11. Preventing Extinctions
© 2019 J.W. Wilson and R.B. Primack, CC BY 4.0 https://doi.org/10.11647/OBP.0177.11
11.1 Studying Species and Populations p. 376
11.2 Saving Species Through Translocations p. 384
11.3 Managing and Facilitating Movement Dynamics p. 395
11.4 Managing Species Sensitive to Climate Change p. 404
11.5 Ex Situ Conservation Strategies p. 406
11.6 Thoughts on Neglected Taxa p. 413
11.8 Topics for Discussion p. 418
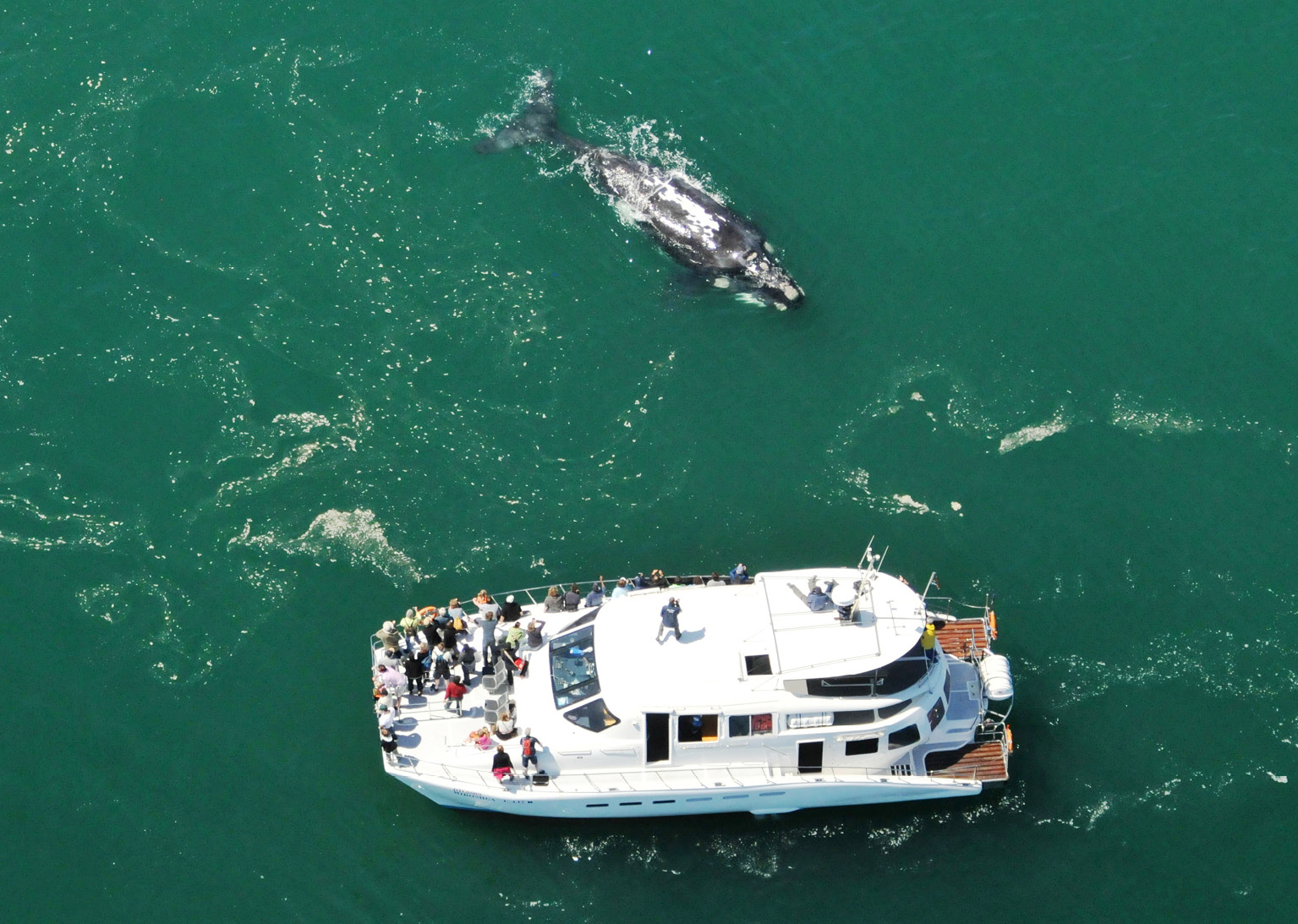
Tourists appreciating a southern right whale (Eubalaena australis, LC) from a whale charter boat operating from Hermanus, South Africa. Considered the “right” whale by 19th century whalers, the species was hunted to near extinction by the early 1900s, when only 300 individuals were left in the world (Reilly et al., 2013). Following the international ban on whale hunting, their populations have steadily recovered. Today it is considered safe from extinction, enabling towns such as Hermanus to base their thriving tourism industry on whale watching. Photograph by Southern Right Charters, CC BY 4.0.
There are many examples in this textbook illustrating how species have been saved from the brink of extinction. For some, the solution was simple: halt the threats that caused their populations to decline. In other cases, more drastic steps were required, like moving the last remaining individuals into captivity until the threats have been reversed. Many species that persist with low population sizes would likely not have survived without human intervention (Figure 11.1).
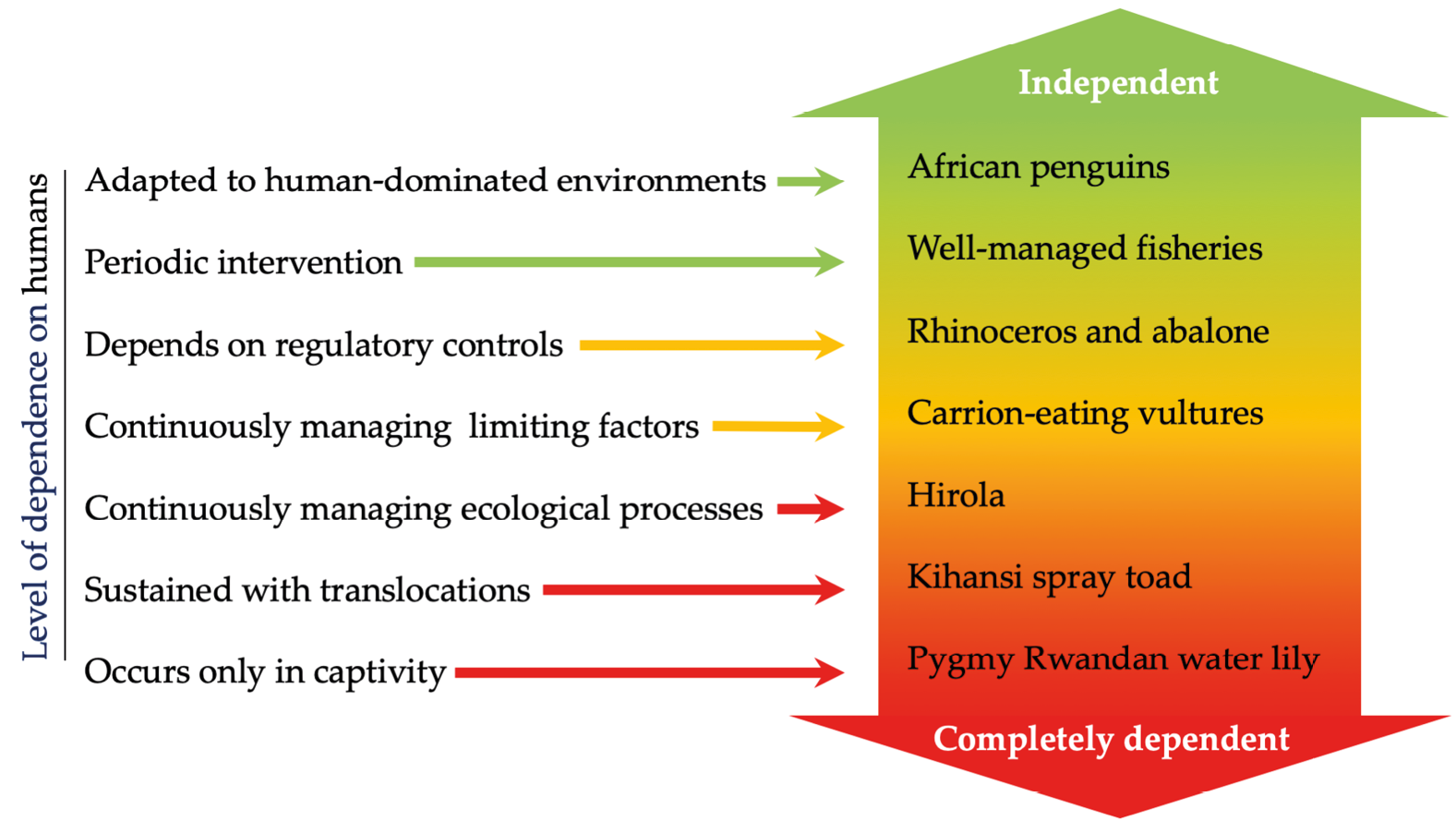
Figure 11.1 The continuum of species management approaches. Some threatened species exist under such low population sizes that they depend on active human intervention for recovery, while others can persist with minimal intervention. Each of the examples have been discussed elsewhere in the book. After Scott et al., 2005, CC BY 4.0.
In each of these success stories, the most important first steps involved determining the ecological needs of the species at risk and understanding the factors that made that species vulnerable to extinction (Section 8.5). This chapter reviews some of the most important concepts for understanding and managing those needs and risks. The concepts reviewed in this chapter include methods to study species and populations, actions that can be taken to increase population sizes, and strategies that can help maintain evolutionary processes such as genetic exchange. This chapter also considers how to manage for climate change and discuss the importance of ex situ conservation strategies.
11.1 Studying Species and Populations
To save a species from extinction, it is vital to have a firm grasp on the species’ distinctive characters, in other words its natural history. To obtain this natural history information, 10 important factors need to be considered:
- Population biology: How many individuals are there in the population? How many males, females, juveniles, breeding adults, and individuals past breeding age are there? What is the species’ life expectancy? How have these aspects changed over time? (see also Chapter 9)
- Habitat: In what kind of environment can the species be found? How do these ecosystems change over time and space? Does the species have a complex life history that requires multiple habitats (e.g. frogs that live on land generally need water for breeding)? What factors are important to maintain suitable habitat?
- Distribution: Where in the world can the species of concern be found? At what rate is its distribution increasing/decreasing? What factors drive these increases/decreases?
- Morphology: What are the defining traits, or range of traits, of the species’ appearance? How do the species’ unique morphological characteristics help it survive? Are there closely-related species that appear similar (i.e. cryptic species) and with which it can be misidentified?
- Limiting resources: What types of resources does the species need to survive? Are any of these resources in short supply? Does the distribution of these important resources change over time and space?
- Physiology: Are there any special requirements the species’ physical and biochemical processes need for it to grow, survive, and reproduce? What are the conditions under which meeting these requirements is especially hard?
- Behaviour: How do individuals act or behave (Box 11.1)? Is the species sedentary, nomadic, or migratory? Do individuals group together, disperse at random throughout landscapes, or space themselves out at regular distances? How do these behaviours help it survive?
- Genetics: How much do genes vary within the species? How are the species’ genetics linked to its morphology, physiology, and behaviour? Are there local genetic adaptations? Is the genetic variation in key traits sufficient to allow the species to adapt to environmental changes? Are there any deleterious genetic concerns? (Section 8.7.1)
- Biological interactions: In what ways do individuals of the species interact with each other and with other species? Which of these interactions are critical for survival? Are there any competitors, predators, parasites, or diseases affecting the species?
- Interactions with humans: How sensitive is the species to human activity? Do humans use the species in any way? Is the species sustainably harvested? Is the species associated with human-wildlife conflict (Section 14.4)?
Box 11.1 The Overlooked Role of Behavioural Ecology in the Conservation of African Mammals
Mammal Research Institute,
Department of Zoology and Entomology,
University of Pretoria, South Africa.
adrian.shrader@up.ac.za
When considering the management and conservation of wild animals, topics linked to population and community ecology (e.g. carrying capacity, Hayward et al., 2007a) often come to mind. This is not surprising, as these disciplines consider broad patterns of population dynamics (e.g. birth rates and mortality rates), which are key to achieving management and conservation goals. While this information is necessary, in many instances, it fails to explain the mechanisms behind the patterns observed and answer key questions. For example, why do species prefer specific habitats? Why do some herbivores adjust their home ranges with the seasons? To answer these sorts of questions, we need to understand an animal’s behavioural ecology.
Take for example the challenge of understanding the impacts that elephants cause within protected areas. A standard way to assess these impacts is to record which tree species are damaged and how many trees are affected (e.g. broken branches, bark stripping) (Boundja and Midgley, 2010). While this provides information on the trees most vulnerable to elephant damage, it does not explain why elephants are damaging the trees. Is it because the trees are a key part of the elephants’ diet, or are these trees just abundant across the landscape and in the way of a moving herd? To answer these questions, we turn to behavioural ecology. By observing foraging elephants, or by walking down their feeding paths after they have left, we can determine the animals’ diet, and generate an acceptability index (number eaten ÷ number available) of each tree species (Shrader et al., 2012). These data allow us to better understand the reasons behind elephant damage.
Other situations where behavioural ecology can help include reintroductions, population management, and human-animal conflict mitigation. For example, in South Africa, oribi are locally threatened by habitat loss and poaching. One conservation strategy is to relocate individuals away from known threats. Oribi are grassland specialists (Figure 11.A) that require both short and tall grasslands—therefore, release sites require a mosaic of these habitats. Moreover, within grasslands oribi perceive woodland patches to be dangerous, and tend to avoid feeding within 15 m of them (Stears and Shrader, 2015). If we do not consider how oribi utilise their environment, our estimate of available habitat at a release site may be greater than the area utilised. This mistake could reduce relocation success.
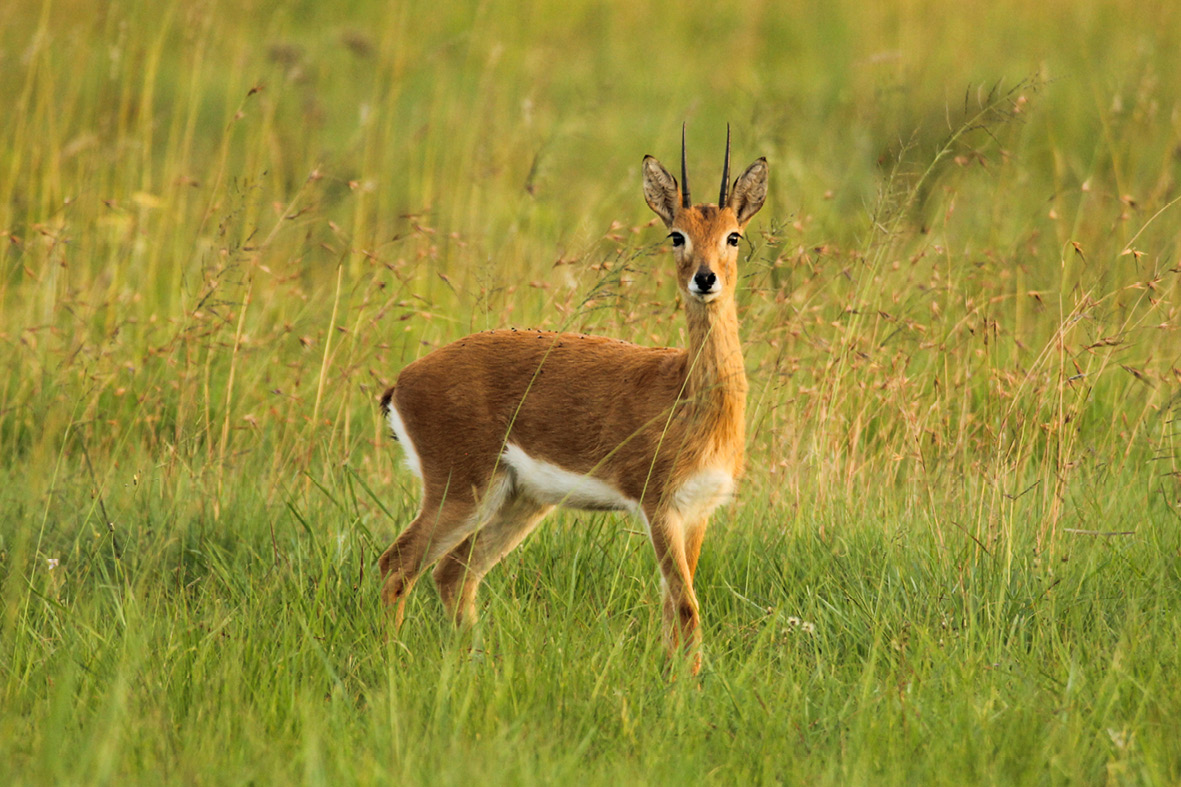
Figure 11.A The oribi is a grassland specialist that requires both short and tall grasslands and tends to forage at least 15 m from wooded patches. Photograph by K. Stears, CC BY 4.0.
With regards to population management, behavioural ecology is central to the conservation of southern white rhinoceros (Ceratotherium simum simum, NT) in the Hluhluwe-iMfolozi Park, South Africa. Within the park, the management policy incorporates space use and social ecology of the rhinos to facilitate population regulation (i.e. dispersal). To do this, the population can grow in the central core of the park. When rhino numbers get too high in the core, individuals naturally disperse into surrounding low-density areas, at which point they are captured by wildlife officers and transported to other areas. Thus, rhino behaviour itself is used to indicate when there are too many individuals within the fenced park (Linklater and Shrader, 2017).
Finally, behavioural ecology has helped reduce human-elephant conflict through the understanding that elephants are afraid of bees and will avoid feeding close to them. To capitalise on this fear, fences that incorporate beehives were designed and constructed around agricultural fields in northern Kenya, which helped reduce crop damage from raiding elephants. Of 32 raids recorded in the area, only one was at a farm with a beehive fence (King et al., 2011). These examples showcase how behavioural ecology can support, expand, and strengthen management and conservation of wildlife. These same principles can be applied to protect a wide range of animals across Africa, and elsewhere.
Understanding the natural history of a species directly informs conservation strategies. For example, if we know where a species occurs and what its habitat needs are, we are in a better position to prioritise which areas need to be protected or how ecosystems need to be restored. Similarly, if we know that an important food resource is missing, perhaps during a drought or due to human activities, conservationists could provide supplemental feeding until the limiting resource has recovered (Figure 11.2). Depending on the species in question, some factors play a more important role than others. For example, managing a disease outbreak may play a more important role in the conservation of a widespread migratory bird (that can spread diseases to other species), while managing for genetic diversity may play a more prominent role in the conservation of a small population of fishes restricted to only one lake. For many widespread species, different factors affect different subpopulations. In such cases each subpopulation might need to be managed as its own evolutionary significant unit (ESU; see e.g. Dubach et al., 2013) to retain unique local adaptations and genetic markers.
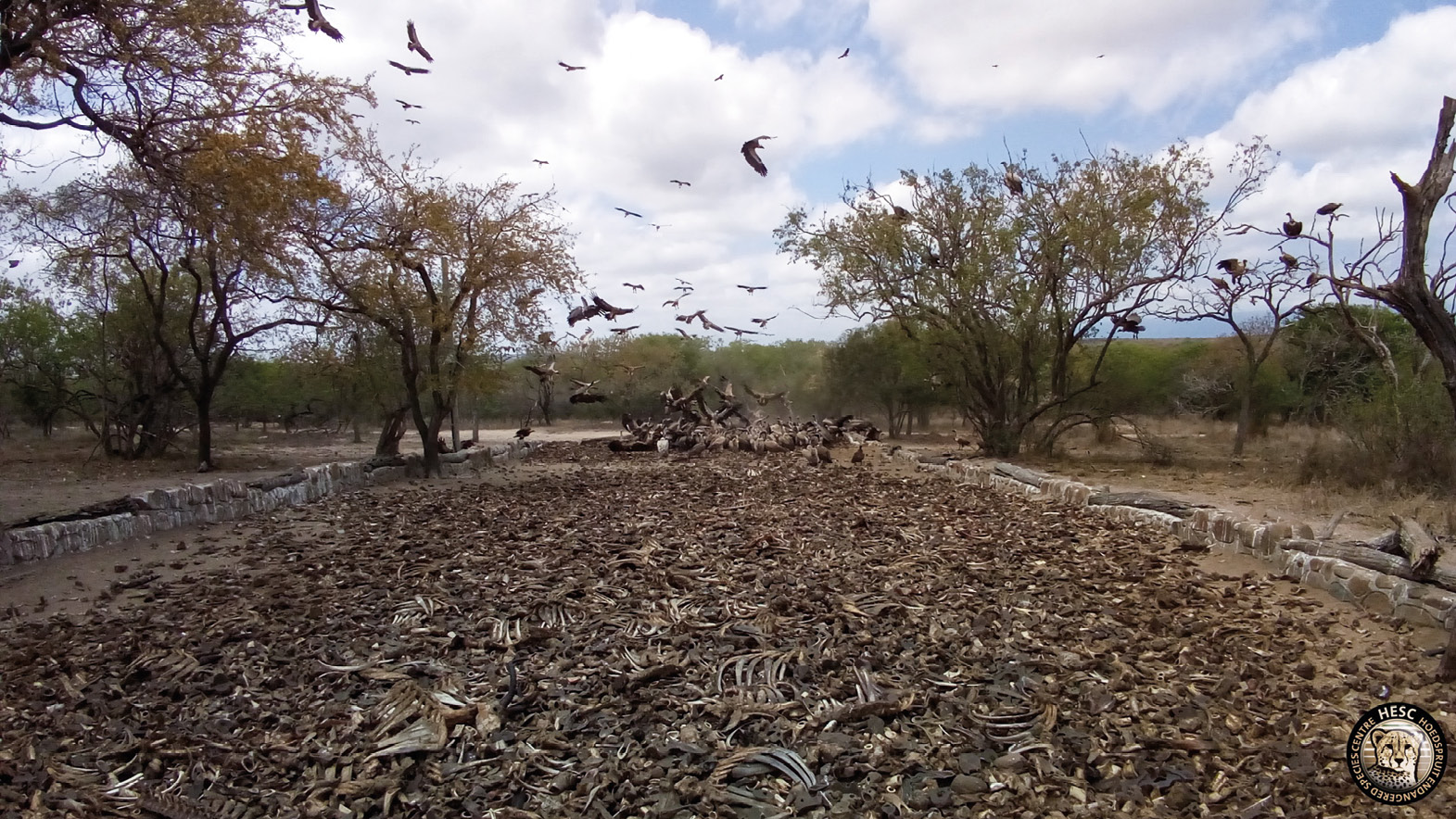
Figure 11.2 In some areas where diminishing food supplies threaten vulture populations, conservationists are supplementing their diets by placing carcasses at “vulture restaurants”. These vulture restaurants often depend on cooperation with local farmers who donate livestock that have died. Photograph by Hoedspruit Endangered Species Centre, CC BY 4.0.
11.1.1 Obtaining natural history data
Conservationists rely on several resources and techniques to obtain natural history information. Initial steps often involve reviewing published and unpublished literature to understand what is known (and not known) about a species. Literature reviews do have some drawbacks: they can take a long time, may uncover contradictory information, and may lack critical information relevant to a local area or specific population. For this reason, and especially when decisions need to be made under tight schedules, conservation biologists may need to speed up their initial species review by sourcing natural history information from subject matter experts who are familiar with the species or ecosystem of concern.
Conservation biologists are increasingly recognising the importance of traditional ecological knowledge—detailed insights that rural people have on the species around them.
Conservation biologists are also increasingly recognising the importance of traditional ecological knowledge (TEK)—detailed insights that rural people have on the ecology, behaviour, and distribution of the species around where they live (Shackeroff and Campbell, 2007; Brook and McLachlan, 2008). For example, while termites are often considered a pest by people living in urban settings, scientists are increasingly relying on TEK to understand the important contributions of termites to food security to human health, as well as to learn about ecological sustainable methods for their control when needed (Sileshi et al., 2009).
While literature reviews, expert opinions, and traditional ecological knowledge are important first steps to collect natural history information, the most reliable method remains fieldwork, where multiple individuals from the population of concern in the area of interest are observed repeatedly over time. Indeed, most of natural history information we have today was obtained during detailed notetaking by naturalists—biologists who dedicate much of their time to better understand the natural world—in the field.
Unfortunately, there are still major gaps in our understanding of the living world. Consequently, a very large number of threatened species, including better-known groups (e.g. reptiles, Tolley et al., 2016), lack the kinds of data necessary to ensure that we can give them the best chance of survival. Filling these gaps is also becoming harder since it is costly and sometimes logistically impossible (or dangerous) for naturalists to spend an extended period in the field. There is also a trade-off in the breadth and depth of data collection possible: the more area one covers, the less detailed the data; conversely, when one collects more detailed data, the scope of the study is reined in for logistical constraints. Further, there is also a limit to the number of organisms any one individual observer can study at any one time.
Recent technological advances have greatly increased our ability to overcome the logistical constraints that impede conservation fieldwork. One of the most useful developments involves the miniaturisation (and reduced costs) of animal-borne biologging devices, such as radio telemetry and GPS tags (Kays et al., 2015). Previously reserved for projects with large grants that focused on large animals, the big clunky devices of a few decades ago have made way for devices small enough to fit comfortably on animals as small as beetles and frogs. Some biologging devices are now also solar-powered and transmit data through Earth observation satellites in real time, allowing researchers to track the behaviours of several organisms at a time from the comfort of their offices. Even better, some tracking technologies also collect environmental data and movement data simultaneously, allowing us to better understand how wildlife responds to changing environmental conditions. These new and sophisticated datasets can then be used to better understand threats to species (e.g. Scantlebury et al., 2014; Childress et al., 2016) and inform management of protected areas (e.g. Maxwell et al., 2011).
Species distribution modelling (SDM), also known as environmental niche modelling, is becoming increasingly popular for determining a species’ distribution and habitat needs. SDMs overlay species location data, obtained during field work or using biologging devices, onto a selection of relevant environmental variables (e.g. forest cover, elevation, soil type) using GIS software, after which special modelling algorithms estimate the species’ ecological niche and distribution (Figure 11.3, see also Figure 10.3). This information enables conservation biologists to identify previously unknown habitat patches (which may represent undiscovered and unprotected populations) or empty habitats (which may be used in translocations, see Section 11.2). The appeal of SDMs lies in the availability of user-friendly software packages that can use very limited datasets. For example, one study from West Africa successfully combined market survey data and SDM to determine the potential for sustainable extraction of 12 medicinal plant species (van Andel et al., 2015). Another study used SDMs to develop a holistic picture of diversity and endemism patterns of nearly all 250 African bat species (Herkt et al., 2016). While distribution modelling offers very useful conservation tools, it is important to learn about the different techniques under the guidance of an expert to avoid making costly mistakes (McPherson et al., 2006; Pearson et al., 2006).
Species distribution modelling, also known as environmental niche modelling, is becoming increasingly popular for determining a species’ distribution and habitat needs.
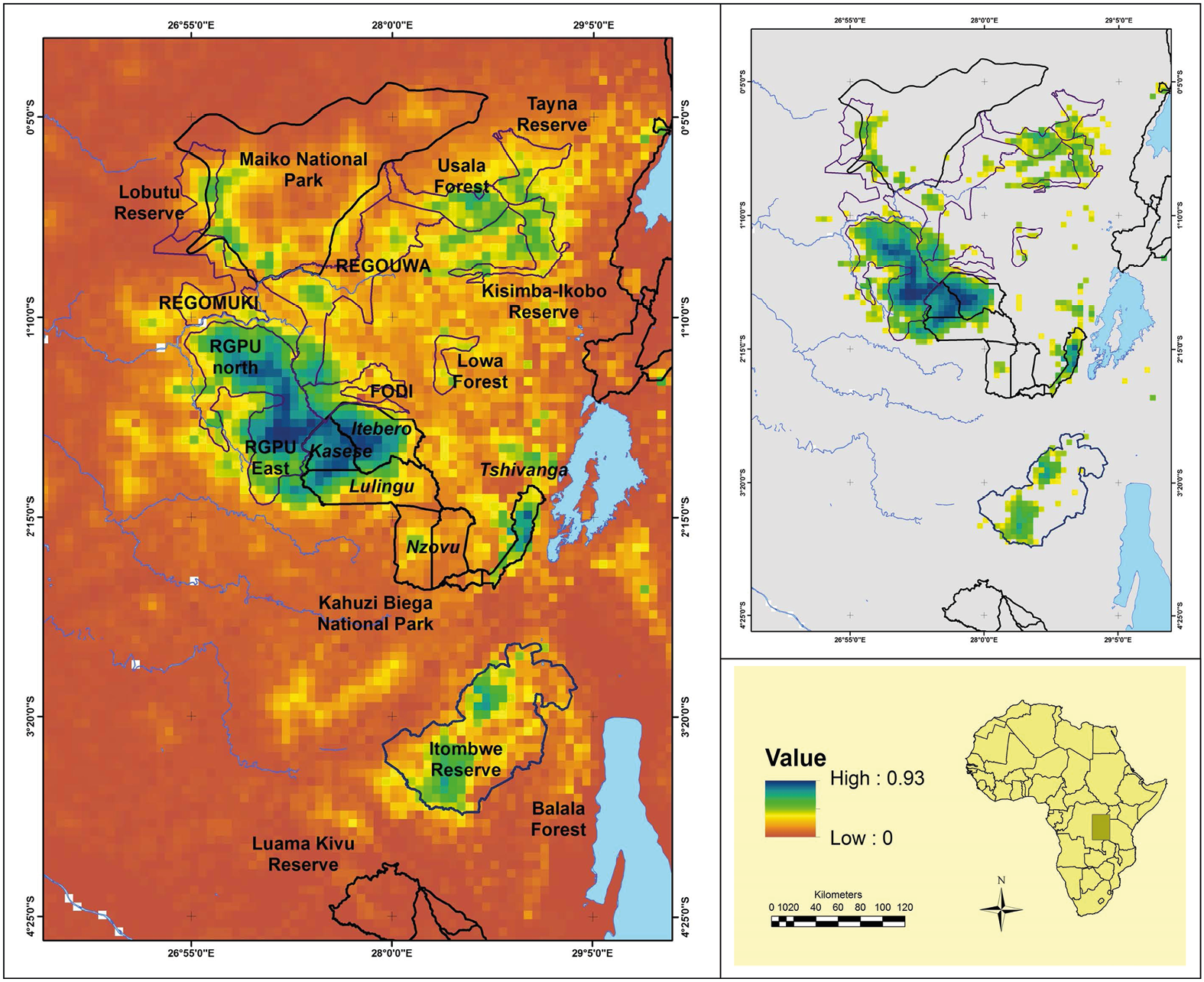
Figure 11.3 A species distribution model over the global range of the Grauer’s gorilla. Purple and green areas indicate potentially suitable habitat while yellow and red areas indicate unsuitable habitat. The analysis highlighted that the gorilla is found in high-altitude forests far from deforestation activity. The map also shows which areas should be safeguarded to secure the species’ survival. Source: Plumptre et al., 2016, CC BY 4.0.
Experimentation offers powerful methods to better understand competing theories and hypotheses, and to gain insight into how specific management actions may influence population dynamics. Experimentation is often associated with controlled environments such as laboratories; however, this is often impossible and sometimes even unethical to perform laboratory experiments on threatened species. Instead, conservation researchers may opt for natural experiments, which allows for the target species or population to be studied in its natural ecosystem.
A chronosequence study is a special type of natural experiment that overcome the long-term commitment some studies require to attain meaningful results. Also called space-for-time experiments, chronosequence studies allow us to infer long-term trends over a short study period using study systems that share similar qualities but are differently aged. Chronosequence studies are particularly popular when studying ecological restoration projects (Section 10.3) since some ecological processes often require many decades to develop (Bonnell et al., 2011). In one such example, conservation biologists needed only three summers worth of vegetation surveys to show that some species recolonise coastal dune forests in the Maputaland-Podoland-Albany Biodiversity Hotspot only after 100 years since disturbance (Wassenaar et al., 2005).
Sometimes, despite their best efforts, biologists may still fail (or may not have enough time) to obtain much needed natural history information during a critical period. To overcome such a challenge, biologists have, at times, used natural history information of a substitute species (which is different from surrogate species, Section 13.3.5) to fill data gaps for a rare species (Caro et al., 2005). An example of this application comes from the USA where researchers used behavioural observations of a common butterfly to predict dispersal of another closely related butterfly that was too rare to properly study (Hudgens et al., 2012). It is important to note that using information from substitute species does have serious limits (Henry et al., 2019). For example, considering that different populations of a single species may have very different environmental needs and adaptations, using data from a different species may be even less useful. Care must therefore be taken when using data from substitute species with proper acknowledgement of the assumptions and uncertainty this approach adds to one’s research.
11.2 Saving Species Through Translocations
Because the probability of extinction increases rapidly for small populations (Section 8.7), conservation biologists often invest considerable energy into increasing the size of small and declining populations. Often, these projects involve improving the extent and quality of suitable habitat (Chapter 10) or mitigating threats such as overharvesting (Chapter 12). When appropriate, conservation biologists may sometimes resort to translocations—moving individuals from sites where they are threatened (e.g. unprotected lands or a paper park) or overabundant (e.g. a well-managed protected area or ex situ conservation facility) to sites where they can offer a larger contribution to conservation efforts.
Understanding a species’ ecological needs is critically important for translocations, because it influences the choice of release site and type of preparations needed.
Conservation biologists generally recognise four basic translocation approaches:
- Restocking (also called augmentation) occurs when wildlife managers increase the size and genetic diversity of existing populations, by releasing individuals that have been raised in captivity or that have been obtained from other wild populations.
- Reintroduction occurs when wildlife managers release individuals into areas where they occurred in the past but not at present. The areas must be ecologically suitable and the factors that caused the extirpation must have been reduced or eliminated for a reintroduction to be successful.
- Introduction involves creating new populations by moving individuals to suitable areas outside that species’ historical range. Introductions are usually considered when reintroductions are impossible because the species’ historical range has been degraded too severely or because persistent threats will lead to reintroduction failure.
- Assisted colonisation (also called assisted migration) is a special class of introduction where biologists “assist” species with poor dispersal capabilities to adapt their ranges in response to environmental changes. It is anticipated that this strategy will become an important conservation tool in preventing extinctions where climate change outpaces the speed of natural migration.
11.2.1 Important considerations for translocations
Section 11.1 broadly discussed the importance of understanding the ecological and other natural history needs when protecting threatened species. Understanding a species’ ecological needs is equally, if not more, important for translocations, because it influences the choice of release site and type of preparations needed (Figure 11.4). Complementing the 10 factors mentioned in Section 11.1, the next section briefly introduces some of the most important considerations during translocations.
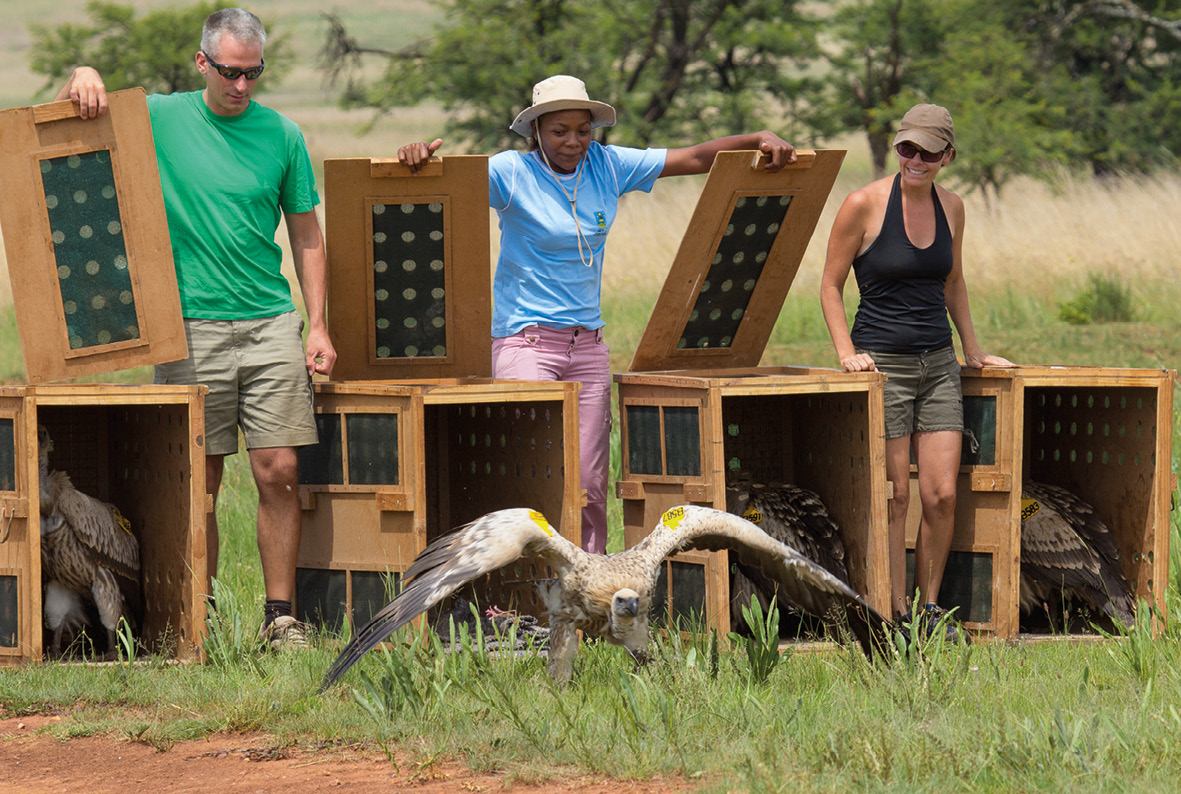
Figure 11.4 A team of wildlife rehabilitators release a group of Cape vultures (Gyps coprotheres, EN) near a vulture restaurant in South Africa. Releasing the vultures near a supplemental food source greatly enhances their chances for survival after release. The vultures have wing tags to enable monitoring of each individual after release. Photograph by VulPro, CC BY 4.0.
Determining need and feasibility
Perhaps the most important factor to consider before starting a translocation is to determine whether it is necessary. Translocations carry risks, not only for the target population to be moved, but also the individuals left behind and for the recipient ecosystem. These risks expose translocation projects to a high risk of failure, particularly if preparations are inadequate and essential resources (e.g. funding, trained staff) are in short supply. Translocations also demand considerable resources—resources that can at times be better spent mitigating the threats the target population face. While these considerations may seem obvious, a recent review found that most translocations projects are initiated without proper cost-benefit analyses (Pérez et al., 2012). To improve translocation practices, conservationists seriously considering a translocation project are encouraged to review the 10 criteria outlined in Pérez et al. (2012), some of which also overlap with the considerations mentioned below.
Support from local stakeholders
It is also important to consider, at an early stage, how the public will view the translocation project. Some people may feel the resources used in a translocation are better invested elsewhere; others dislike translocations because they view it as a threat to their livelihood—this is especially true when carnivores are involved (Gusset et al., 2008a). Because of these and other potential conflicts and emotions, it is crucial that translocation projects (like any conservation activity) obtain the support from local stakeholders at an early stage. It is helpful to be transparent from the outset and to explain the project’s goals, as well as the benefits the local community may gain (e.g. attract more tourists, restore a degraded ecosystem service). Good public outreach also provides opportunities to address the public’s concerns and misconceptions about the project and about biodiversity conservation in general.
Identifying suitable habitat
It goes without saying that the probability for success is greatly improved when the translocated individuals are released in good quality habitat. This is particularly true for species with poor dispersal capabilities, such as plants that reproduce through vegetative propagation: the plants could die in an environment that is too sunny, shady, wet, or dry. While this point may seem obvious, many translocations fail because individuals are released in inferior habitats (Armstrong and Seddon, 2007). One of the reasons for this potential habitat mismatch is because wildlife may perceive the environment differently than humans, so a site that may look good to the human eye may lack one or more overlooked limiting resource. Refugee species—species forced to live in suboptimal habitat due to threats present in their preferred habitat (e.g. Ali et al., 2017)—also present a challenge to biologists who may unwittingly view inferior habitat as optimal and base conservation decisions on essentially bad information. The same challenge presents itself at ecological traps—unsuitable environments that an organism mistakenly perceives as optimal habitat (e.g. Sherley et al., 2017). These are some of the most important reasons why biologists need to be cautions when using species distribution models (SDM) when identifying areas suitable for translocations. To mitigate costly translocation failures, it is advisable that releases start small, and have multiple phases, to assess how released individuals respond to their new environment. Conducting experimental and adaptive releases can also reduce uncertainty by evaluating different release scenarios (Menges et al., 2016).
It is also important to ensure that any habitat identified as suitable is free from threats such as pollution and invasive species that may lead to declining health or even death for released individuals. A project in the Cape Floristic Region in South Africa provides a good example of how alert conservation biologists mitigated a threat that could have caused a translocation failure. The Clanwilliam sandfish (Labeo seeberi, EN) was once widespread in the region’s Olifants-Doring River system. However, recent surveys indicated that the species had gone extinct in the Olifants River. Although biologists did find some juvenile fish in the Doring River and some of its tributaries, they also noticed that invasive fish predated on most of those juveniles before they reached adulthood (Jordaan et al., 2017). These ill-fated individuals were thus dispersing from the last remaining reproductive subpopulation persisting in the headwaters of one single Doring tributary to other parts of the river, which acted as a population sink. To prevent the species’ extinction, biologists initiated a habitat restoration plan involving restoring natural stream flow regimens and eradicating predatory invasive fish in the headwaters of a second Doring tributary. They then installed barriers that prevented invasive fish from reaching the restored area before translocating 338 juvenile fish (Figure 11.5) there. With this habitat restoration plan, the biologists hope to establish a second viable population, and to improve the juveniles’ chances of surviving to adulthood before they disperse back to areas where the invasive fishes occur (Jordaan et al., 2017).
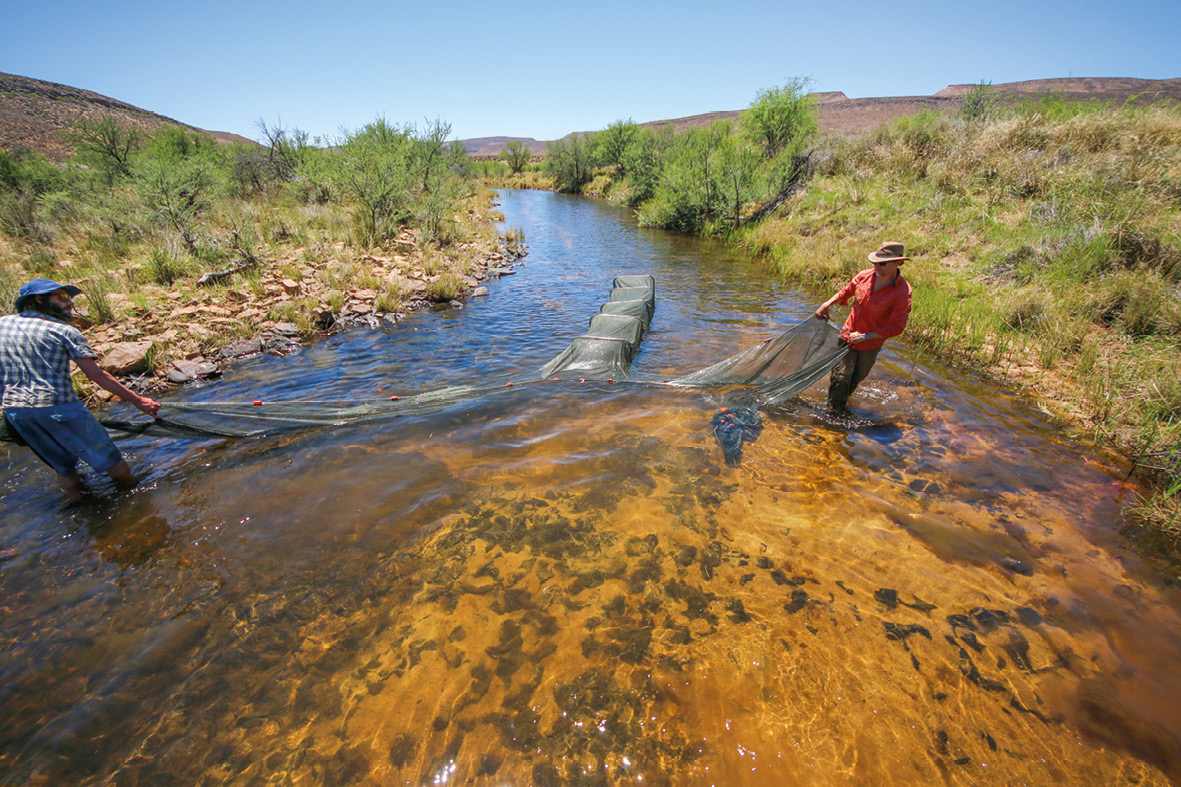
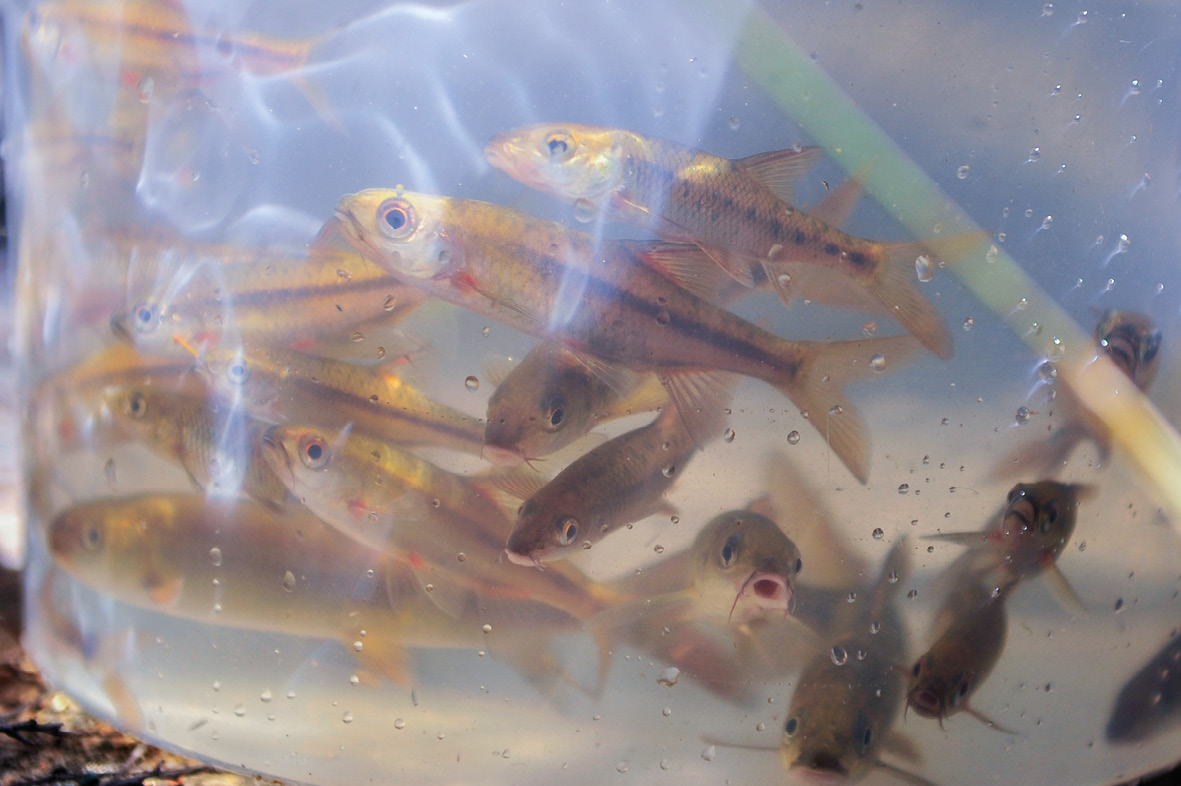
Figure 11.5 (Top) Conservation biologists collecting threatened Clanwilliam sandfish for a reintroduction project in the Cape Floristic Region, South Africa. Photograph by John Lucas/explore4knowledge®, CC BY 4.0. (Bottom) A close-up view of Clanwilliam sandfish. Photograph by Gustav Klotz/explore4knowledge®, CC BY 4.0.
Considering genetics and behaviour
Translocation projects also need to consider the genetic makeup, social organisation, and behaviour of a species that is being released. It is preferable to use individuals from the same genetic stock as individuals that already occur (or have occurred) in the release area to avoid outbreeding depression and to capture local adaptations (Sections 8.7.1). Such efforts simultaneously also contribute to conservation of genetic diversity, as opposed to the pollution thereof if individuals from different genetic stock are mixed.
Group-living species, particularly those vulnerable to Allee effects (Section 8.7.2), need to be released in sufficient numbers so they can maintain their natural social organisation and behaviour. For species that need to be released in groups, it is preferable to release socially integrated animals rather than individuals unfamiliar with each other (Gusset et al., 2008b). Releasing groups of animals does have its own set of challenges. For example, social groups abruptly released from captivity may disperse explosively, possibly leading to project failure. This happened with African buffalo (Syncerus caffer, NT) herds translocated to South Africa’s Addo Elephant National Park which fragmented into smaller groups after release, making them more vulnerable to lion (Panthera leo, VU) predation (Tambling et al., 2013). Fortunately, in this case, the buffaloes underwent several behavioural modifications over time, which eventually allowed their numbers to stabilise (Box 11.2). This contrasts with failed rock hyrax (Procavia capensis, LC) reintroductions in South Africa, where group disintegration post release exposed the animals to unsustainable predation levels (Wimberger et al., 2009).
Box 11.2 Large Predator Reintroductions: A Balancing Act
Department of Zoology and Entomology, University of Fort Hare,
Alice, South Africa.
ctambling@ufh.ac.za
Large predator numbers are declining, and African carnivores are no exception (Ripple et al. 2014). How to conserve African carnivores are a hotly debated topic now, with “fortress” type conservation areas considered the most viable option by many (Packer et al., 2013). In South Africa, this conservation model is the norm, and many small protected areas are now translocating large carnivores for ecotourism. However, these large carnivore translocations have repercussions for resident prey species. Understanding the ecological and biodiversity consequences of these translocations is thus important for the management of these small protected areas (Tambling et al., 2014).
In 2003, lions and spotted hyenas (Crocuta crocuta, LC) were reintroduced into the Addo Elephant National Park Main Camp Section after being absent from the area for over 100 years. Post-release monitoring of the six reintroduced lions indicated that at least 50% of their diet in the first two years following reintroduction was African buffalo. This was especially concerning to South African National Parks as this resident buffalo population contributes substantially to game auction sales each year, with the money raised being used to expand the national park system in South Africa (SANParks, 2009).
Following high predation rates of buffalo by lion and a 2007 buffalo census suggesting low juvenile recruitment, the coexistence of lion and buffalo in Addo was questioned. These concerns lead to a detailed assessment of buffalo behaviour and demographics between 2008 and 2011 (Tambling et al., 2012), which showed that by 2008–2009, juvenile buffalo recruitment (Figure 11.B) had rebounded to levels reminiscent of those prior to the lion reintroduction. Direct observations of the buffalo population showed drastic behavioural alteration following the high initial predation rates by lions. These behavioural changes included: (1) increased breeding herd sizes, (2) a reduction in nocturnal movement, and (3) greater use of open habitats at night and early morning when lions are hunting. These behavioural adjustments enabled the active defence of the breeding herds, reducing successful predation by lions and ensuring an increase in buffalo recruitment. Although this study suggests that prey populations are capable of behavioural adjustments to reduce predation, this is not always the case, with some species unable to respond, leading to precipitous declines in prey populations such as eland (Tragelaphus oryx, LC) (Leaver, 2014).
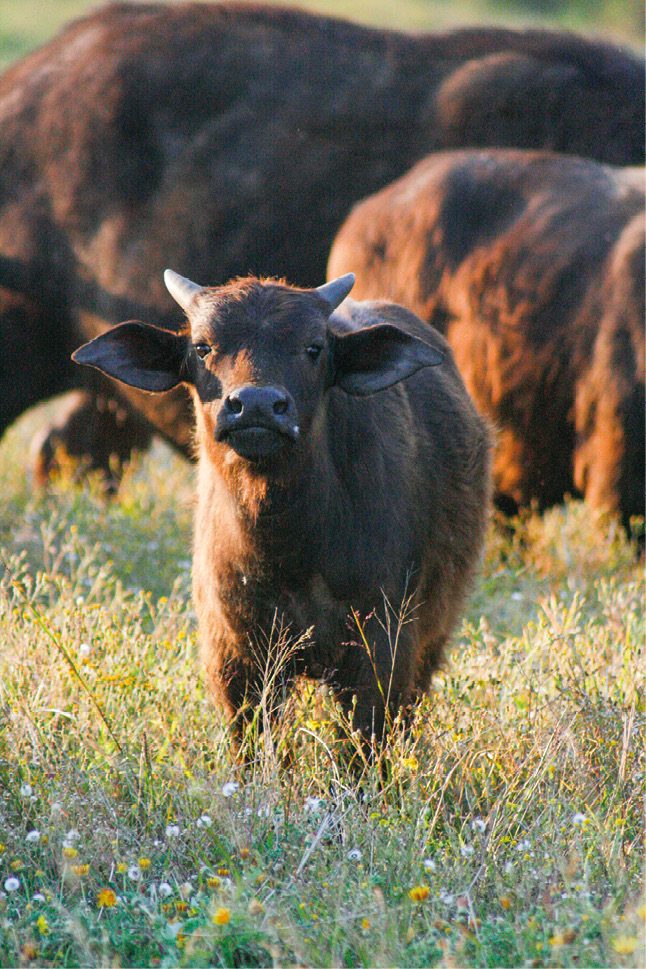
Figure 11.B After lions were reintroduced into the Addo Elephant National Park Main Camp Section, high levels of predation of buffalo (in particular, juveniles) prompted an investigation into the demographics and behaviour of the buffalo population. Results showed that buffalo were adjusting their behaviour to make greater use of open habitats, which subsequently led to improved juvenile buffalo survival. Photograph by C. Tambling, CC BY 4.0.
The ultimate aim of most translocation projects is to establish populations that are self-sustaining, free from inbreeding, and interactive participants of their communities and ecosystems.
Case studies of predator-prey interactions following large predator reintroductions highlight the management challenges faced by small reserves where ecotourism, biodiversity, and financial goals each need to be met. Due to the small size of these “fortress” reserves, a local overabundance of predators can have severe ecological effects on prey populations. However, in many reserves, the high demand for large predators for ecotourism often results in costly reactive, rather than scientifically sound proactive, management. There is, however, a growing body of research on the proactive management of large carnivores, where wildlife managers aim to replicate ecological processes (i.e. lion inter-birth intervals) to limit management interventions required to control large predator numbers (Ferreira and Hofmeyr, 2014). In small reserves, lion inter-birth intervals are shorter than in large ecosystems, and so lengthening the inter-birth intervals to that observed in large ecosystems can reduce lion population growth rate in these small reserves (Miller et al., 2015). Understanding predator-prey interactions is important regardless of the conservation model employed to protect these large charismatic species.
How many individuals to release
The ultimate aim of translocation projects is to establish ecologically relevant populations, meaning populations that are self-sustaining, free from inbreeding, and an interactive participant of its community and ecosystem. The probability of achieving this goal increases as more individuals are being released. Because translocation projects typically do not have an unlimited supply of individuals to release, wildlife managers often rely on quantitative models (Section 9.2) to estimate the minimum number of individuals that should be released and how many times releases should occur. For example, a population viability analysis (PVA) on western lowland gorillas (Gorilla gorilla gorilla, CR) reintroduced to Gabon and the Republic of the Congo showed that the probability of persistence of an apparently established population could be increased significantly if more individuals were released (King et al., 2014).
The ability to establish new populations through translocations does not reduce the need to protect threatened species still in their natural habitats.
While releasing more individuals certainly improves the likelihood of establishing a self-sustaining population, it is also important to determine how many individuals the target community can sustain. In other words, the release area should contain enough suitable habitat to support the territories of all the released individuals. To determine how many individuals can be sustained, wildlife managers may calculate the release area’s carrying capacity—an estimate of the maximum number of individuals an ecosystem can support. The carrying capacity concept has its roots in the livestock trade, where farmers wanted to maximise the number of animals on their land without risking overgrazing. While the concept has gained popularity in conservation biology in recent decades, calculating the carrying capacity for wildlife is very complex because of all the multi-faceted interactions that characterise healthy ecosystems. For example, the carrying capacity for a wild population can depend on factors such as food, water, shelter, soil nutrients, and sunlight availability, as well as more species-specific natural history factors such as habitat quality, home range, sex ratios (Tambling et al., 2014), and interactions with other species (Lindsey et al., 2011).
Over the past few decades, through trial and error, adaptive management (Section 10.2.3), and the collection of vast amounts of demographic data, scientists have made significant progress in calculating carrying capacity for wildlife populations. Perhaps the most progress has been made in calculating carrying capacities for large ungulates, by monitoring vegetation biomass, which in turn is affected by soil nutrients and rainfall (Fritz and Duncan, 1994). Much progress has also been made in calculating carrying capacities of predators by monitoring prey densities (Hayward et al., 2007a). For most populations, however, carrying capacity isn’t explicitly calculated, but implicitly estimated based on intuition. Refining existing carrying capacity models and developing new methods for other taxa remain an active area of research that will hopefully reduce conservation biologists’ over-reliance on intuition in future years. But even in the absence of carrying capacity calculations, wildlife managers can track a population’s health and overall fitness. When the health of a particularly successful population or its environment starts declining, a root cause may be that too many individuals have been released, or the population is being sustained above carrying capacity.
Preparing individuals for release
Translocation projects using individuals obtained from the wild are generally much more successful than those using captive-bred individuals, given that wild individuals are already adapted to a life where they must fend for themselves. Nevertheless, some projects may have to use captive-bred individuals, particularly when the target species is extinct in the wild, or when individuals were brought to an ex situ conservation facility because it is easier to breed them under human care in controlled conditions. In such cases, a great amount of effort may be required to prepare the captive-bred individuals for releases.
A great amount of effort may be required to prepare captive-bred individuals for translocation because they may have lost adaptations required for survival and reproduction in the wild.
A major drawback when using captive-bred individuals is that they may have lost the important adaptations required for survival and successful reproduction in the wild. Pre-release training, which varies according to the species, can sometimes overcome this drawback. For predators, it may involve providing low risk prey, such as chickens and domestic rabbits in holding facilities until their hunting skills are better developed (Houser et al., 2011). For plants propagated indoors, it may involve hardening them off by placing them outside for increasingly longer periods to gradually introduce them to sun, wind, and temperature changes during the day. To help young birds disassociate humans from food, human trainers sometimes use puppets or wear costumes (Figure 11.6) during feeding time to mimic the appearance and behaviour of wild individuals (Valutis and Marzluff, 1999). Another method, which may promote behavioural enrichment, involves cross-fostering, in which unrelated parents helps raise the offspring of a threatened species. In carnivore conservation, this technique has shown much promise to augment litter size and encourage gene flow using orphaned African wild dog (Lycaon pictus, EN) pups (McNutt et al., 2008). Interspecific cross-fostering has also been used in bird conservation, where biologists use common species to incubate eggs abandoned by threatened species (e.g. Powell and Cuthbert, 1993). However, cross-fostering using different species may lead to a new set of problems, like behavioural changes and hybridisation, if the young subsequently associate with the wrong species. A great amount of care and research are thus needed before such strategies are attempted.
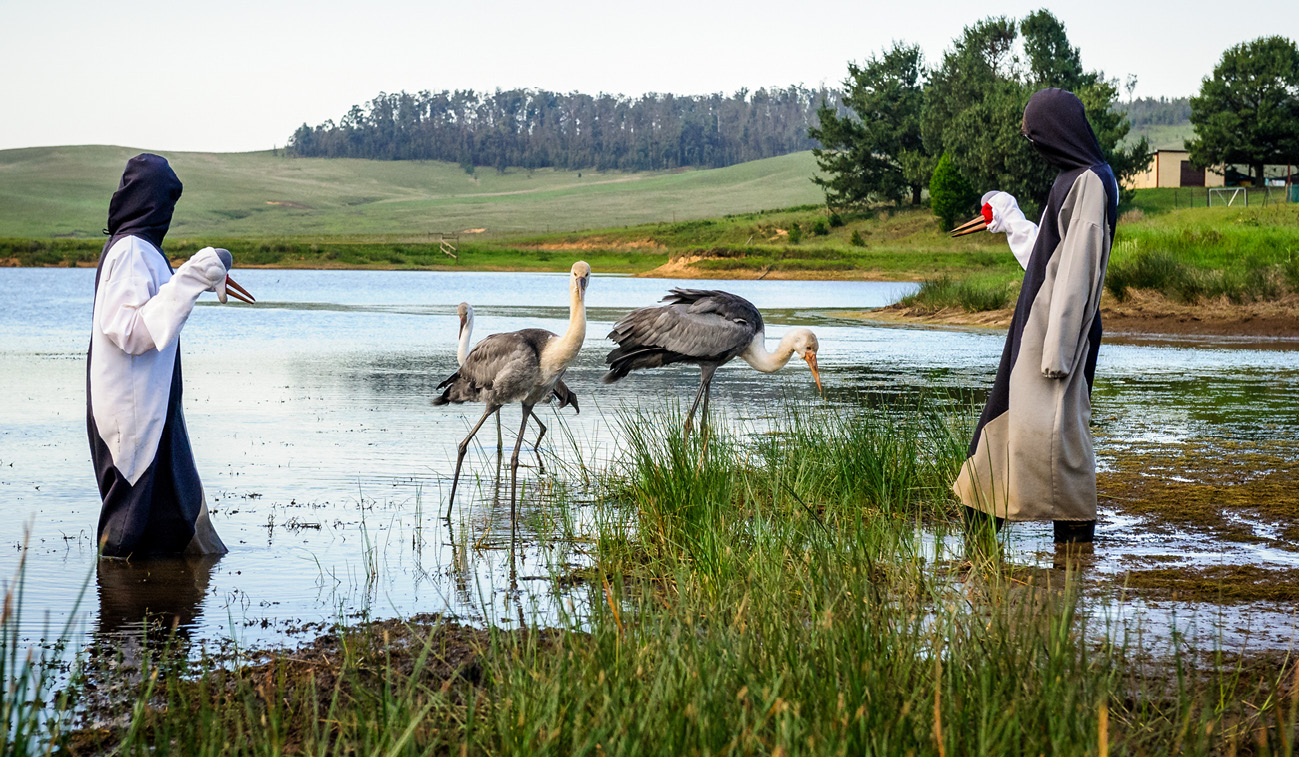
Figure 11.6 Wattled cranes (Grus carunculate, VU) sometimes lay two eggs, but always abandon the second egg. Conservation biologists in South Africa are collecting the discarded eggs, which are then hatched in a captive breeding programme. To avoid the captive chicks associating humans with food and safety after release, handlers use special crane costumes when interacting with the birds. Photograph by Daniel Dolpire, CC BY 4.0.
Whether using captive-bred or wild individuals for translocations, individuals may have to be fed, sheltered, trained, or otherwise cared for after release to give them time to become more familiar with their new surroundings. This approach, known as soft release, involves keeping the released individuals in pre-release holding facilities for a period; it may also include some form of assistance after release to increase opportunities for success. Soft releases also provide an opportunity to introduce captive-bred organisms to wild individuals of the same species that can act as “instructors” for survival in the new environment, or for unfamiliar individuals to bond into cohesive units (Gusset et al., 2006).
The alternative to soft release is a hard release—an abrupt release of individuals from captivity without assistance such as food supplementation. While hard releases are popular (because they are relatively easy to perform), it is a risky strategy that faces a high risk to failure (Brown et al., 2007; Wimberger et al., 2009). Hard releases can however be appropriate under the right conditions (Hayward et al., 2007b). For example, hard releases are often use in head-starting programmes (Figure 11.7) for reptiles and amphibians (Scheele et al., 2014), where conservation biologists collect wild individuals and raise them past their most vulnerable life stages before releasing them again where they were collected.
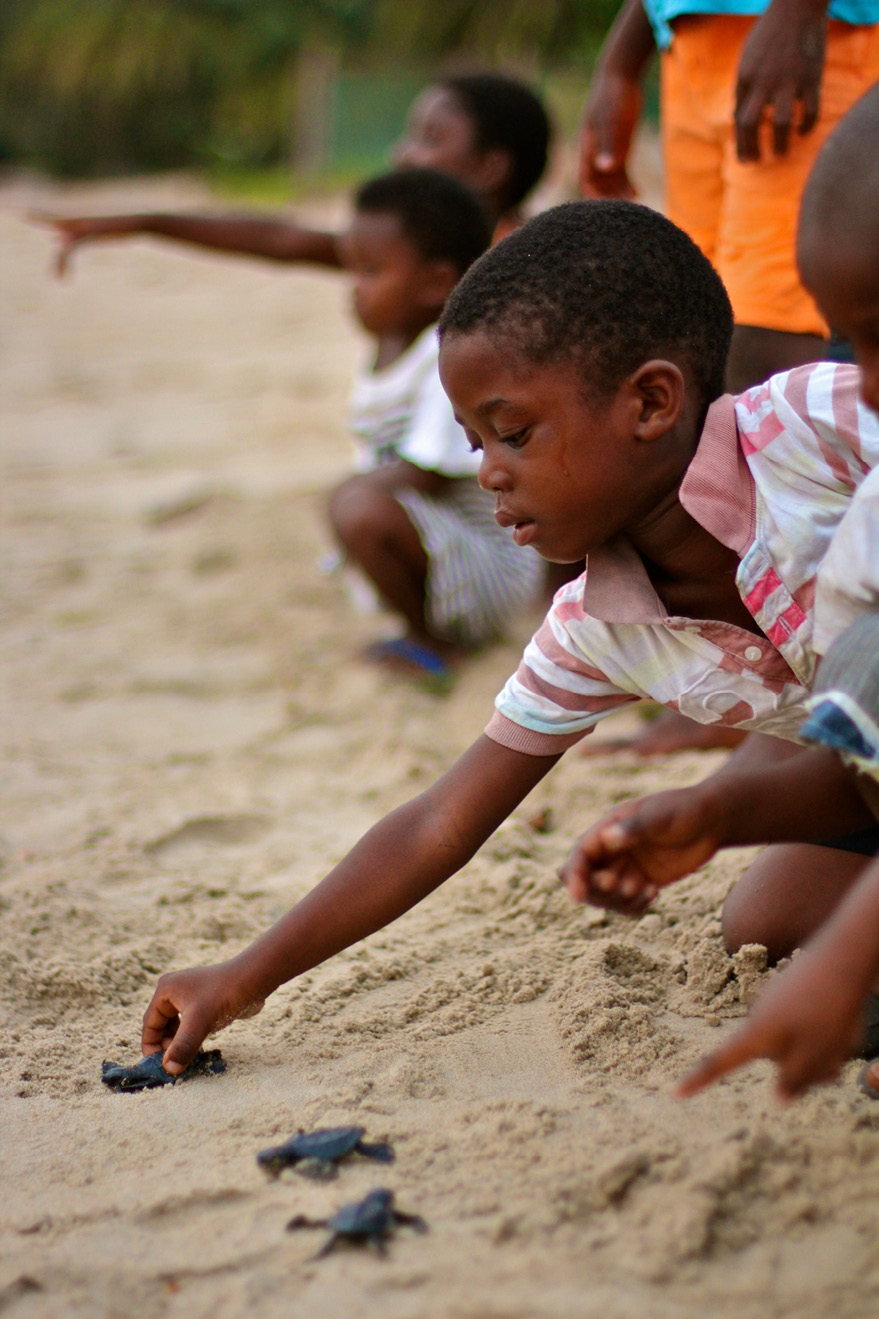
Figure 11.7 Local children releasing leatherback sea turtles (Dermochelys coriacea, VU) as part of an environmental education project in Equatorial Guinea. Known as head-starting, conservationists would sometimes collect sea turtle eggs in the wild, hatched in captivity, and raise the offspring past their most vulnerable stage before releasing them back where they were found. Photograph by Katharine Clukey/TOMAGE-INDEFOR, CC BY 4.0.
Post-release monitoring
A translocation project does not end after the last individual was released. Rather, ongoing monitoring should be implemented to determine whether a translocation was successful, what degree of success was achieved, whether adaptive management is needed, whether additional releases should be conducted, or whether the project should be aborted. A well-designed monitoring plan can also highlight the consequences of translocation on the broader ecosystem, such as the impact that predators introduced to a new area may have on prey populations (Box 11.2) and competing species (Groom et al., 2017). Because some responses in translocated populations can be rather subtle and take many years to show or subside, post-release monitoring should ideally be a long-term endeavour. For example, by monitoring seemingly successful elephant reintroductions across five protected areas in South Africa, researchers found that stress hormones in released animals continued to decline 24 years post release (Jachowski et al., 2013). Long-term monitoring will also help wildlife managers better understand the ultimate fate of the released individuals. Many apparently successful translocations fail because the released individuals die after several years without ever reproducing. Highlighting the importance of post-release monitoring, one study from South Africa found that 70% of captive-bred oribi (Oribia oribi, LC) died within two months of release, mostly due to predation (Grey-Ross et al., 2009). Another study found that reintroduced cheetahs were all killed within a year of release (Houser et al., 2011). These were expensive lessons, but post-release monitoring ensured that the reason for failures are known and can be addressed ahead of future releases.
Helping other translocation projects
Strategies used in successful translocation projects were nearly always informed by releases conducted by other wildlife managers who circulated their experiences to the wider conservation community. It is important to pay this effort forward; new translocation projects should make every effort to track and publish their results to inform others. While it is always easier to present the results of successful projects, publishing the lessons from failed projects is also important (Wimberger et al., 2010; Godefroid et al., 2011). Equally important is the publication of project costs, to enable wildlife managers to better determine under which conditions translocations represent a cost-effective conservation strategy. For example, a large African wild dog reintroduction programme in South Africa achieved their initial goal of establishing nine self-sustaining packs much more quickly than expected—five years rather than 10—yet reintroducing all these populations cost 20 times more than if the funds were used to enhance protection of existing packs within protected areas (Lindsey et al., 2005). With more information available, future conservationists would hopefully be able to have better guidelines to maximise cost-effectiveness and the likelihood of project success.
11.3 Managing and Facilitating Movement Dynamics
Some ecosystems are transient in nature—their character is temporary and will change because of disturbance and succession. Consequently, species that occupy those transient habitats are bound to be naturally extirpated at one time or another. Consider, for example, a small population of wildflowers occurring in a river’s floodplain; at some stage, there is going to be a flood that will wash away those flowers. But the flooding also disperses seeds downstream, allowing for new wildflower populations to establish in suitable habitat elsewhere. These shifting populations linked by movements between them are better characterised as a metapopulation (a “population of populations”) (Figure 11.8) consisting of several subpopulations. For some metapopulations, every subpopulation is transient: their distribution changes dramatically with each generation. Other metapopulations involve relatively permanent subpopulations with only a few individuals dispersing each generation. Some metapopulations consist of one or more source populations whose sizes are stable or increasing, and several sink populations whose sizes fluctuate depending on environmental conditions. Some sink subpopulations may undergo such dramatic fluctuations that they would be extirpated in unfavourable years were it not for population rescue by immigrants from source populations.
A metapopulation (a “population of populations”) consists of several subpopulations linked by movements of individuals between them.
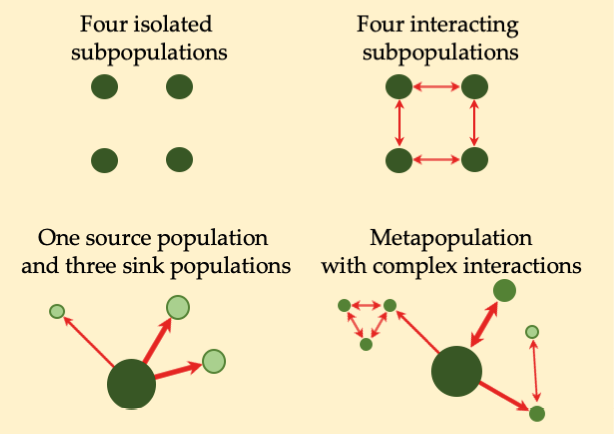
Figure 11.8 A range of metapopulation patterns is possible in nature. In this illustration, population size is represented by the size of the circle, while movement direction and intensity are indicated by the direction and thickness of the arrows. After White, 1996, CC BY 4.0.
Habitat fragmentation threatens metapopulation dynamics by reducing opportunities for dispersal across the landscape (Chapter 5). When there is too little movement of individuals between habitat fragments, the dwindling subpopulations within those fragments are at risk of extirpation or even extinction (Section 8.7). In contrast, well-connected subpopulations maintain themselves by colonising empty niches, exchanging genetic material, and adapting to changing environments. Dispersal also maintains critical ecosystem processes, such as pollination and seed dispersal (Section 4.2.5). Consequently, conservation biologists have invested significant resources in recent years to maintain and restore wildlife movements within fragmented ecosystems.
11.3.1 Connectivity in terrestrial ecosystems
Maintaining and restoring ecosystem connectivity—the ability of ecosystems to facilitate the dispersal of individuals between different areas—involves maintaining and restoring wildlife movements that are (at risk of being) impeded by human activities. The most popular method to maintain (or restore) connectivity in a fragmented landscape is to maintain (or restore) habitat linkages, also called wildlife corridors, habitat corridors, dispersal corridors, or movement corridors. All these terms refer to continuous tracts of suitable habitat with little to no dispersal barriers that connect otherwise isolated habitat patches and populations.
Maintaining and restoring ecosystem connectivity is an important strategy for conserving wildlife whose movements are impeded by human activities.
Some of the most prominent efforts to restore habitat linkages involve habitat restoration. For example, plans are currently underway to use forest regeneration to reconnect nine forest fragments in Tanzania’s East Usambara Mountain; if successful, this project would establish the largest contiguous forest block (over 3,000 km2) in the Eastern Arc Mountain Biodiversity Hotspot (Newmark, 2008). The positive impact of this project is expected to be immense. It has been estimated that the restoration of just 80 km2 of forest would stave off the first fragmentation-induced extinctions by over 2,000 years, compared to an estimated seven years until the first extinction if these forest fragments were to remain unconnected (Newmark et al., 2017).
Connectivity is important in every ecosystem on Earth. However, given the linear characteristic of riparian zones along rivers and stream—and hence a larger proportional impact of edge effects (Section 5.1.2)—we might consider connectivity in these spatially restricted systems to be particularly important (Figure 11.9). Protecting and restoring riparian zones as habitat linkages resonates with a variety of people because these areas provide a range of important ecosystem services, including flood control and water purification (Section 4.2.4). Conservationists can tap into this energy by lobbying for laws that prohibit activities such as logging, housing, and industrial developments within a certain distance from a river or stream. By protecting ecosystem services associated with riparian zones, these laws simultaneously also maintain wildlife refuges (Monadjem and Reside, 2008), source populations (Vosse et al., 2008), and habitat linkages (Bentrup et al., 2012; McLennan and Plumptre, 2012). In contrast, inadequate protection of riparian ecosystems not only compromises connectivity, but also negatively affect species not overtly dependent on these buffer areas. For example, research from Southeast Asia has shown that losing riparian ecosystems in an otherwise palm oil dominated landscape reduced stream quality, which in turn reduced local fish diversity by up to 36% (Giam et al., 2015). In contrast, protecting riparian zones were found to increase palm oil yields (Horton et al., 2018). With so many riparian areas currently being degraded and destroyed, there is an urgent need for stronger riparian protection laws (Chapter 12), and for more effective enforcement of those laws.
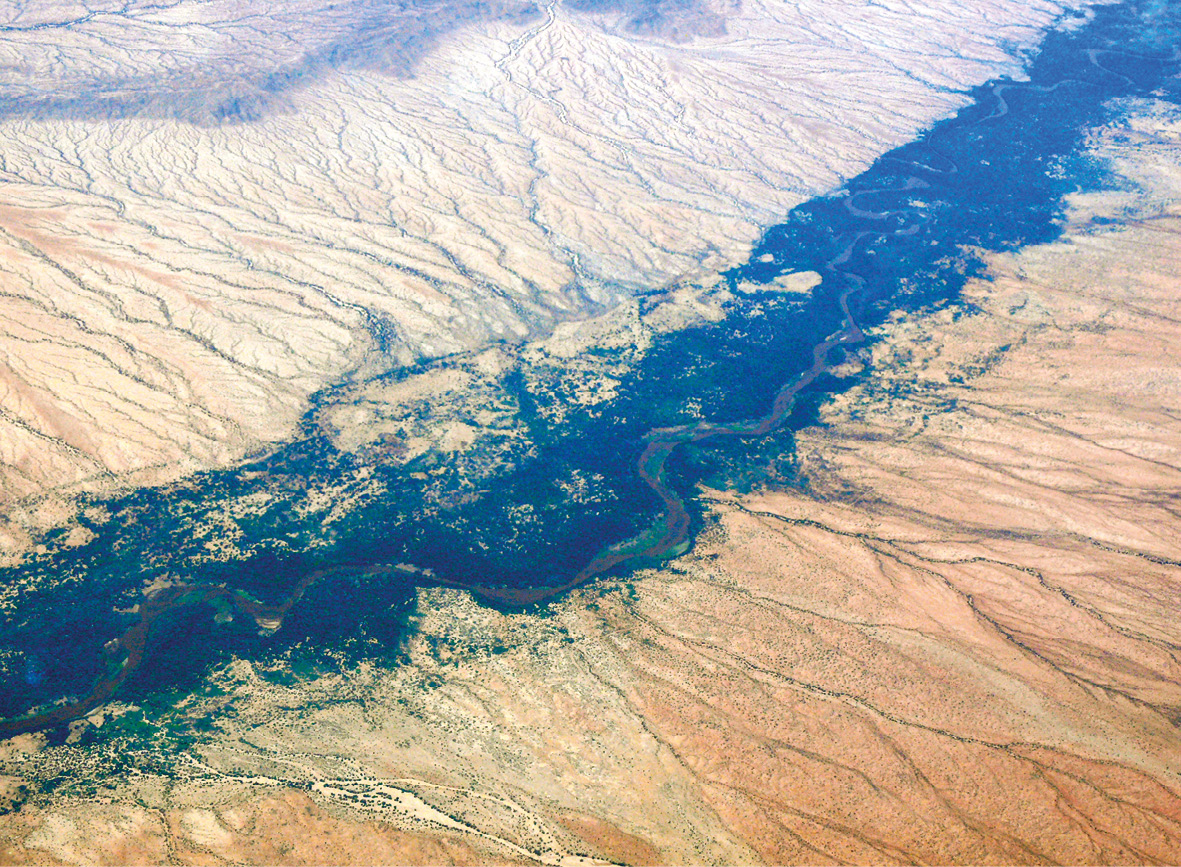
Figure 11.9 Protecting riparian zones such as this one along the Turkwel River in northern Kenya is an effective strategy for maintaining connectivity and securing a range of ecosystem services. Photograph by Bernard Dupont, https://www.flickr.com/photos/berniedup/17966234205, CC BY-SA 2.0.
Restoring connectivity may also involve removing or otherwise mitigating human constructs that block wildlife dispersal. This is a major aim of TFCAs, which aim to restore dispersal between protected areas (Jones et al., 2012) by removing fences and other human constructs while still maintaining sustainable land tenures (Andersson et al., 2013). These efforts, accomplished through partnerships with local communities, are re-establishing historical mass migration routes, which in turn will hopefully also boost those areas’ ecotourism potential (Box 11.3). Efforts to revive extinct mass migrations also seem to be paying off! For example, in Botswana, the removal of veterinary fences—meant to prevent spread of diseases from wildlife to livestock, but also cutting off the world’s second largest wildebeest migration—have seen several hundred plains zebras (Equus quagga, NT) returning to old migration routes within four years (Bartlam-Brooks et al., 2011).
Box 11.3 Transfrontier Conservation Areas: Managing Biodiversity Across International Boundaries
Kavango-Zambezi Transfrontier Conservation Area Programme,
Kasane, Botswana.
http://www.kavangozambezi.org
TFCAs are components of a larger ecosystems that straddles the border between two or more countries, encompassing one or more protected areas as well as multiple-resource areas used by communities and private landholders. They are also managed for sustainable use of natural resources (Singh, 1998). The concept recognises that borders are political rather than ecological (Dallimer and Strange, 2015), and aims to ensure that key ecological processes continue to function where political borders have divided ecosystems, river basins, or wildlife corridors (Cumming, 1999).
TFCAs are widely being established in Africa. One of these is the 520,000 km2 Kavango-Zambezi TFCA (KAZA)—a conservation and development initiative of Angola, Botswana, Namibia, Zambia, and Zimbabwe.
The benefits of the KAZA include:
- Re-establishment of the seasonal wildlife migration routes and connectivity among the many protected areas (national parks, community conservancies, and wildlife and forest reserves) within the region (Figure 11.C). The primary wildlife focus is the savannah elephant (Loxodonta africana), whose population of about 250,000 is predominantly concentrated in Chobe National Park (Botswana), Hwange National Park (Zimbabwe), and Bwabwata National Park (Namibia). Elephants need unimpeded movement to protected areas where population densities are much lower, such as Luengue-Luiana and Mavinga National Parks (Angola), and Sioma Ngwezi and Kafue National Parks (Zambia). This movement would reduce pressure on the ecosystems that are currently overpopulated and enable elephants and other species to better coexist—especially grazing herbivores that depend on the same habitats as the elephant.
- Expanding the wildlife-based economy, primarily ecotourism, into agricultural marginal areas (with predominantly Kalahari sand soils), through community-private partnerships. Through these partnerships, local communities would benefit from employment and business opportunities in ecotourism activities.
- Opportunities for local communities to participate in decision-making, and influencing policies and legislation related to natural management such as coordination of the fishing closed season between Namibia and Zambia during the fish breeding season (December–March) in the Zambezi River.
- Formation of alliances among different stakeholders (governments, private sector, NGOs, and local communities) to maximise skills and resources in promoting sustainable land use, conserving biodiversity and alleviating poverty.
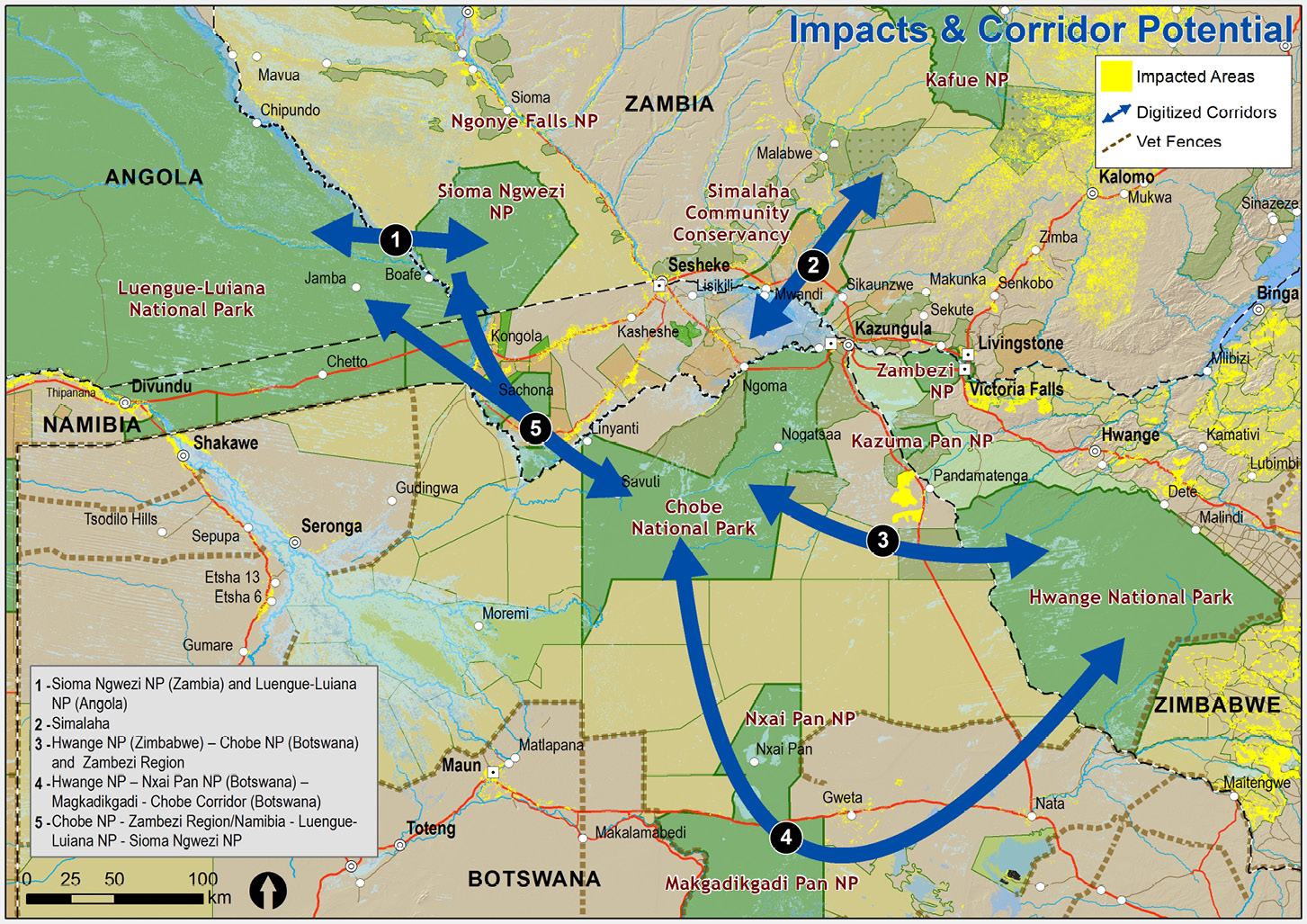
Figure 11.C Location of priority wildlife dispersal corridors between the various national parks of the KAZA TFCA. Map by Peace Parks Foundation, CC BY 4.0.
Despite these benefits, there are obstacles to progress in attaining the benefits of the KAZA. Notable among these are social and political factors, such as increasing human population density, increasing cultivation of land, and expanding human settlements in wildlife corridors. Many of these factors trigger human-wildlife conflicts and poaching both for local consumption of bushmeat and for the illegal sale of elephant ivory. To mitigate these threats, the following strategies are being implemented:
- A Master Integrated Development Plan for the KAZA has been developed, which provides initial zoning. Its key feature is spatially allocating land into various uses (human settlement, agriculture, and protected wildlife areas, including wildlife dispersal corridors). The Master Integrated Development Plan also assists in creating awareness about the value of the wildlife corridors, which traverse communal areas.
- Promotion of conservation agriculture as a tool for improving land stewardship, intensification of agriculture, and improving crop yields per unit area of land, and therefore decreasing the likelihood of cutting down forested areas in and around wildlife corridors to plant new agricultural fields. Currently, within the KAZA, conservation agriculture is being piloted in Angola, Namibia, and Zambia. Conservation agriculture is crop production that strives to achieve acceptable profits together with high and sustained production levels while concurrently conserving the environment.
- Promotion of community-private partnerships in ecotourism development. Over the past four years, Ngoma safari lodge (Botswana), and Machenje sport fishing lodge (Zambia) have been developed specifically in support of securing wildlife corridors. They also provide incentives to the local communities for adopting wildlife conservation as a supplement to their land use practices. These lodges are in addition to the numerous existing tourist resorts in the KAZA.
- A law enforcement and anti-poaching strategy for the KAZA is being developed to coordinate transboundary law enforcement surveillance and fines to prevent poaching of protected wildlife. In addition, KAZA partner countries are integrating other security agencies, such as the military, police, immigration, and customs officials, to prevent the illegal export of wildlife products such as elephant ivory and bushmeat out of the KAZA.
- Reducing human-wildlife conflicts (Section 14.4) through improved land use planning, solar-powered electrified fencing encircling clusters of village fields and facilities and use of chilli-pepper-based olfactory repellents to deter elephants from entering crop fields.
The KAZA has made considerable progress to date in coordinating conservation efforts among the wildlife agencies and national parks across five countries in Southern Africa. The principal success has been measures to allow the continued migration of elephants along existing migration routes across international borders. The challenges ahead—from inadequate funding for wildlife patrolling and anti-poaching activities to increasing populations of rural people outside the protected areas and across migration routes—remain significant.
Section 5.1.1 discussed how inconsiderate fence placements threaten wildlife, while the paragraph above explained how removing fences can improve connectivity. Ironically, and illustrating the difficulties conservationists face when dealing with conflicting demands, strategically placed fences can sometimes also be used as a conservation tool. For example, researchers working on a fragmented lion population in Botswana found that the most effective way to improve this population’s viability was through strategic placement of fences to direct dispersal between protected areas (Cushman et al., 2016). Strategically placed predator-proof fences may at times also be required to avoid human-wildlife conflict (Packer et al., 2013, but see Creel et al., 2013), and to facilitate the recovery of threatened species, as is the case for Africa’s rarest antelope, the hirola (Beatragus hunter CR) (Ng’weno et al., 2017). The final word here is that management must remain responsive to both positive and negative impacts of tools, such as fences, rather than relegating them to bins, such as good or bad. (See also Dupuis-Desormeaux et al., [2018] for the use of fence-gaps and exclusionary fences to mitigate some negative fence impacts.)
Protecting and restoring stepping stone habitats can maintain connectivity in areas where it is impractical to establish or restore continuous habitat linkages.
At times, when it is impractical to establish or restore continuous habitat linkages, biologists may opt to protect and restore stepping stone habitats (Figure 11.10). As the name implies, stepping stone habitats are a special type of habitat linkage that facilitate dispersal along a patchwork of isolated habitat patches within a matrix of unsuitable or inhospitable habitat. Stepping stones thereby divide long dispersal events through a long stretch of inhospitable terrain up into shorter, and thus more manageable, sections. Stepping stone habitats are particularly important for migratory species that rest and refuel at stop-over sites between the end-points of their migratory route (Runge et al., 2015)—each stop-over site can be viewed as a stepping stone habitat. Prominent examples of stepping stone habitats that deserve protection include sacred forests which can act as stop-over sites for migratory forest birds; wetlands and estuaries (see Box 5.3), which can act as stop-over sites by migratory waterbirds; and small forest reserves, which can act as stepping stones between a network of other protected areas (Riggio and Caro, 2017).
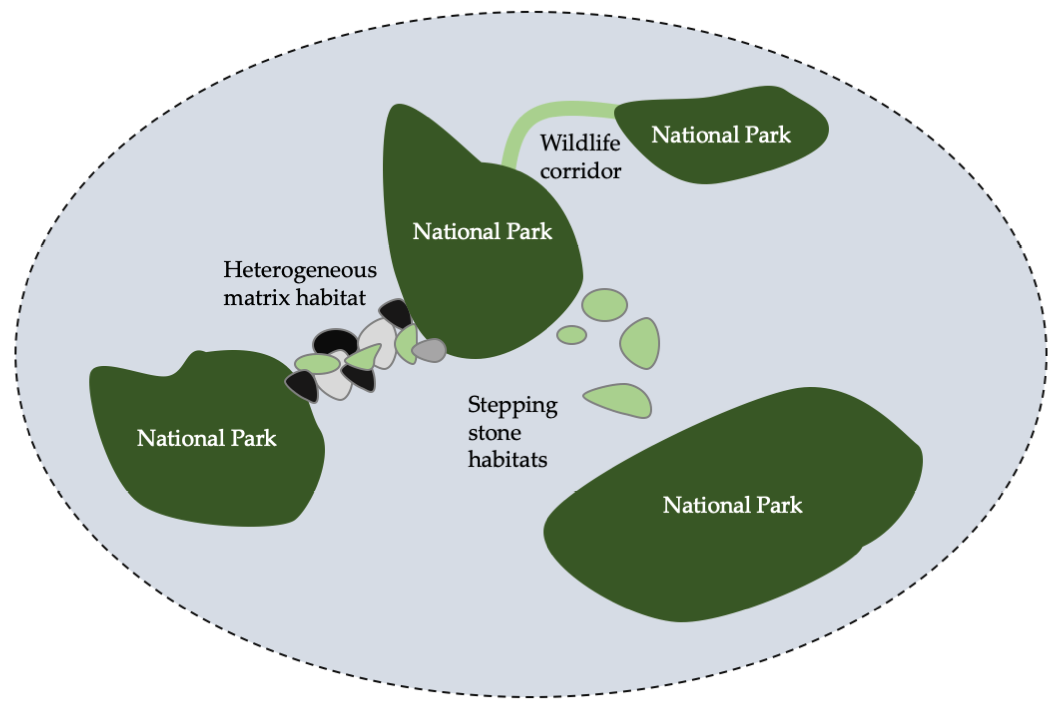
Figure 11.10 Methods to reconnect fragmented metapopulations (or maintain connectivity) can take many forms. The three main strategies are to maintain or restore wildlife corridors (e.g. to link two isolated forest patches), maintain or restore stepping stone habitats (e.g. a patchwork of wetlands or sacred forests), or facilitating movement through the matrix with sustainable land use tenures (e.g. removing fences). After Bennett, 2004, CC BY 4.0.
11.3.2 Connectivity in freshwater ecosystems
Dams have always played an important role in hydropower generation and securing a year-round supply of water for farms, industries, and cities. Unfortunately, recent evidence suggests that reservoirs may create more problems than they solve (Section 5.3.2). Of concern is their contribution to greenhouse gases (Deemer et al., 2016), as well as their role in blocking dispersal of aquatic organisms. To counter these negative impacts, governments across the world are decommissioning and removing dams and other types of artificial water impoundments. For instance, over the past 30 years more than 1,174 dams were removed in the USA; the 72 dams removed in 2016 alone restored more than 3,000 km of streams (Thomas-Blate, 2016). Similar efforts are also underway in Europe (http://www.ecrr.org), where river restoration efforts have been initiated at over 1,100 locations across 31 countries. Unfortunately, not only are efforts to restore freshwater connectivity lagging across Africa; in many cases, even more rivers are currently being dammed (Winemiller et al., 2016).
While dams play an important role in hydropower generation and securing a year-round supply of water, recent evidence suggests that they create many environmental problems, including blocking species dispersal.
11.3.3 Connectivity in marine ecosystems
Ecosystem connectivity is also important in marine ecosystems. Many marine organisms, including economically important species, breed and feed in different areas at different times of the year, and use established dispersal routes to move between those areas. It is thus important to protect these dispersal routes so we can maintain these marine ecosystems and ecosystem services.
Maintaining movement dynamics in marine seascapes involves protecting and restoring marine corridors, estuarine linkages, and coastal habitat linkages.
There are three main strategies to maintain and restore movement dynamics of marine seascapes. First, marine corridors—zones used by whales and other marine species to move between feeding and breeding grounds—should be protected. Marine biologists in several countries successfully reduced collisions between whales and ocean-faring vessels with minor adjustments to shipping lanes that previously crossed marine corridors (Silber et al., 2012). Second, estuarine linkages should be protected, and restored where needed. For example, biologists in South Africa restored the natural flow regime of the St Lucia Estuary, Africa’s largest estuarine lake, by removing dredge spoil in the estuary mouth (Nunes et al., 2018). Third, coastal habitat linkages—beaches and littoral shallows used by wildlife for dispersal, breeding, and feeding—need to be maintained. Studies from South Africa have highlighted how poor protection of connectivity pathways between coastal habitats can compromise these areas’ high levels of species richness and endemism (von der Heyden, 2009; Harris et al., 2014).
11.3.4 Mimicking connectivity
In the absence of habitat linkages, wildlife managers may be able to mimic dispersal dynamics by sporadically translocating a few individuals between subpopulations. Managing populations in this way may be a good alternative in cases where areas earmarked for translocations are too small to sustain a single viable population. Such is the case in South Africa, where conservation biologists occasionally move threatened predators within a small and fragmented protected areas network, where none of the areas are large enough to host a viable population on their own (see Box 8.3). Managing isolated and small populations so intensively nearly always requires sound underlying principles and extensive quantitative analyses (Chapter 9) for guidance.
11.3.5 Management considerations in connectivity conservation
While intuitively appealing, there are a few potential drawbacks to connectivity that conservation planners should consider when planning to establish new habitat linkages (reviewed in Haddad et al., 2014). Prominently, connecting historically isolated populations may lead to outbreeding depression, for example when populations with different local adaptations are connected. Habitat linkages may also act as bottlenecks that expose dispersing animals to greater risks of predation and enable pests and diseases to spread easier. Care must be taken to ensure that wildlife do indeed perceive the landscape “connected”; a habitat linkage that may look good to the human eye may in fact be perceived as inhospitable habitat to wildlife (Newmark, 2008). A recent study from the Americas has shown that the habitat quality of a single stepping stone habitat can determine whether a migration is successful or not (Gómez et al., 2017).
Although the benefits for reconnecting fragmented landscapes generally outweigh the drawbacks, it is important to carefully plan to avoid those drawbacks.
Although the benefits for connecting landscapes for conservation generally outweigh the drawbacks (Haddad et al., 2014), it is important to carefully plan to avoid those drawbacks. Genetic studies can be useful in both determining connectivity among populations (von der Heyden, 2009; Godley et al., 2010) and help researchers detecting potential deleterious factors, such as outbreeding depression (Figure 11.11, see also Frankham et al., 2011; Ralls et al., 2018). Modelling approaches that combine a target species’ movement limitations with radio tracking technologies (e.g. Godley et al., 2010) or remotely sensed environmental variables (e.g. Wegmann et al., 2014) could help to estimate whether a landscape is indeed connected. Much effort has also been invested in finding the optimal width of habitat corridors. For example, one study in lowland forests suggested that corridors that are 30–40 m wide might be adequate for migration of most species while corridors that are 200 m wide will be adequate for all species (Laurance and Laurance, 1999). This is useful guidance, but ecosystems vary, as do target species (Wilson et al., 2010; Pryke and Samways, 2012) and, thus, some corridors may need to be even wider.
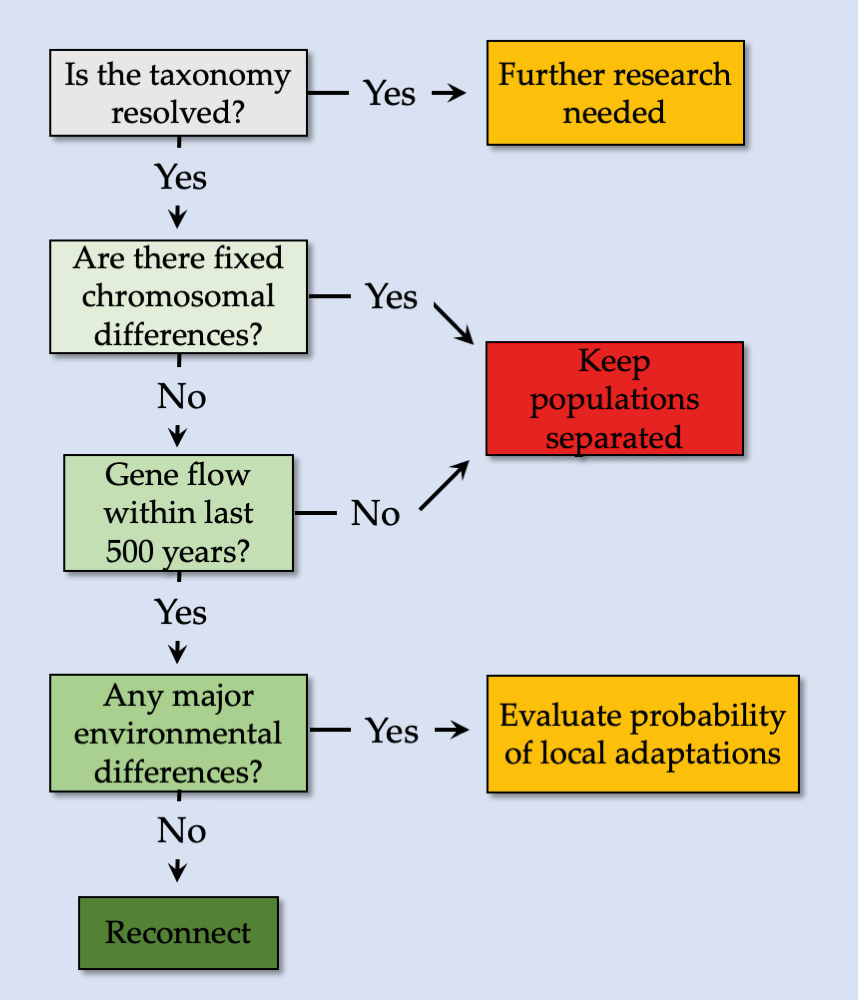
Figure 11.11 An example of a decision tree to avoid outbreeding depression, which can be used guide decisions for reconnecting fragmented landscapes. After Frankham et al., 2011, CC BY 4.0.
11.4 Managing Species Sensitive to Climate Change
Earth’s temperature is well on its way to exceed the 2°C increase cap set by global authorities in 2016 (Paris Agreement, Section 12.2.1). Many species that need to adapt to these changes are unable to do so, either because of their limited dispersal capabilities or because of human-induced habitat fragmentation (Section 6.3.5). Others that can disperse may risk decoupling of important symbiotic relationships, as the species involved may not disperse at the same speed, or the same distance (Section 6.3.2). While slowing habitat loss could slow the overall impacts of climate change (Section 10.4), preventing the extinction of many climate-sensitive species will require a range of pro-active conservation management strategies that allow species to adapt at their own pace as and when needed.
Preventing the extinction of climate-sensitive species will require a range of pro-active conservation strategies that allow those species to adapt at their own pace as and when needed.
One of the most important strategies for protecting climate-sensitive species is to identify and protect their likely future habitats. This task of predicting where suitable habitats may be found in future is generally accomplished by identifying and projecting a species’ climatic niche (or bioclimatic envelope) using species distribution models (SDM, Pearson and Dawson, 2003). Section 11.1.1 described how SDM use location data overlaid onto environmental variables to estimate a species’ environmental niche, and how this information can then be used to predict where else a species may occur in a landscape. A similar strategy is followed when predicting a species’ future climate-adapted range. Here, location data are overlaid onto present-day climate variables (e.g. average temperature and rainfall) to define the species’ climatic niche; these niche limits are then projected onto the landscape of interest using future climate scenarios (Section 6.2). Much effort has also been made in recent years to incorporate aspects, such as physiology (Kearney and Porter, 2009) and biological interactions (e.g. Araújo and Luoto, 2007), in predicting future ranges.
Once future ranges have been identified, the next task is to recognize and protect/restore critical dispersal pathways (Section 11.3). While a general strategy of increasing ecosystem-wide connectivity will certainty also benefit climate-sensitive species, conservationists could specifically target climate adaption, by maintaining and restoring climate corridors—dispersal pathways between the current and future ranges (Mawdsley et al., 2009). Several efforts (e.g. Williams et al., 2005; Phillips et al., 2008; Ayebare et al., 2013) are currently underway to establish and protect species-specific and community-specific climate corridors, as predicted using advanced distribution modelling techniques. These and other studies have shown that likely climate corridors often include north–south river valleys, ridges, and coastlines to facilitate poleward distribution shifts, while habitat linkages that cross gradients of elevation, rainfall, and soil types will help climate adaptation across more complex landscapes.
Species with dispersal limitations and specialised interactions may not always benefit from increased connectivity. Instead, those species may rely on climate refuges—areas that are resilient to climate change and thus able to continue to support climate-sensitive communities in future. Africa offers two good examples that illustrate how climate refuges can be identified. The first study, on South African birds, identified climate refuges as areas where temperatures seldom rise above the threshold known to negatively impact a specific species’ fitness (Cunningham et al., 2013). The second study, on northern Mozambique’s coral reefs (McClanahan and Muthiga, 2017), identified two kinds of climate refuges: (a) areas where temperatures never reached a point where it would kill the corals, and (b) areas situated in deeper and cooler water but with the full spectrum of light, which allowed corals to thrive while avoiding heat stress. Both these studies highlight why protecting and restoring complex natural ecosystems (see also Betts et al. 2018) is so important for climate change mitigation.
Climate-sensitive species that are dispersal-limited may not benefit from increased connectivity. Instead, they will rely on climate refuges—areas that are resilient to climate change.
Assisted colonisation is an alternative conservation strategy to save species with dispersal limitations and specialised interactions. Also called assisted migration, assisted colonisation involves the pro-active translocation of climate-sensitive species from their present ranges to their future ranges. Sometimes, even species able to self-disperse may require assisted colonisation. For example, African penguins (Spheniscus demersus, EN) are currently undergoing population declines because of climate change-induced shifts in fish populations on which they depend for food (Sherley et al., 2017). To re-establish this important biological interaction, conservationists are currently using assisted colonisation to establish two new penguin colonies further east from existing colonies (Birdlife South Africa, 2019), in an area where fish populations have remained healthy (Figure 11.12).
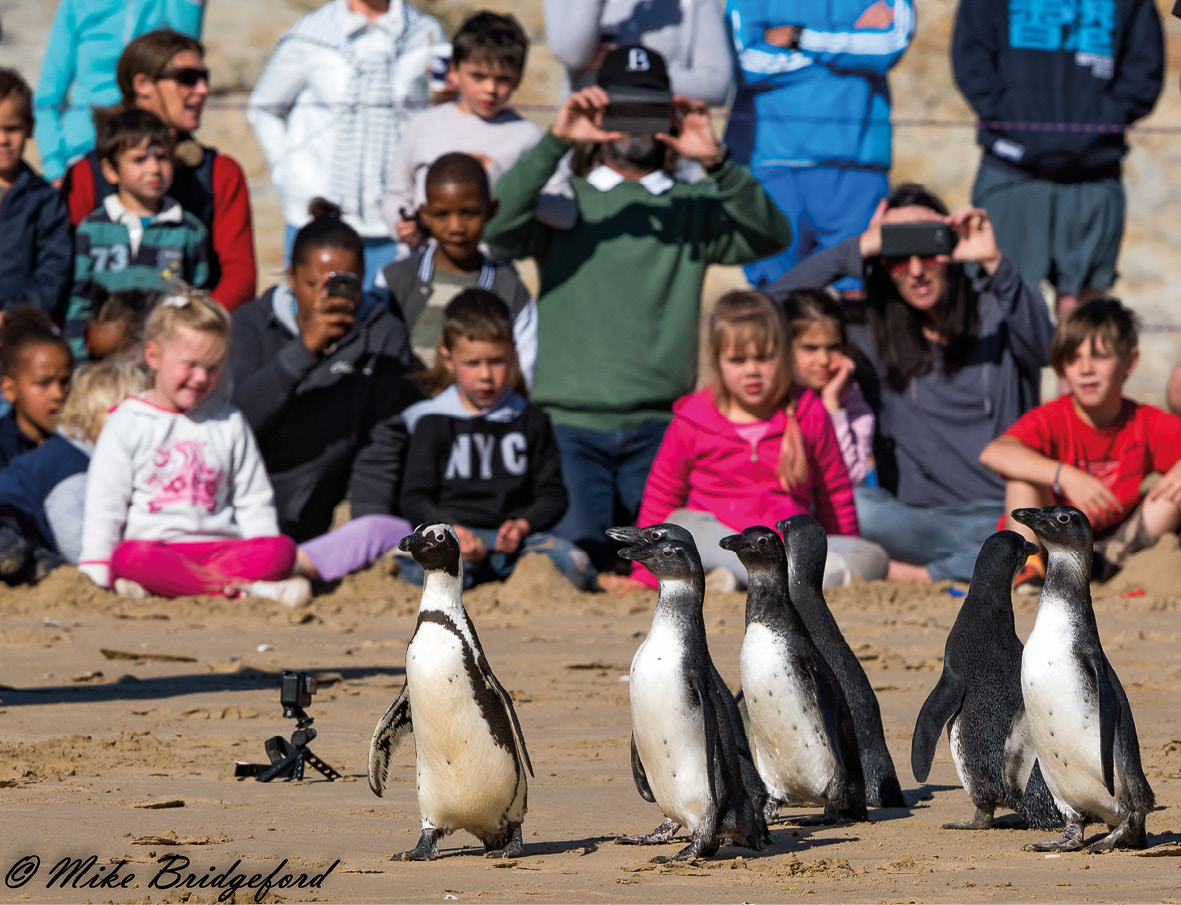
Figure 11.12 BirdLife South Africa, in partnership with CapeNature, are introducing rehabilitated African penguins to two new sites several hundred kilometres east of existing colonies. The hope is that the translocated penguins will establishing colonies that is buffered from the negative effects of climate change and fluctuating fish populations. Here members of the public are witnessing the first releases. Photograph by Michael Bridgeford, CC BY 4.0.
As with any translocation project, introducing climate-sensitive species to new areas carries significant risks, including decoupling them from critical limiting resources and symbiotic relationships. It is thus imperative to start small, by translocating only a few well-monitored individuals. If monitoring shows that the initial releases were successful, one can then plan for further releases over time. Because this strategy is still new, it is also important to disseminate your experiences to the broader conservation community, for example by presenting results at conferences or in scientific journals.
11.5 Ex Situ Conservation Strategies
The best strategy for protecting biodiversity over the long term is to protect existing wild populations in their natural ecosystems. This strategy, known as on-site, or in situ conservation, not only protect entire ecological communities—including thousands of species and their interactions—but also natural processes and ecosystem services. However, if the last populations of a threatened species are too small to remain viable, if they continue to decline despite conservation efforts, or if their threats do not subside, then in situ conservation may prove ineffective. In such cases, sometimes the only option left to prevent an imminent extinction is to capture those last remaining individuals and transfer them to a facility where they can be cared for under artificial, human-controlled conditions. This strategy is known as off-site, or ex situ conservation, and may involve individuals that were collected in the wild, orphaned, confiscated, or displaced and have nowhere else to go. Thanks to ex situ efforts, several African plants and animals that are extinct in the wild continue to survive in zoos, aquaria, and botanical gardens. Examples include four to seven species of ancient cycads (Encephalartos spp.) from Southern Africa, and the pygmy Rwandan water lily (Nymphaea thermarum, EW), which is the world’s smallest water lily (IUCN, 2019).
For species facing imminent extinction, sometimes the only option left may be to capture the remaining individuals and transfer them to captivity.
Ex situ and in situ conservation are complementary strategies (Figure 11.13; see also Conde et al., 2011). For example, many ex situ conservation programmes aim to raise enough healthy individuals to support translocation projects when appropriate habitats are available. Ex situ conservation efforts were instrumental in preventing the extinction of the live-bearing Kihansi spray toad (Nectophrynoides asperginis, EW). Populations of this Tanzanian endemic declined precipitously following the establishment of a hydroelectric dam, which caused the toad’s waterfall spray-zone habitat to dry up. The species was subsequently declared Extinct in the Wild in 2009. Tanzanian conservationists, however, demonstrated good foresight by inviting zoos from the USA to collect adults for a captive breeding effort even before the dam was built. This effort is now yielding positive results: after a decade of captive breeding, the erection of an artificial sprinkler system for habitat restoration, and experimental releases (Vandvik et al., 2014), nearly 10,000 toads were released to their former range in May 2018 (Anon, 2018).
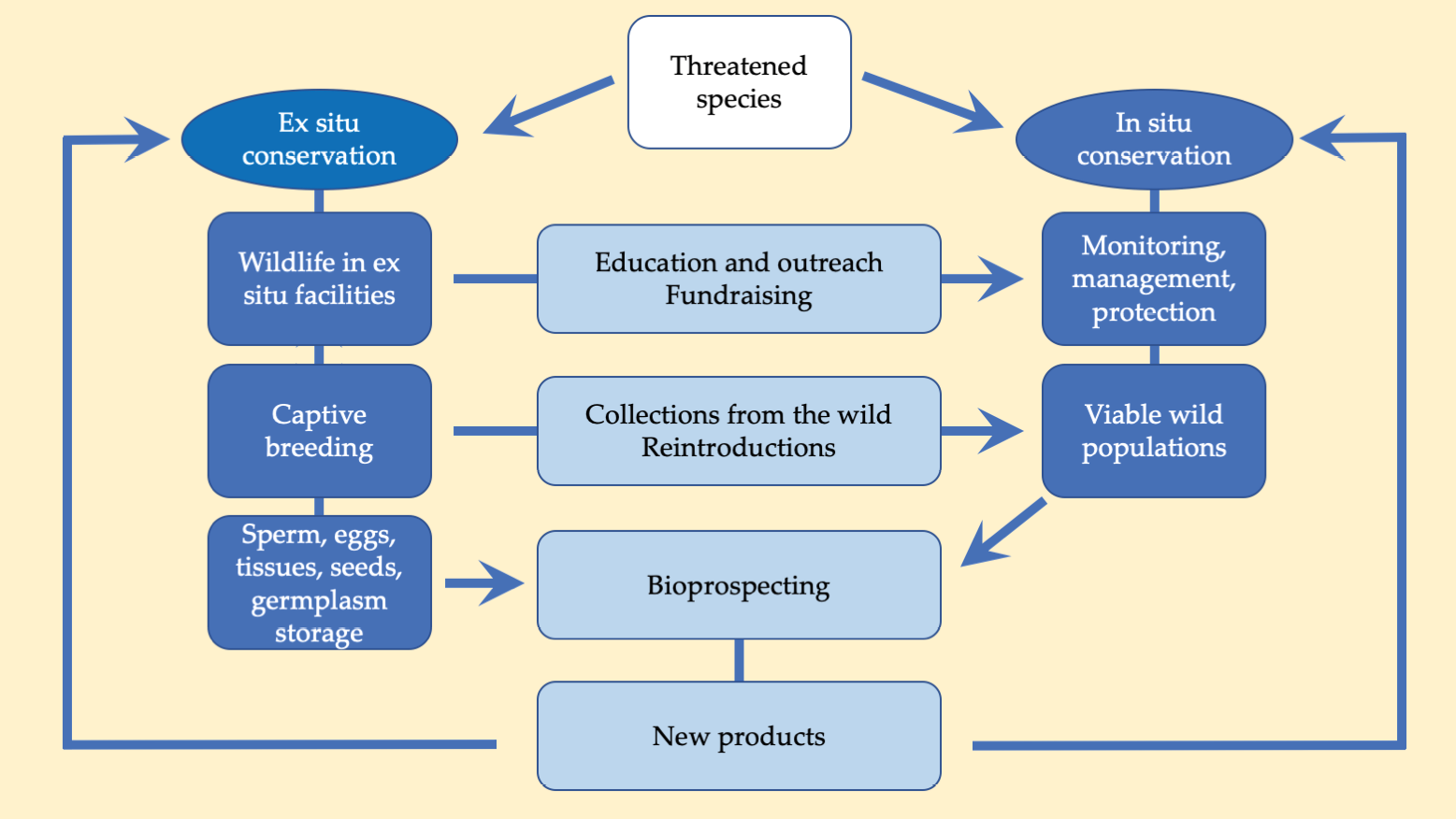
Figure 11.13 There are several ways in which in situ (on site) and ex situ (off-site) conservation can complement each other. No species conforms exactly to this idealised model, but nearly all species present some of these elements. After Maxted, 2001, CC BY 4.0.
Safeguarding a well-represented sample of the world’s biodiversity play only a small role in ex situ conservation efforts. Maintaining self-sustaining wildlife populations under human care not only reduce the need to collect individuals for research from the wild; it also allows researchers to study aspects such as physiology, genetics, and demographics of threatened species (Conde et al., 2019) using methods that might not be possible without animals in captivity. These studies can then provide knowledge and experience to help protect both ex situ and in situ populations. For example, the establishment of the Demographic Species Knowledge Index (Conde et al., 2019), summarise demographic data obtained from ex situ conservation facilities, play a crucial role in filling gaps in datasets for population viability analyses (Section 9.2) Ex situ facilities also play a critical role in captive breeding, head-starting, public outreach, education, and fundraising for in situ conservation. Many ex situ facilities have also become directly involved—and sometimes even taking leading roles—in field conservation efforts (Wilson et al., 2019). Lastly, many ex situ facilities directly connect conservation to social and economic progress through off-site education, employment, and implementation of a range of different community development activities (Ferrie et al., 2013).
Recent efforts to increase knowledge transfer among ex situ facilities has greatly enhanced their contribution to overall conservation efforts. Facilitated by organisations such as the IUCN’s Conservation Planning Specialist Group (CPSG), ex situ facilities now regularly share information on best practices for care and handling of species in human care, including aspects such as nutritional requirements, optimal housing conditions, and veterinary techniques to anaesthetize, immobilise, and reduce stress for animals when they are being moved or during medical treatments (see http://www.cpsg.org). Much of this information is stored in a central database called the Zoological Information Management System (ZIMS). Maintained by Species360, ZIMS keeps track of animal husbandry, medical, and breeding information on over 6.8 million animals belonging to more than 21,000 species for over 1,000 member institutions in 90 countries. Ex situ facilities that maintain these records and comply with operations standards in animal welfare, conservation, education, and research can also apply to become an accredited institution with the Pan-African Association for Zoos and Aquaria (PAAZA), or its parent organisation, the World Association of Zoos and Aquariums (WAZA). As of mid-2019, four Sub-Saharan African ex situ facilities were accredited by WAZA, and 19 by PAAZA.
11.5.1 Types of ex situ facilities
Many types of facilities help to preserve ex situ populations. Here we describe some of the most common, including zoos and aquaria for animals, and botanical gardens and seed banks for plants.
Ex situ conservation facilities compliment field conservation efforts through captive breeding, public outreach, education, knowledge generation, and fundraising.
Zoos around the world currently contribute to the conservation of nearly 7,000 species of terrestrial vertebrates (mammals, birds, reptiles, and amphibians) by caring for more than 500,000 individual animals. They do not do this alone; they often work with government agencies, universities, and a variety of other organisations who use zoo animals for research, education, and other conservation activities. While zoos traditionally focussed on displaying charismatic animals that draw visitors, many zoos are now also investing in the conservation of small threatened vertebrates, as well as invertebrates, such as butterflies, beetles, dragonflies, spiders, and molluscs (many of which are also cheaper to maintain). South Africa’s National Zoological Gardens, which houses more than 9,000 individual animals belonging to 705 species, is Africa’s largest zoo by variety of captive species and individuals. The zoo also hosts a variety of daily school programmes meant to inspire kids to a career in conservation; these include holiday courses, a zoo club, and guided tours at night.
Aquaria are the aquatic version of zoos, specialised in caring, displaying, and conserving marine and freshwater biodiversity, such as fishes, corals, molluscs, and crustaceans (Figure 11.14). One such institution is South Africa’s uShaka Marine World, the world’s fifth largest aquarium and home to more than 390 marine species—most from the Western Indian Ocean—held in 11 million litres of seawater. Most organisms currently in aquaria have been obtained from the wild, but conservationists are constantly refining techniques to breed more species in captivity to limit wild collecting. Recent and dramatic increases in aquaculture, which currently accounts for roughly a third of fish and shellfish production globally, have made ex situ conservation of aquatic species even more important. The hope is that these ex situ populations will help maintain genetic stocks and act as insurances against disease outbreaks introduced by domestic fish, molluscs, and crustaceans.
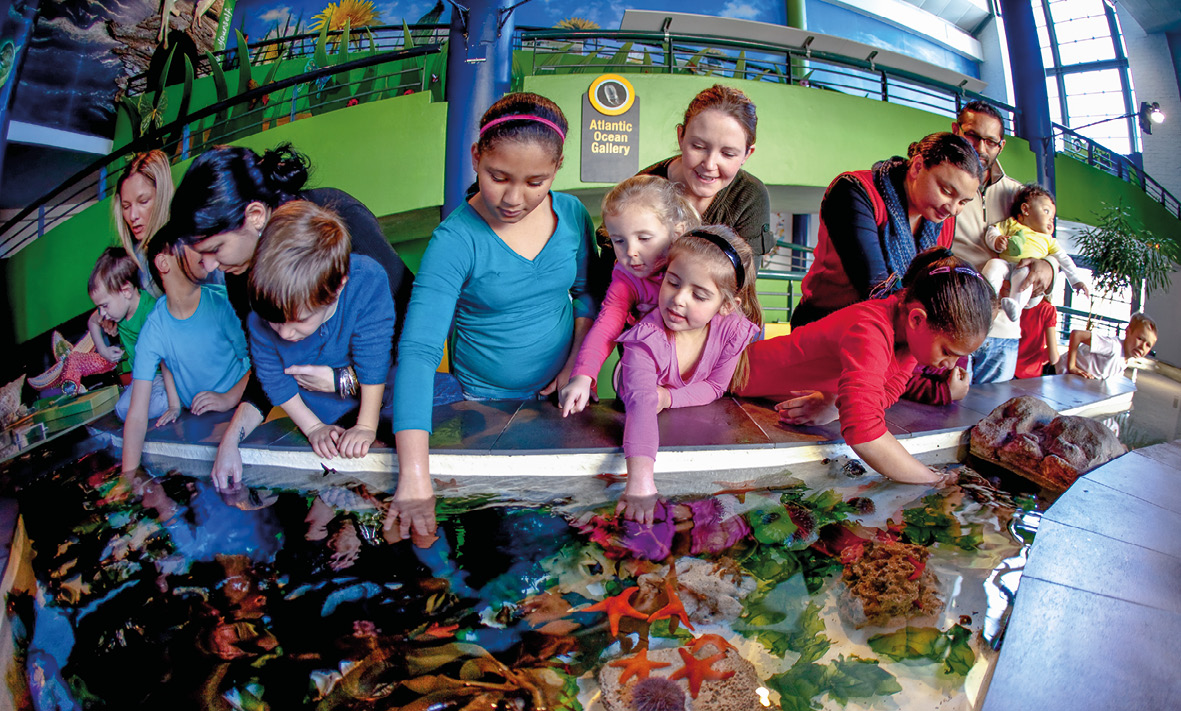
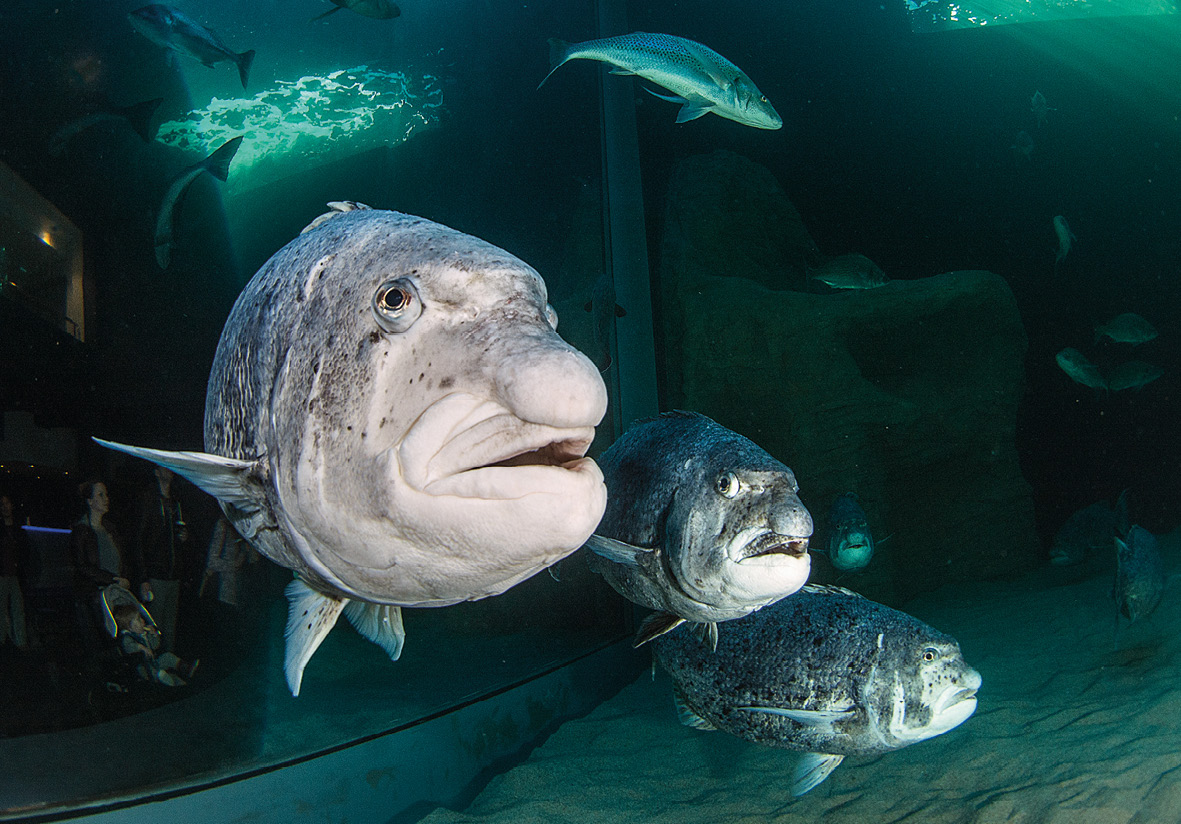
Figure 11.14 (Top) Many aquaria host tours and children’s programmes, some involving opportunities to touch the organisms, for additional enrichment to visitors. Photograph by Karen Schermbrucker, courtesy of Two Oceans Aquarium, CC BY 4.0. (Bottom) Aquaria also provide opportunities to observe species such as this black musselcracker (Cymatoceps nasutus, VU) at South Africa’s Two Oceans Aquarium, which many people would not have experienced otherwise. Photograph by Geoff Spiby, courtesy of Two Oceans Aquarium, CC BY 4.0.
Botanical gardens (and arboretums, which specialise on trees and other woody plants) are dedicated to the collection, cultivation and educational curation of living plant species. Botanical gardens across the world house more than 6 million living plants, representing over 80,000 species—approximately 25% of the world’s vascular flora (Wyse Jackson, 2001). The world’s oldest and largest botanical garden—the Royal Botanic Gardens in London, UK—maintains over 28,000 plant taxa, nearly 10% of plant taxa in the world. In Sub-Saharan Africa, there are at least 153 botanical gardens in 33 countries, which range from small community-organised centres to world-famous conservation hubs, such as South Africa’s Kirstenbosch Botanical Garden. Like zoos and aquaria, botanical gardens play a critical role in conservation efforts through public outreach and education. For example, Ghana’s Aburi Botanical Garden established a model medicinal plant garden where the public can gain first-hand knowledge on how to combine conservation, cultivation, and sustainable use of medicinal plants (Gillett et al. 2002).
A few botanical gardens and research institutes have developed collections of seeds, known as seed banks, which take advantage of the fact that seeds of most plants can survive for long periods when stored in cold, dry conditions. The seeds deposited in seed banks may be obtained from the wild, or from cultivated specimens. When gathering material from the wild, botanists generally target populations from across a species’ geographical and habitat ranges so their collections can capture as much of each species’ genetic diversity as possible. In this way, seed banks play a crucial role not only in conservation of plant species richness, but also genetic diversity. Seed banks may even be the only means some plant species are protected. Because many seeds of each species are usually collected, seed banks also provide a convenient opportunity for translocation projects. That is because safeguarded seed collections can be used to propagate not just large numbers of seedlings but, in some cases, custom-developed genetic mixtures to maximise local adaptations. The world’s largest and most diverse seed bank is the Millennium Seed Bank, UK. At the end of 2018, the Millennium Seed Bank catalogued over 2.25 billion seeds from over 39,000 species; its billionth seed, from an African bamboo, was deposited in April 2007. In addition to safeguarding a portion of plant diversity, the Millennium Seed Bank has also benefitted countries, such as Botswana, Burkina Faso, and Mali through the redistribution of banked seeds to aid ecological restoration efforts.
Seed banks contribute to conservation of genetic diversity of plants by collecting material across target species’ geographical and habitat ranges.
11.5.2 Challenges facing ex situ facilities
While the contribution of ex situ conservation facilities to overall biodiversity conservation strategies is significant (Conde et al., 2011), there are some drawbacks that need to be considered. For example, due to the limited number of individuals that can be maintained under human care, especially for larger animals, there is an increased risk that captive populations may suffer from threats facing small populations, such as inbreeding depression and demographic stochasticity (Section 8.7). There is also a concern that ex situ conservation can contribute to hybridisation concerns, for example if different cryptic species are accidentally managed as a single species. To avoid these threats, many ex-situ facilities manage their captive populations jointly as a single interbreeding metapopulation. They do this through studbooks which track the origin, pedigree, and demographic history of each individual in participating facilities. By maintaining and referring to these studbooks, ex situ conservation facilities can make informed decisions regarding transfer and breeding recommendations. The establishment of a European studbook for African dwarf crocodiles (Osteolaemus spp.) even addressed concerns about potential hybridisation between cryptic species (Schmidt et al., 2015).
Ex-situ facilities often manage captive populations as a single metapopulation using studbooks to track the origin and demographic history of breeding individuals.
Funding also remains an obstacle, given that ex situ facilities typically require large, long-term, funding commitments, in comparison to many in situ conservation activities. One consequence of funding limitations is that ex situ facilities mostly focus on showy or charismatic species that attract visitors, so small and less charismatic species are not always afforded equal protection (Brooks et al., 2009). Many ex situ facilities are also more inclined to house non-threatened species that are easier and less costly to care for, rather than threatened species with specialised needs (Table 11.1). For example, despite the fear of looming mass amphibian extinctions due to a disease caused by the chytrid fungus (Batrachochytrium dendrobatidis) (Alroy, 2015), 75% of ex situ amphibian collections consist of non-threatened species, with only 6.2% of all threatened amphibians afforded ex situ protection (Dawson et al., 2016). Neglecting threatened species in ex situ conservation efforts also creates a feedback loop, by maintaining a limited understanding on how to care for the species most in need.
Table 11.1 Number and percentages of terrestrial vertebrate species from Sub-Saharan Africa currently maintained in the world’s ex situ facilities. Values in parenthesis represent percentage of all speciesa, threatened speciesb, and CITES-listed speciesc for each taxon class, respectively.
Mammals |
Birds |
Reptiles |
Amphibians |
Total |
|
Worldwidea |
659 (55%) |
1,470 (65%) |
197 (27%) |
44 (5%) |
2,370 (47%) |
Africa |
110 (9%) |
234 (10%) |
34 (4%) |
6 (1%) |
384 (8%) |
Asia |
136 (11%) |
327 (14%) |
22 (3%) |
2 (0%) |
487 (10%) |
Oceania |
37 (3%) |
61 (3%) |
6 (1%) |
1 (0%) |
105 (2%) |
Europe |
191 (12%) |
465 (20%) |
73 (10%) |
19 (2%) |
748 (15%) |
North America |
145 (12%) |
311 (14%) |
53 (7%) |
14 (2%) |
523 (10%) |
South America |
40 (3%) |
72 (3%) |
197 (27%) |
44 (5%) |
353 (7%) |
Threatened speciesb |
45 (23%) |
42 (20%) |
22 (21%) |
8 (4%) |
117 (16%) |
Extinct in the Wild |
1 (100%) |
0 (0%) |
0 (0%) |
1 (100%) |
2 (100%) |
Critically Endangered |
7 (26%) |
4 (19%) |
5 (25%) |
3 (5%) |
19 (15%) |
Endangered |
13 (16%) |
12 (15%) |
2 (5%) |
3 (3%) |
30 (10%) |
Vulnerable |
24 (27%) |
26 (23%) |
1 (2%) |
66 (23%) |
|
CITES-listed speciesc |
95 (50%) |
121 (62%) |
45 (25%) |
1 (6%) |
262 (45%) |
Appendix I species |
30 (58%) |
4 (44%) |
8 (80%) |
1 (6%) |
43 (49%) |
Appendix II species |
58 (44%) |
112 (62%) |
37 (22%) |
0 (0%) |
207 (43%) |
Appendix III species |
7 (100%) |
5 (100%) |
0 (0%) |
0 (0%) |
12 (100%) |
Source: https://zims.species360.org, current as of April-2019. Compiled by Johanna Staerk (Species360).
Fortunately, ex situ facilities have responded to these concerns by developing several innovative mechanisms that enables them to contribute more to the conservation of threatened species. For example, ex situ facilities all agree that attracting more visitors attracts more funding. To attract more visitors, zoos and aquaria are increasingly keeping animals in enclosures that are representative of their natural environments; this keeps the animals heathier and providing more opportunities to exhibit natural behaviours which, in turn, leave visitors more satisfied. Some zoos and aquaria have also established special displays where visitors can feed, touch, or otherwise interact with animals. Many ex situ facilities have also started inviting local artists to display sculptures and other artwork, which adds to the experience for visitors and attracting people that might not otherwise have visited. A rather unusual—but very successful—attempt to increase foot traffic comes from the USA, where the California Academy of Sciences hosts dance parties with laser shows, food, and drinks every Thursday night (http://www.calacademy.org/nightlife), which visitors can enjoy while visiting the Academy’s aquarium and other conservation exhibits.
While the contribution of ex situ facilities to species conservation is significant, many rare species are ill-suited for ex situ efforts. Some species simply do not adapt or reproduce in captivity, while others that do relatively well in captivity experience behavioural and physiological changes or acquire diseases (Brossy et al., 1999) that prevent releases in the wild. Even so, the conservation biologists working at ex situ facilities constantly try to find ways to overcome these challenges. For example, staff at ex situ facilities sometimes use assisted reproductive techniques such as artificial incubation of bird and reptile eggs, or artificial insemination (Box 11.4) to overcome reproductive challenges (e.g. if individuals cannot mate because they are in different locations). Others use cryopreservation and genome resource banks for the long-term storage of embryos, eggs, sperm, or purified DNA, at least until those tissues can be used to increase a species’ genetic diversity, or perhaps even to resurrect an extinct species (see de-extinction, Section 8.8). However, many ex situ conservation techniques are difficult and expensive to implement. When possible, it is almost always preferable to preserve species in situ where they can be self-sustaining, free from inbreeding, and an interactive participant of their community and ecosystem.
Box 11.4 Saving the Northern White Rhinoceros with Assisted Reproduction Technologies
Embryo Plus,
Brits, South Africa.
http://www.embryoplus.com
A few centuries ago, Earth’s wildernesses enabled animals to roam and breed relatively freely. Today, sprawling cities, agriculture, and fences not only restrict animals’ ability to forage, but also limit reproduction between differing gene pools. These stresses create smaller and more isolated populations which are being edged toward extinction.
There are several landscape-scale conservation initiatives to counteract these imbalances. But some species and populations are so rare that they depend on intensive management to remain viable. Assisted reproductive techniques (ART) provide promise for helping such species. Over the past 30 years, ARTs have greatly enhanced how the livestock industry preserve, improve, and proliferate genetic stock. Now, efforts are also underway to use ARTs to ensure the preservation of biodiversity.
The many types of ARTs
ARTs include a wide array of medical procedures to address infertility, and to make reproduction possible between individuals unable to do so naturally (e.g. animals in different protected areas). In this way, biologists can ensure genetic exchange while eliminating the risks inherent in translocation such as spread of diseases, adaptation to new environments, and disruption of group dynamics.
ARTs have various levels from relatively simple to very complex. The most basic technique is artificial insemination. A major advantage of this technique is that it can multiply male genetic contributions by inseminating more females than would be possible in nature. Much progress has also been made in improving viability of cryopreserved semen to overcome challenges with timing of female reproductive cycles and other logistical constraints.
As for multiplying female genetic contributions, methods involve embryo transfer and in vitro fertilisation (IVF). With multiple ovulation embryo transfer (MOET) egg fertilisation occurs naturally; with IVF, it occurs in a laboratory incubator. In both cases, an embryo is eventually transferred to a surrogate mother which will carry it until birth. Scientists are currently working on improving viability of stored germplasm, so that embryos can be cryopreserved until a suitable surrogate mother is ready.
The third technique is nuclear transfer, also known as cloning. This very delicate procedure involves replacing the haploid DNA of an unfertilised egg with diploid DNA of another; cells are then cultured, after which the embryo is transferred to a surrogate mother.
Using ARTs to save the northern white rhino
Once widespread across Central Africa, poaching has pushed the northern white rhinoceros (Ceratotherium simum cottoni, EW) to the brink of extinction. Today, only two females remain, both in a semi-captive setting at Ol Pejeta Conservancy, Kenya. Incapable of natural reproduction, this species is committed to extinction without drastic intervention.
A cutting-edge initiative is currently underway to use ARTs to save this iconic species. While the project’s exact trajectory is still being developed, likely steps include optimising procedures for harvesting, maturing, and fertilising eggs, followed by embryo transfer into surrogate southern white rhinoceros. Some preliminary successes have also been achieved to generate stem cells from skin biopsies (Ben-Nun et al., 2011), which could be used in cloning. Genetic material (tissue samples and semen) of several northern white rhinos has been cryopreserved at various places around the world. However, there is a limited amount of sperm available (there are no males left), and so artificial insemination and IVF with northern white rhinoceros depends on embryo transfer successes. Many partners have been assembled to pool resources and ideas in support of this initiative, including Ol Pejeta Conservancy, Embryo Plus, Fauna & Flora International (FFI), Back to Africa, Dvur Karlove Zoo, Leibniz-IZW, Avantea, San Diego Zoo, and Kenya Wildlife Service.
Refining ARTs on other species
Before ARTs are implemented on the near-extinct rhinoceros, it is advisable to optimise procedures on another species. A logical choice would be the closely related southern white rhinoceros (C. simum simum, NT). However, the southern subspecies is also threatened, so we should look for more common mammals first.
Veterinarians at Embryo Plus routinely perform ARTs on domestic cattle, so efforts are currently focussed on building from this experience to work with wild bovines (Figure 11.D). For example, Embryo Plus recently produced the world’s first African buffalo (Syncerus caffer, NT) through IVF; the healthy calf named Pumelelo (meaning success in isiZulu) was born in June 2016. Embryo Plus has also produced several western Zambian sables (Hippotragus niger kirkii) from southern sable (H. niger niger) surrogates using embryo transfer. Plans are also underway to investigate the viability of using eland (Tautragus oryx, LC) and domestic horses (Equus ferus) as surrogate mothers for mountain bongo (T. eurycerus isaaci, CR) and Grevy’s zebra (E. grevyi, EN), respectively.
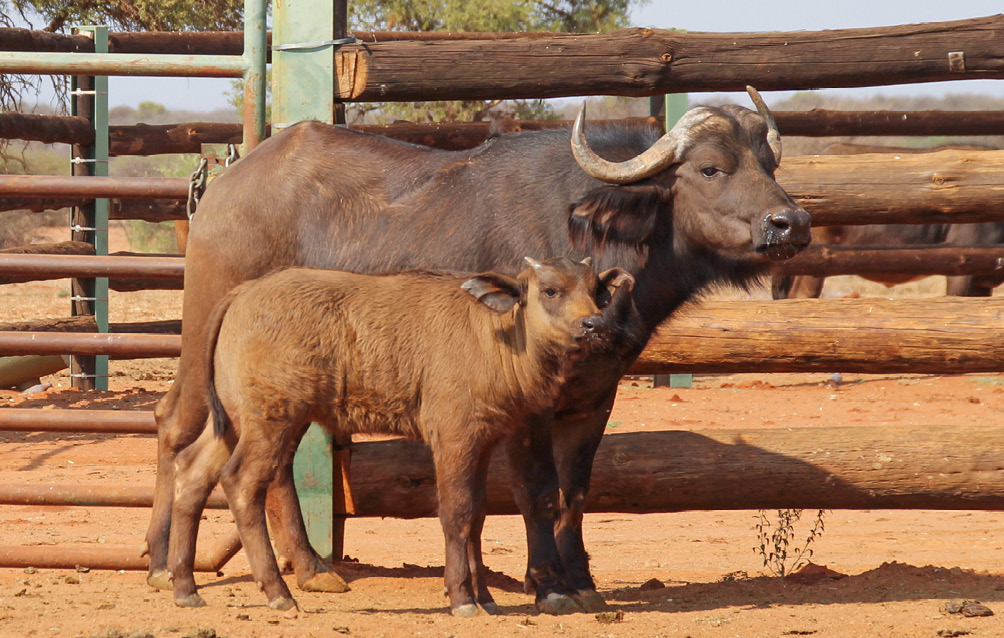
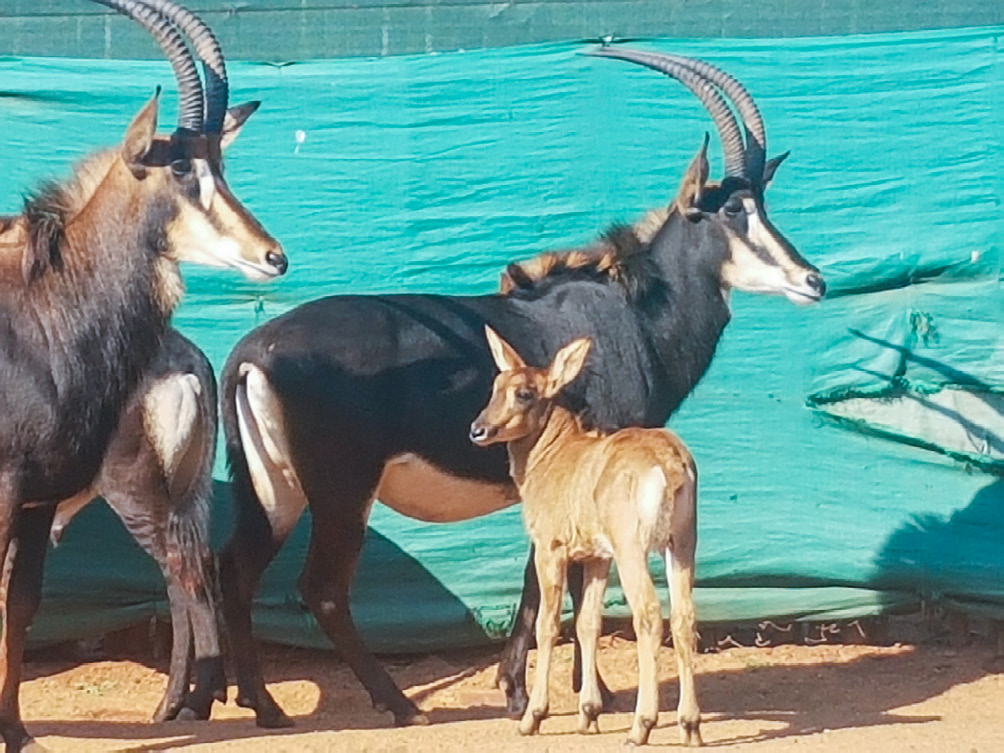
Figure 11.D (Top) The world’s first African buffalo calf conceived by in vitro fertilisation. (Bottom) The world’s first western Zambian sable born from a southern sable surrogate mother. Both species also breed successfully on their own, but scientists are refining their techniques on more common species before attempting them on highly threatened species. Photographs by Morné de la Rey/Embryo Plus, CC BY 4.0.
From dream to reality
The long-term objective of the northern white rhinoceros project is to establish a viable breeding herd which can be reintroduced into secure habitats. But much work remains for this dream to become reality. While there was one successful attempt in producing a healthy bongo calf by transferring an embryo to an eland mother (Woolf, 1986), inter-species embryo transfer remains challenging. Due to a rhinoceros’ size, we also need to ensure ART procedures can be performed safely without placing undue stress on the patient. Lastly, because each species’ embryos have different requirements in the laboratory, extensive research is necessary before ARTs can be attempted on a new species.
Although ARTs in wildlife management is still in its infancy, we are confident that early breakthroughs hold promise for the survival of the northern white rhinoceros, as well as other threatened species that may one day benefit from these procedures.
11.6 Thoughts on Neglected Taxa
Most of today’s species-centric conservation initiatives are biased towards species that are showy, charismatic, or economically important. Consequently, conservation efforts for the vast majority of taxa are neglected, particularly in Africa where conservation funding is often more limited than elsewhere. One well-known example is known as plant blindness, the perception that animals take precedence above plants in conservation efforts. This isn’t just a case of hurt feelings among botanists: there are likely significantly more plant than animal species that should be considered as threatened (see Table 2.1); however, thorough threat assessments are hampered because, as a group, plants receive significantly less funding compared to animals (Negron-Ortiz, 2014). One explanation for this disparity is that plants are often seen as the backdrop of the environment rather than the critical foundation (as primary producers) of every food web on Earth. While showy plant species may indeed have highly visible roles in maintaining the environment and regional economies, neglected species may play an equally—sometimes even more—important role in maintaining ecosystems and ecosystem services (Schleuning et al., 2016).
Fortunately, the number of professional and amateur societies interested in protecting neglected taxa, such as reptiles, amphibians, invertebrates, fungi, and plants are rising. Some groups of experts are also organised into Specialist Groups (https://www.iucn.org/ssc-groups) by the IUCN. These societies and expert groups highlight the plight of neglected taxa and are willing to provide in-house expertise on best practices for protecting those species.
11.7 Summary
- A species may be threatened by a combination of many factors, all of which must be addressed in a comprehensive conservation plan that considers its natural history.
- New populations of threatened species can be established in the wild using either captive-raised or wild-caught individuals. Animals used in translocation projects sometimes require special care and behavioural training before release as well as care and monitoring after release.
- Maintaining and facilitating movement dynamics is very important for protecting wildlife in their natural ecosystems. To do this, connectivity must be preserved by ensuring that habitat linkages such as wildlife corridors and stepping stone habitats that are intact, functional, and free from human-made obstacles.
- Preventing biodiversity losses under climate change requires ecosystem preservation, maintaining and restoring climate corridors and refugia, and assisted colonisation for species unable to adapt their ranges quick enough.
- Some species that are in danger of going extinct in the wild can be maintained in zoos, aquaria, botanical gardens, and seed banks; this strategy is known as ex situ conservation. Ex situ conservation contributes to field conservation through research, skills development, public outreach, conservation education, fundraising, captive breeding, and head-starting.
11.8 Topics for Discussion
- How do you judge whether a reintroduction project is successful? Develop simple and then increasingly detailed criteria to evaluate a project’s success.
- Tying concepts from different chapters together, what are the biggest challenges standing in the way of conserving Africa’s migratory birds?
- Use the advanced search functions on the IUCN Red List website (https://www.iucnredlist.org) to pick one species occurring in your country that is threatened by climate change. Referring to Chapter 6, how does climate change threaten this species? What strategies can be used to prevent this species’ extinction?
- What roles do ex situ facilities play in the conservation of threatened species in Africa? Discuss two or three different roles. Do you think there are certain aspects in conservation that they can make a larger contribution to than is currently the case?
- Find two or three examples of wild or semi-wild populations of African species maintained on other continents? Are those species threatened in their natural distribution ranges? Does maintaining populations of African species on other continents represent a successful conservation strategy? Explain your answer.
11.9 Suggested Readings
Hoffmann, M., J.W. Duckworth, K. Holmes, et al. 2015. The difference conservation makes to extinction risk of the world’s ungulates. Conservation Biology 29: 1303–13. https://doi.org/10.1111/cobi.12519 Conservation action does make a difference, as illustrated by ungulate conservation.
IUCN/SSC. 2013. Guidelines for Reintroductions and Other Conservation Translocations (Gland: IUCN/SSC). https://portals.iucn.org/library/efiles/documents/2013-009.pdf Guidelines for species reintroductions and translocations.
Mawdsley, J.R., R. O’Malley, and D.S. Ojima. 2009. A review of climate-change adaptation strategies for wildlife management and biodiversity conservation. Conservation Biology 23: 1080–89. https://doi.org/10.1111/j.1523-1739.2009.01264.x A review of methods to combat climate change.
Menges, E.S., S.A. Smith, and C.W. Weekley. 2016. Adaptive introductions: How multiple experiments and comparisons to wild populations provide insights into requirements for long-term introduction success of an endangered shrub. Plant Diversity 38: 238–46. https://doi.org/10.1016/j.pld.2016.09.004 Experimentation can reduce uncertainty in population translocation.
Miller, B., W. Conway, R.P. Reading, et al. 2004. Evaluating the conservation mission of zoos, aquariums, botanical gardens, and natural history museums. Conservation Biology 18: 86–93. https://doi.org/10.1111/j.1523-1739.2004.00181.x Eight tough questions directed at ex situ facilities.
Nogués-Bravo, D., D. Simberloff, C. Rahbek, et al. 2016. Rewilding is the new Pandora’s box in conservation. Current Biology 26: R87–R91. https://doi.org/10.1016/j.cub.2015.12.044 Are there limits to wildlife translocation dreams?
Pérez, I., J.D. Anadón, M. Díaz, et al. 2012. What is wrong with current translocations? A review and a decision‐making proposal. Frontiers in Ecology and the Environment 10: 494–501. https://doi.org/10.1890/110175 Important considerations for translocations.
Sherley, R.B., K. Ludynia, B.M. Dyer, et al. 2017. Metapopulation tracking juvenile penguins reveals an ecosystem-wide ecological trap. Current Biology 27: 563–68. https://doi.org/10.1016/j.cub.2016.12.054 Detecting and dealing with ecological traps requires holistic thinking.
Bibliography
Ali, A.H., A.T. Ford, J.S. Evans, et al. 2017. Resource selection and landscape change reveal mechanisms suppressing population recovery for the world’s most endangered antelope. Journal of Applied Ecology 54: 1720–29. https://doi.org/10.1111/1365-2664.12856
Alroy, J. 2015. Current extinction rates of reptiles and amphibians. Proceedings of the National Academy of Sciences 112: 13003–08. https://doi.org/10.1073/pnas.1508681112
Andersson, J., M. de Garine-Wichatitsky, D. Cumming, et al. 2013. Transfrontier Conservation Areas: People Living on the Edge (New York: Routledge).
Anon. 2018. 9,873 Kihansi spray toads return to their environment. IPP Media. https://go.shr.lc/2B6N7wB
Araújo, M.B., and M. Luoto. 2007. The importance of biotic interactions for modelling species distributions under climate change. Global Ecology and Biogeography 16: 743–53. https://doi.org/10.1111/j.1466-8238.2007.00359.x
Armstrong, D.P., and P.J. Seddon. 2007. Directions in reintroduction biology. Trends in Ecology and Evolution 23: 20–25. https://doi.org/10.1016/j.tree.2007.10.003
Ayebare, S., R. Ponce-Reyes, S.B. Segan, et al. 2013. Identifying climate resilient corridors for conservation in the Albertine Rift (Chicago: MacArthur Foundation).
Bartlam-Brooks, H.L.A., M.C. Bonyongo, and S. Harris. 2011. Will reconnecting ecosystems allow long-distance mammal migrations to resume? A case study of a zebra Equus burchelli migration in Botswana. Oryx 45: 210–16. https://doi.org/10.1017/S0030605310000414
Ben-Nun, I.F., S.C. Montague, M.L. Houck, et al. 2011. Induced pluripotent stem cells from highly endangered species. Nature Methods 8: 829–31. https://doi.org/10.1038/nmeth.1706.
Bennett, G. 2004. Linkages in practice: A review of their conservation value (Gland: IUCN). https://portals.iucn.org/library/node/8412
Bentrup, G., M. Dosskey, G. Wells, et al. 2012. Connecting landscape fragments through riparian zones. In: Forest Landscape Restoration, ed. by J. Stanturf, et al. (Dortrecht: Springer). https://doi.org/10.1007/978-94-007-5326-6
Betts, M.G., B. Phalan, S.J.K. Frey, et al. 2018. Old‐growth forests buffer climate‐sensitive bird populations from warming. Diversity and Distributions 24: 439–47. https://doi.org/10.1111/ddi.12688
BirdLife South Africa. 2019. Creating new penguin colonies. https://www.birdlife.org.za/what-we-do/seabird-conservation/what-we-do/coastal-seabird-conservation/creating-penguin-colonies/
Bonnell, T.R., R. Reyna-Hurtado, and C.A. Chapman. 2011. Post-logging recovery time is longer than expected in an East African tropical forest. Forest Ecology and Management 261: 855–64. https://doi.org/10.1016/j.foreco.2010.12.016
Boundja, R.P., and J.J. Midgley. 2010. Patterns of elephant impact on woody plants in the Hluhluwe-iMfolozi Park, Kwazulu-Natal, South Africa. African Journal of Ecology 48: 206–14. https://doi.org/10.1111/j.1365-2028.2009.01104.x
Brook, R.K., and S.M. McLachlan. 2008. Trends and prospects for local knowledge in ecological and conservation research and monitoring. Biodiversity and Conservation 17: 3501–12. https://doi.org/10.1007/s10531-008-9445-x
Brooks, T.M., S.J. Wright, and D. Sheil. 2009. Evaluating the success of conservation actions in safeguarding tropical forest biodiversity. Conservation Biology 23: 1448–57. https://doi.org/10.1111/j.1523-1739.2009.01334.x
Brossy, J.J., A.L. Plös, J.M. Blackbeard, et al. 1999. Diseases acquired by captive penguins: What happens when they are released into the wild? Marine Ornithology 27: 185–86
Brown, M., M. Perrin, and B. Hoffman. 2007. Reintroduction of captive-bred African Grass-Owls Tyto capensis into natural habitat. Ostrich 78: 75–79. https://doi.org/10.2989/OSTRICH.2007.78.1.11.55
Caro, T.J. Eadie, and A. Sih. 2005. Use of substitute species in conservation biology. Conservation Biology 19: 1821–26. https://doi.org/10.1111/j.1523-1739.2005.00251.x
Childress, B., D. Harper, B. Hughes, et al. 2004. Satellite tracking lesser flamingo movements in the Rift Valley, East Africa: Pilot study report. Ostrich 75: 57–65. https://doi.org/10.2989/00306520409485413
Conde, D.A., J. Staerk, F. Colchero, et al. 2019. Data gaps and opportunities for comparative and conservation biology. Proceedings of the National Academy of Sciences 116: 9658–64. https://doi.org/10.1073/pnas.1816367116
Conde, D.A., N. Flesness, F. Colchero, et al. 2011. An emerging role of zoos to conserve biodiversity. Science 331: 1390–91. https://doi.org/10.1126/science.1200674
Creel, S., M.S. Becker, S.M. Durant, et al. 2013. Conserving large populations of lions–the argument for fences has holes. Ecology Letters 16: 1413. https://doi.org/10.1111/ele.12145
Cumming, D.H.M. 1999. Study on development of transboundary natural resources management areas in Southern Africa — environmental context: Natural resources, land use and conservation (Washington: Biodiversity Support Programme).
Cunningham, S.J., A.C. Kruger, M.P. Nxumalo, et al. 2013. Identifying biologically meaningful hot-weather events using threshold temperatures that affect life-history. PLoS ONE 8: e82492. https://doi.org/10.1371/journal.pone.0082492
Cushman, S.A., N.B. Elliot, D.W. Macdonald, et al. 2016. A multi-scale assessment of population connectivity in African lions (Panthera leo). Landscape Ecology 31: 1337–53. https://doi.org/10.1007/s10980-015-0292-3
Dallimer, M., and N. Strange. 2015. Why socio-political borders and boundaries matter in conservation. Trends in Ecology and Evolution 30: 132–39. https://doi.org/10.1016/j.tree.2014.12.004
Dawson, J., F. Patel, R.A. Griffiths, et al. 2016. Assessing the global zoo response to the amphibian crisis through 20-year trends in captive collections. Conservation Biology 30: 82–91. https://doi.org/10.1111/cobi.12563
Deemer, B.R., J.A. Harrison, S. Li, et al. 2016. Greenhouse gas emissions from reservoir water surfaces: A new global synthesis. BioScience 66: 949–64. https://doi.org/10.1093/biosci/biw117
Dubach, J.M., M.B. Briggs, P.A. White, et al., 2013. Genetic perspectives on “Lion Conservation Units” in Eastern and Southern Africa. Conservation Genetics 14: 741–55. https://doi.org/10.1007/s10592-013-0453-3
Dupuis-Desormeaux, M., T.N. Kaaria, M. Mwololo, et al. 2018. A ghost fence-gap: Surprising wildlife usage of an obsolete fence crossing. PeerJ 6: e5950. https://dx.doi.org/10.7717%2Fpeerj.5950
Ferreira, S.M., and M. Hofmeyr. 2014. Managing charismatic carnivores in small areas: Large felids in South Africa. South African Journal of Wildlife Research 44: 32–42. https://doi.org/10.3957/056.044.0102
Ferrie, G.M., K.H. Farmer, C.W. Kuhar, et al. 2014. The social, economic, and environmental contributions of Pan African Sanctuary Alliance primate sanctuaries in Africa. Biodiversity and Conservation 23: 187–201. http://doi.org/10.1007/s10531-013-0592-3
Frankham, R., J.D. Ballou, M.D.B. Eldridge, et al. 2011. Predicting the probability of outbreeding depression. Conservation Biology 25: 465–75. https://doi.org/10.1111/j.1523-1739.2011.01662.x
Fritz, H., and P. Duncan. 1994. On the carrying capacity for large ungulates of African savanna ecosystems. Proceedings of the Royal Society B 256: 77–82. https://doi.org/10.1098/rspb.1994.0052
Giam, X., R.K. Hadiaty, H.H. Tan, et al. 2015. Mitigating the impact of oil‐palm monoculture on freshwater fishes in Southeast Asia. Conservation Biology 29: 1357–67. https://doi.org/10.1111/cobi.12483
Gillett, H. 2002. Conservation and sustainable use of medicinal plants in Ghana (Cambridge: UNEP-WCMC). https://wedocs.unep.org/bitstream/handle/20.500.11822/7487/Conservation_sustainable_use_medicinal_plants_Ghana.pdf
Godefroid, S., C. Piazza, G. Rossi, et al. 2011. How successful are plant species reintroductions? Biological Conservation 144: 672–82. https://doi.org/10.1016/j.biocon.2010.10.003
Godley, B.J., C. Barbosa, M. Bruford, et al. 2010. Unravelling migratory connectivity in marine turtles using multiple methods. Journal of Applied Ecology 47: 769–78. https://doi.org/10.1111/j.1365-2664.2010.01817.x
Gómez, C., N.J. Bayly, D.R. Norris, et al. 2017. Fuel loads acquired at a stopover site influence the pace of intercontinental migration in a boreal songbird. Scientific Reports 7: 3405. https://doi.org/10.1038/s41598-017-03503-4
Grey-Ross, R., C.T. Downs, and K. Kirkman. 2009. Reintroduction failure of captive-bred oribi (Ourebia ourebi). South African Journal of Wildlife Research 39: 34–38. https://doi.org/10.3957/056.039.0104
Groom, R.J., K. Lannas, and C.R. Jackson. 2017. The impact of lions on the demography and ecology of endangered African wild dogs. Animal Conservation 20: 382–90. https://doi.org/10.1111/acv.12328
Gusset, M., A.H. Maddock, G.J. Gunther, et al. 2008a. Conflicting human interests over the re-introduction of endangered wild dogs in South Africa. Biodiversity and Conservation 17: 83–101. http://doi.org/10.1007/s10531-007-9232-0
Gusset, M., R. Slotow, and M.J. Somers. 2006. Divided we fail: The importance of social integration for the re‐introduction of endangered African wild dogs (Lycaon pictus). Journal of Zoology 270: 502–11. https://doi.org/10.1111/j.1469-7998.2006.00168.x
Gusset, M., S.J. Ryan, M. Hofmeyr, et al. 2008b. Efforts going to the dogs? Evaluating attempts to re‐introduce endangered wild dogs in South Africa. Journal of Applied Ecology 45: 100–08. https://doi.org/10.1111/j.1365-2664.2007.01357.x
Haddad, N.M., L.A. Brudwig, A.I. Damschen, et al. 2014. Potential negative ecological effects of corridors. Conservation Biology 28: 1178–87. https://doi.org/10.1111/cobi.12323
Harris, L., E.E. Campbell, R. Nel, et al. 2014. Rich diversity, strong endemism, but poor protection: Addressing the neglect of sandy beach ecosystems in coastal conservation planning. Diversity and Distributions 20: 1120–35. https://doi.org/10.1111/ddi.12226
Hayward, M.W., J. Adendorff, L. Moolman, et al. 2007b. The successful reintroduction of leopard Panthera pardus to the Addo Elephant National Park. African Journal of Ecology 45: 103–04. https://doi.org/10.1111/j.1365-2028.2006.00673.x
Hayward, M.W., J. O’Brien, and G.I.H. Kerley. 2007a. Carrying capacity of large African predators: Predictions and tests. Biological Conservation 139: 219–29. https://doi.org/10.1016/j.biocon.2007.06.018
Henry, E., E. Brammer-Robbins, E. Aschenough, et al. 2019. Do substitute species help or hinder endangered species management? Biological Conservation 232: 127–30. https://doi.org/10.1016/j.biocon.2019.01.031
Herkt, K.M.B., G. Barnikel, A.K. Skidmore, et al. 2016. A high-resolution model of bat diversity and endemism for continental Africa. Ecological Modelling 320: 9–28. http://doi.org/10.1016/j.ecolmodel.2015.09.009
Horton, A.J., E.D. Lazarus, T.C. Hales, et al. 2018. Can riparian forest buffers increase yields from palm oil plantations? Earth’s Future 6: 1082–96. https://doi.org/10.1029/2018EF000874
Houser, A., M. Gusset, C.J. Gragg, et al. 2011. Pre-release hunting training and post-release monitoring are key components in the rehabilitation of orphaned large felids. South African Journal of Wildlife Research 41: 11–20. https://doi.org/10.3957/056.041.0111
Hudgens, B.R., W.F. Morris, N.M. Haddad, et al. 2012. How complex do models need to be to predict dispersal of threatened species through matrix habitats? Ecological Applications 22: 1701–10. https://doi.org/10.1890/11-1048.1
IUCN. 2019. The IUCN Red List of Threatened Species. http://www.iucnredlist.org
Jachowski, D.S., R. Slotow, and J.J. Millspaugh. 2013. Delayed physiological acclimatization by African elephants following reintroduction. Animal Conservation 16: 575–83. http://doi.org/10.1111/acv.12031
Jones, T., A.J. Bamford, D. Ferrol-Schulte, et al. 2012. Vanishing wildlife corridors and options for restoration: A case study from Tanzania. Tropical Conservation Science 5: 463–74. https://doi.org/10.1177/194008291200500405
Jordaan, M., A. Lubbe, C. Bragg, et al. 2017. Labeo seeberi. The IUCN Red List of Threatened Species 2017: e.T11071A100162293. http://doi.org/10.2305/IUCN.UK.2017-3.RLTS.T11071A100162293.en
Kays, R., M.C. Crofoot, W. Jetz, et al. 2015. Terrestrial animal tracking as an eye on life and planet. Science 348: aaa2478. http://doi.org/10.1126/science.aaa2478
Kearney, M., and W. Porter. 2009. Mechanistic niche modelling: Combining physiological and spatial data to predict species’ ranges. Ecology Letters 12: 334–50. https://doi.org/10.1111/j.1461-0248.2008.01277.x
King, L.E., I. Douglas-Hamilton, and F. Vollrath. 2011. Beehive fences as effective deterrents for crop-raiding elephants: Field trials in northern Kenya. African Journal of Ecology 49: 431–39. https://doi.org/10.1111/j.1365-2028.2011.01275.x
King, T., C. Chamberlan, and A. Courage. 2014. Assessing reintroduction success in long-lived primates through population viability analysis: Western lowland gorillas Gorilla gorilla gorilla in Central Africa. Oryx 48: 294–303. https://doi.org/10.1017/S0030605312001391
Laurance, S.G., and W.F. Laurance. 1999. Tropical wildlife corridors: Use of linear rainforest remnants by arboreal mammals. Biological Conservation 91: 231–39. https://doi.org/10.1016/S0006-3207(99)00077-4
Leaver, J. 2014. Options for eland: A multi-scale assessment of antipredatory responses of a vulnerable prey species to their major predator in the Eastern Cape, South Africa. MSc thesis (Port Elizabeth: NMMU). http://hdl.handle.net/10948/6570
Lindsey, P., C.J. Tambling, R. Brummer, et al. 2011. Minimum prey and area requirements of the Vulnerable cheetah Acinonyx jubatus: implications for reintroduction and management of the species in South Africa. Oryx 45: 587–99. https://doi.org/10.1017/S003060531000150X
Lindsey, P.A., R. Alexander, J.T. du Toit, et al. 2005. The cost efficiency of wild dog conservation in South Africa. Conservation Biology 19: 1205–14. https://doi.org/10.1111/j.1523-1739.2005.00088.x
Linklater, W., and A.M. Shrader. 2017. Rhino challenges: Spatial and social ecology for habitat and population management. In: Conserving Africa’s Mega-diversity in the Anthropocene: The Hluhluwe-iMfolozi Park Story, ed. by J.P.G.M. Cromsigt, et al. (Cambridge: Cambridge University Press). https://doi.org/10.1017/9781139382793
Mawdsley, J.R., R. O’Malley, and D.S. Ojima. 2009. A review of climate‐change adaptation strategies for wildlife management and biodiversity conservation. Conservation Biology 23: 1080–89. https://doi.org/10.1111/j.1523-1739.2009.01264.x
Maxted, N. 2001. Ex situ, in situ conservation. In: Encyclopedia of Biodiversity, ed. by S.A. Levin (San Diego: Academic Press).
Maxwell, S.M., G.A. Breed, B.A. Nickel, et al. 2011. Using Satellite tracking to optimize protection of long-lived marine species: Olive ridley sea turtle conservation in Central Africa. PLoS ONE 6: e19905. https://doi.org/10.1371/journal.pone.0019905
McClanahan, T.R., and N.A. Muthiga. 2017. Environmental variability indicates a climate-adaptive center under threat in northern Mozambique coral reefs. Ecosphere 8: e01812. https://doi.org/10.1002/ecs2.1812
McLennan, M.R., and A.J. Plumptre. 2012. Protected apes, unprotected forest: Composition, structure and diversity of riverine forest fragments and their conservation value in Uganda. Tropical Conservation Science 5: 79–103. https://doi.org/10.1177/194008291200500108
McNutt, J.W, M.N. Parker, M.J. Swarner, et al. 2008. Adoption as a conservation tool for endangered African wild dogs (Lycaon pictus). African Journal of Wildlife Research 38: 109–13. https://doi.org/10.3957/0379-4369-38.2.109
McPherson, J.M., W. Jetz, and D.J. Rogers. 2006. Using coarse-grained occurrence data to predict species distributions at finer spatial resolutions—possibilities and limitations. Ecological Modelling 192: 499–522. http://doi.org/10.1016/j.ecolmodel.2005.08.007
Menges, E.S., S.A. Smith, and C.W. Weekley. 2016. Adaptive introductions: How multiple experiments and comparisons to wild populations provide insights into requirements for long-term introduction success of an endangered shrub. Plant Diversity 38: 238–46. https://doi.org/10.1016/j.pld.2016.09.004
Miller, S.M., C.J. Tambling, and P.J. Funston. 2015. GrowLS: Lion (Panthera leo) population growth simulation for small reserve management planning. African Journal of Wildlife Research 45: 169–77. https://doi.org/10.3957/056.045.0169
Monadjem, A., and A. Reside. The influence of riparian vegetation on the distribution and abundance of bats in an African savanna. Acta Chiropterologica 10: 339–48. http://doi.org/10.3161/150811008X414917
Negrón-Ortiz, V. 2014. Pattern of expenditures for plant conservation under the Endangered Species Act. Biological Conservation 171: 36–43. https://doi.org/10.1016/j.biocon.2014.01.018
Newmark, W.D. 2008. Isolation of African protected areas. Frontiers in Ecology and the Environment 6: 321–28. https://doi.org/10.1890/070003
Newmark, W.D., C.N. Jenkins, S.L. Pimm, et al. 2017. Targeted habitat restoration can reduce extinction rates in fragmented forests. Proceedings of the National Academy of Sciences 114: 9635–40. https://doi.org/10.1073/pnas.1705834114
Ng’weno, C.C., N.J. Maiyo, A.H. Ali, et al. 2017. Lions influence the decline and habitat shift of hartebeest in a semiarid savanna. Journal of Mammalogy 98: 1078–87. https://doi.org/10.1093/jmammal/gyx040
Nunes, M., J.B. Adams, and G.M. Rishworth. 2018. Shifts in phytoplankton community structure in response to hydrological changes in the shallow St Lucia Estuary. Marine Pollution Bulletin 128: 275–86. https://doi.org/10.1016/j.marpolbul.2018.01.035
Packer, C., A. Loveridge, S. Canney, et al. 2013. Conserving large carnivores: Dollars and fence. Ecology Letters 16: 635–41. https://doi.org/10.1111/ele.12091
Pearson, R.G., and T.P. Dawson. 2003. Predicting the impacts of climate change on the distribution of species: Are bioclimate envelope models useful? Global Ecology and Biogeography 12: 361–71. https://doi.org/10.1046/j.1466-822X.2003.00042.x
Pearson, R.G., W. Thuiller, M.B. Araújo, et al. 2006. Model‐based uncertainty in species range prediction. Journal of Biogeography 33: 1704–11. https://doi.org/10.1111/j.1365-2699.2006.01460.x
Pérez, I., J.D. Anadón, M. Díaz, et al. 2012. What is wrong with current translocations? A review and a decision‐making proposal. Frontiers in Ecology and the Environment 10: 494–501. https://doi.org/10.1890/110175
Phillips, S.J., P. Williams, G. Midgley, et al. 2008. Optimizing dispersal corridors for the Cape Proteaceae using network flow. Ecological Applications 18: 1200–11. https://doi.org/10.1890/07-0507.1
Plumptre, A.J., S. Nixon, D.K. Kujirakwinja, et al. 2016. Catastrophic decline of world’s largest primate: 80% loss of Grauer’s gorilla (Gorilla beringei graueri) population justifies Critically Endangered status. PloS ONE 11: e0162697. https://doi.org/10.1371/journal.pone.0162697
Powell, A.N., and F.J. Cuthbert. 1993. Augmenting small populations of plovers: An assessment of cross‐fostering and captive‐rearing. Conservation Biology 7: 160–68. https://doi.org/10.1046/j.1523-1739.1993.07010160.x
Pryke, J.S., and M.J. Samways. 2012. Conservation management of complex natural forest and plantation edge effects. Landscape Ecology 27: 73–85. https://doi.org/10.1007/s10980-011-9668-1
Ralls, K., J.D. Ballou, M.R. Dudash, et al. 2018. Call for a paradigm shift in the genetic management of fragmented populations. Conservation Letters 11: 1–6. https://doi.org/10.1111/conl.12412
Reilly, S.B., J.L. Bannister, P.B. Best, et al. 2013. Eubalaena australis. The IUCN Red List of Threatened Species 2013: e.T8153A44230386. http://doi.org/10.2305/IUCN.UK.2018-1.RLTS.T8153A50354147.en
Riggio, J., and T. Caro. 2017. Structural connectivity at a national scale: Wildlife corridors in Tanzania. PLoS ONE 12: e0187407. https://doi.org/10.1371/journal.pone.0187407
Ripple, W.J., J.A. Estes, R.L. Beschta, et al. 2014. Status and ecological effects of the world’s largest carnivores. Science 343: 12341484. https://doi.org/10.1126/science.1241484
Runge, C.A., J.E.M. Watson, S.H.M. Butchart, et al. 2015. Protected areas and global conservation of migratory birds. Science 350: 1255–58. http://doi.org/10.1126/science.aac9180
SANParks. 2009. Sought-after disease-free Addo buffalo to go on auction. SANParks News. https://www.sanparks.org/about/news/?id=1246
Scheele, B.C., D.A. Hunter, L.F. Grogan, et al. 2014. Interventions for reducing extinction risk in Chytridiomycosis‐threatened amphibians. Conservation Biology 28: 1195–205. https://doi.org/10.1111/cobi.12322
Schleuning, M., J. Fründ, O. Schweiger, et al. 2016. Ecological networks are more sensitive to plant than to animal extinction under climate change. Nature Communications 7: 13965. https://doi.org/10.1038/ncomms13965
Schmidt, F., F.A. Franke, M.H. Shirley, et al. 2015. The importance of genetic research in zoo breeding programmes for threatened species: The African dwarf crocodiles (genus Osteolaemus) as a case study. International Zoo Yearbook 49: 125–36. https://doi.org/10.1111/izy.12082
Scott, J.M., D.D. Goble, J.A. Wiens, et al. 2005. Recovery of imperilled species under the Endangered Species Act: The need for a new approach. Frontiers in Ecology and the Environment 3: 383–89. https://doi.org/10.1890/1540-9295(2005)003[0383:ROISUT]2.0.CO;2
Shackeroff, J.M., and L.M. Campbell. 2007. Traditional ecological knowledge in conservation research: Problems and prospects for their constructive engagement. Conservation and Society 5: 343
Sherley, R.B., K. Ludynia, B.M. Dyer, et al. 2017. Metapopulation tracking juvenile penguins reveals an ecosystem-wide ecological trap. Current Biology 27: 563–68. https://doi.org/10.1016/j.cub.2016.12.054
Shrader, A.M., C. Bell, L. Bertolli, et al. 2012. Forest or the trees: At what scale do elephants make foraging decisions? Acta Oecologica 42: 3–10. http://doi.org/10.1016/j.actao.2011.09.009
Silber, G.K., A.S.M. Vanderlaan, A.T. Arceredillo, et al. 2012. The role of the International Maritime Organization in reducing vessel threat to whales: Process, options, action and effectiveness. Marine Policy 36: 1221–33. http://doi.org/10.1016/j.marpol.2012.03.008
Sileshi, G.W., P. Nyeko, P.O.Y. Nkunika, et al. 2009. Integrating ethno-ecological and scientific knowledge of termites for sustainable termite management and human welfare in Africa. Ecology and Society 14: 48. http://www.ecologyandsociety.org/vol14/iss1/art48
Singh, J. 1998. The lessons learnt: The development and management of transboundary parks world-wide. In: Study on the Development and Management of Trans-boundary Conservation Areas in Southern Africa (Gabarone: RCSA).
Stears, K., and A.M. Shrader. 2015. Increases in food availability can tempt oribi antelope into taking greater risks at both large and small spatial scales. Animal Behaviour 108: 155–64. http://doi.org/10.1016/j.anbehav.2015.07.012
Tambling, C.J., D.J. Druce, M.W. Hayward, et al. 2012. Spatial and temporal changes in group dynamics and range use enable anti-predator responses in African buffalo. Ecology 93: 1297–304. https://doi.org/10.1890/11-1770.1
Tambling, C.J., J.W. Wilson, P. Bradford, et al. 2014. Fine-scale differences in predicted and observed cheetah diet: Does sexual dimorphism matter? South African Journal of Wildlife Research 44: 90–94. https://doi.org/10.3957/056.044.0109
Tambling, C.J., S.M. Ferreira, J. Adendorff, et al. 2013. Lessons from management interventions: Consequences for lion-buffalo interactions. South African Journal of Wildlife Research 43: 1–11. https://doi.org/10.3957/056.043.0116
Thomas-Blate, J. 2017. Celebrating a great year for dam removals in 2016. American Rivers. https://www.americanrivers.org/2017/02/celebrating-great-year-dam-removal-2016
Tolley, K.A., G.J. Alexander, W.R. Branch, et al. 2016. Conservation status and threats for African reptiles. Biological Conservation 204: 63–71. http://doi.org/10.1016/j.biocon.2016.04.006
Valutis, L.L., and J.M. Marzluff. 1999. The appropriateness of puppet‐rearing birds for reintroduction. Conservation Biology 13: 584–91. https://doi.org/10.1046/j.1523-1739.1999.97443.x
van Andel, T.R., S. Croft, E.E. van Loon, et al. 2015. Prioritizing West African medicinal plants for conservation and sustainable extraction studies based on market surveys and species distribution models. Biological Conservation 181: 173–81. https://doi.org/10.1016/j.biocon.2014.11.015
Vandvik, V., I.E. Måren, H.J. Ndangalasi, et al. 2014. Back to Africa: Monitoring post-hydropower restoration to facilitate reintroduction of an extinct-in-the-wild amphibian. Ecosphere 5: 1–16. https://doi.org/10.1890/ES14-00093.1
von der Heyden, S. 2009. Why do we need to integrate population genetics into South African marine protected areas planning? African Journal of Marine Science 31: 263–69. https://doi.org/10.2989/AJMS.2009.31.2.14.886
Vosse, S., K.J. Esler, D.M. Richardson, et al. 2008. Can riparian seed banks initiate restoration after alien plant invasion? Evidence from the Western Cape, South Africa. South African Journal of Botany 74: 432–44. https://doi.org/10.1016/j.sajb.2008.01.170
Wassenaar, T.D., R.J. van Aarde, S.L. Pimm, et al. 2005. Community convergence in disturbed subtropical dune forests. Ecology 86: 655–66. https://doi.org/10.1890/03-0836
Wegmann M, L. Santini, B. Leutner, et al. 2014. Role of African protected areas in maintaining connectivity for large mammals. Philosophical Transactions of the Royal Society B 369: 20130193. https://doi.org/10.1098/rstb.2013.0193
White, P.S. 1996. Spatial and biological scales in reintroduction. In: Restoring Diversity: Strategies for Reintroduction of Endangered Plants, ed. by D.A. Falk, et al. (Washington: Island Press).
Williams, P., L. Hannah, S. Andelman, et al. 2005. Planning for climate change: Identifying minimum‐dispersal corridors for the Cape proteaceae. Conservation Biology 19: 1063–74. https://doi.org/10.1111/j.1523-1739.2005.00080.x
Wilson, J.W., R. Bergl, L.J. Minter, et al. 2019. The African elephant Loxodonta spp. conservation programmes of North Carolina Zoo: Two decades of using emerging technologies to advance in situ conservation efforts. International Zoo Yearbook 53: in press. https://doi.org/10.1111/izy.12216
Wilson, J.W., R.L. Stirnemann, Z.S. Schaikh, et al. 2010. The response of small mammals to natural and human-altered edges associated with Afromontane forests of South Africa. Forest Ecology and Management 259: 926–31. http://doi.org/10.1016/j.foreco.2009.11.032
Wimberger, K., C.T. Downs, and M.R. Perrin. 2009. Two unsuccessful reintroduction attempts of rock hyraxes (Procavia capensis) into a reserve in the KwaZulu-Natal Province, South Africa. South African Journal of Wildlife Research 39: 192–201. https://doi.org/10.3957/056.039.0213
Wimberger, K., C.T. Downs, and R.S. Boyes. 2010. A survey of wildlife rehabilitation in South Africa: Is there a need for improved management? Animal Welfare 19: 481–99.
Winemiller, K.O., P.B. McIntyre, L. Castello, et al. 2016. Balancing hydropower and biodiversity in the Amazon, Congo, and Mekong. Science 351: 128–29. http://doi.org/10.1126/science.aac7082
Woolf, N.B. 1986. New hope for exotic species. BioScience 36: 594–97. https://doi.org/10.2307/1310192.
Wyse Jackson, P.S. 2001. International review of the ex situ plant collections of the botanic gardens of the world. Botanic Gardens Conservation News 3: 22–33.