover c-theories.