4. Why Should We Protect Biodiversity?
© 2019 J.W. Wilson and R.B. Primack, CC BY 4.0 https://doi.org/10.11647/OBP.0177.04
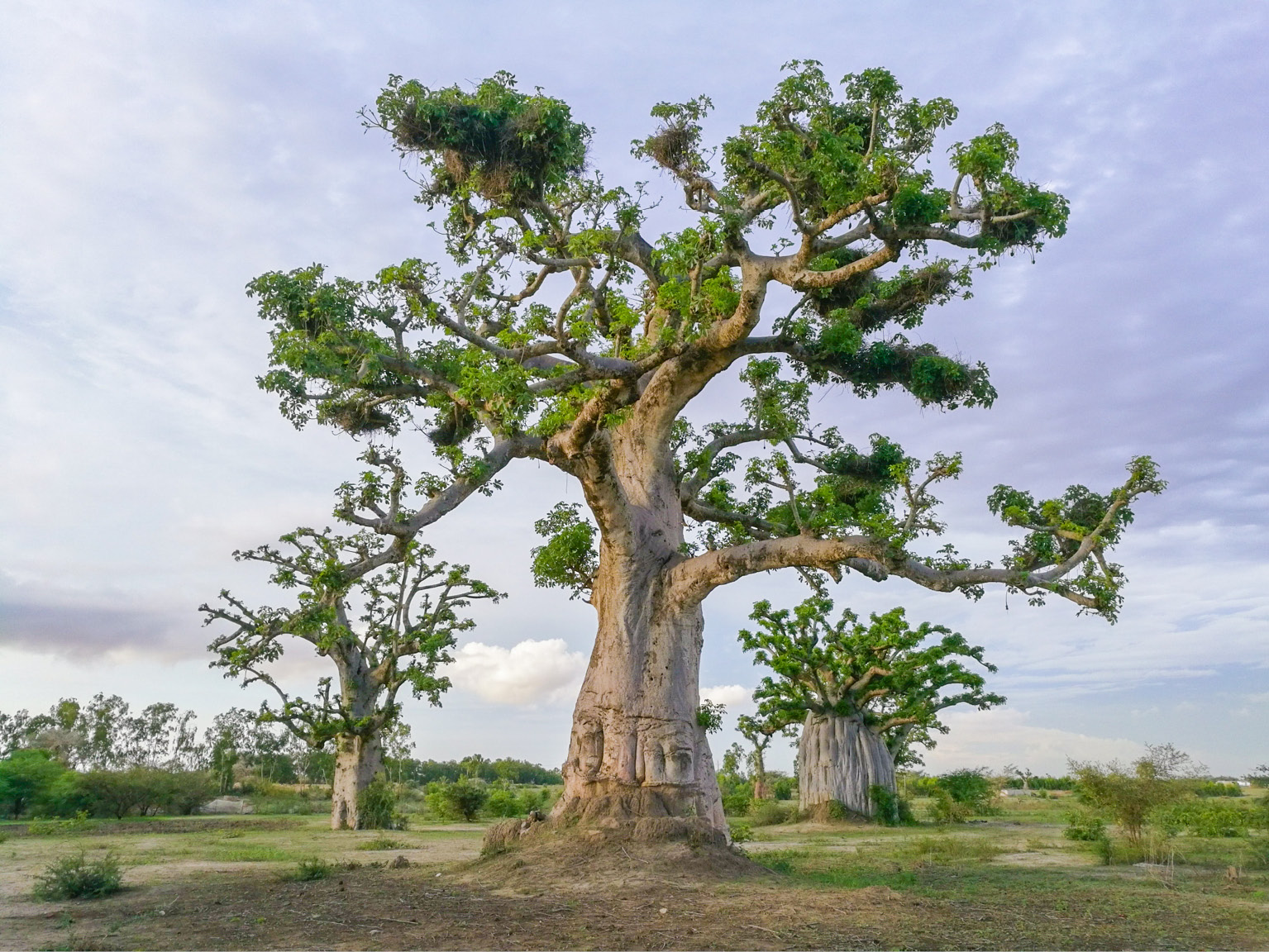
The charismatic baobab (Adansonia digitata) standing tall outside Dakar, Senegal. Sometimes called the “Tree of Life” for its enormous value to humans, the baobab is also a keystone species. In addition to providing food for a great number of species, baobabs also provide an important refuge for several bat and bird species that exclusively use these trees for roosting, nesting, and breeding. Photograph by Mattia Menchetti, https://www.inaturalist.org/photos/24373230, CC BY-SA 4.0.
All of us depend on nature for survival, whether we live off the land, or in a city where we can buy natural resources, transported to us from a distance, at the market. When we do not take care of nature, our quality of life suffers. To illustrate this point, in the book, Collapse (2011), prize-winning author Jared Diamond describes how, throughout history, ineffective responses to ailing environments have contributed to human conflicts. In one case study, Diamond examines how overpopulation contributed to Rwanda’s collapse into genocide in the early 1990s. Prior to the genocide, Rwanda had one of the highest human population densities in the world, putting enormous strain on its natural resources. Widespread deforestation led to erosion, which in turn contributed to famine, further escalating conflict over what resources remain.
Rwanda—situated in the Albertine Rift Biodiversity Hotspot—is not the only country in which environmental degradation has led to human pain and suffering. Between 1950 and 2000, 80% of the world’s armed conflicts occurred within the boundaries of the world’s 36 Global Biodiversity Hotspots (Hanson et al., 2009)—areas with high levels of biodiversity that also suffer from substantial environmental degradation. Even today, environmental degradation continues to play a major role in fuelling ongoing conflicts, such as those of the Middle East (Gleick, 2014), West Africa’s Sahel region (Benjaminsen, 2008), and the Horn of Africa (Markakis, 1995). Preventing these conflicts, which also impact biodiversity negatively (Nackoney et al., 2014; Brito et al., 2018; Daskin et al., 2018), from escalating and new conflicts from developing requires political and societal changes. People in government and local communities must recognize the value of healthy ecosystems and become their champions. After all, complex and adaptive ecosystems provide jobs, food, and other resources, thereby contributing to our overall well-being.
A healthy environment improves our overall wellbeing by enabling us to live healthy and prosperous lives. In other words, it is our life support system.
But what exactly are we losing when we fail to protect biodiversity? Why should we care if a species goes extinct, or an ecosystem becomes degraded? What evidence do we have that the natural world is our life support system? To better understand the importance of biodiversity for human well-being and quality of life, and the variety of benefits people freely gain from biodiversity, the UN brought together a group of leading scientists to study nature’s contributions to people (NCP, Díaz et al., 2018), more commonly referred to as ecosystem services. This group, called the Intergovernmental Science-Policy Platform on Biodiversity and Ecosystem Services (IPBES), recognises three categories of ecosystem services, namely material contributions, regulation services, and nonmaterial contributions. Note that there are broad overlaps and interdependence among the three categories; consequently, some contributions and services can easily fit under more than one category.
4.1 Material Contributions
Nature’s material contributions to people, also called provisioning services, commodity values or direct use values, represent contributions derived from the direct extraction and physical consumption of natural resources (Figure 4.1). This category is often the most visible and marketed of all ecosystem services. Also, because of their important contribution to the economy, economists are often interested in calculating the values of material contributions and associated services, which they do by monitoring the cost of each product at several points along its life cycle, as well as the behaviours of target groups of people.
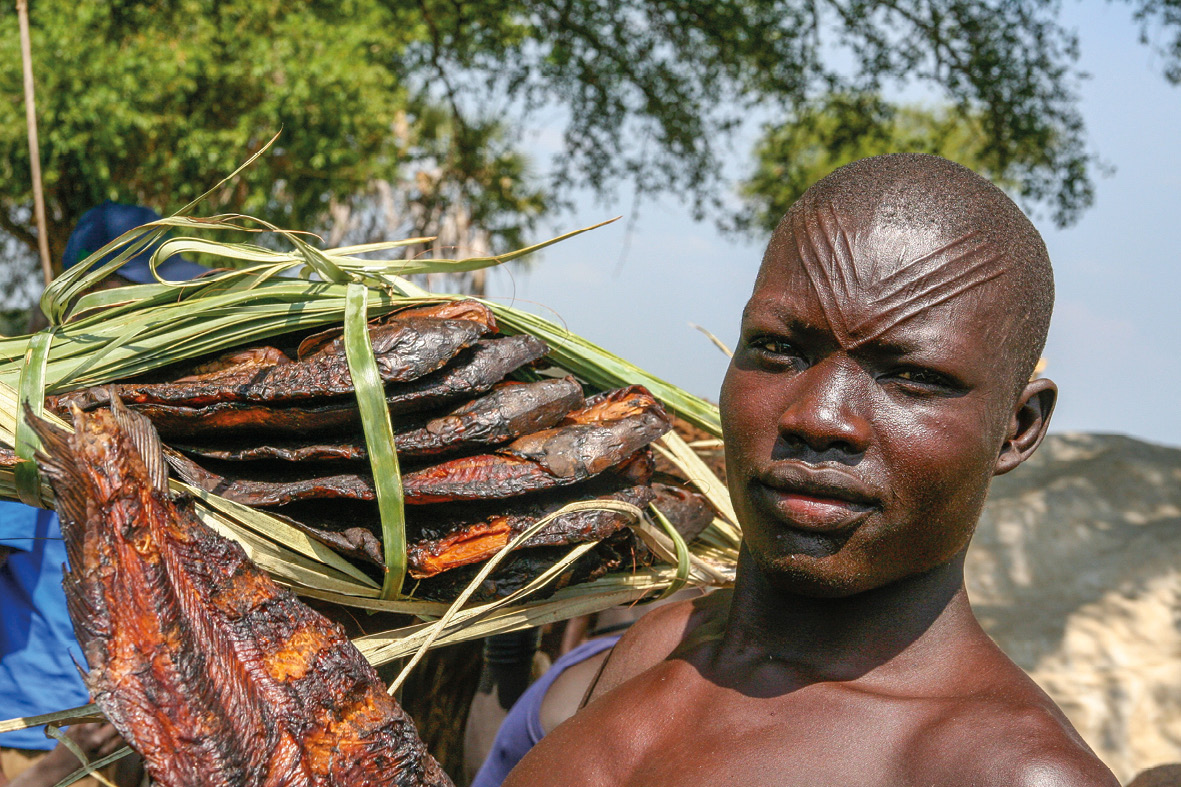
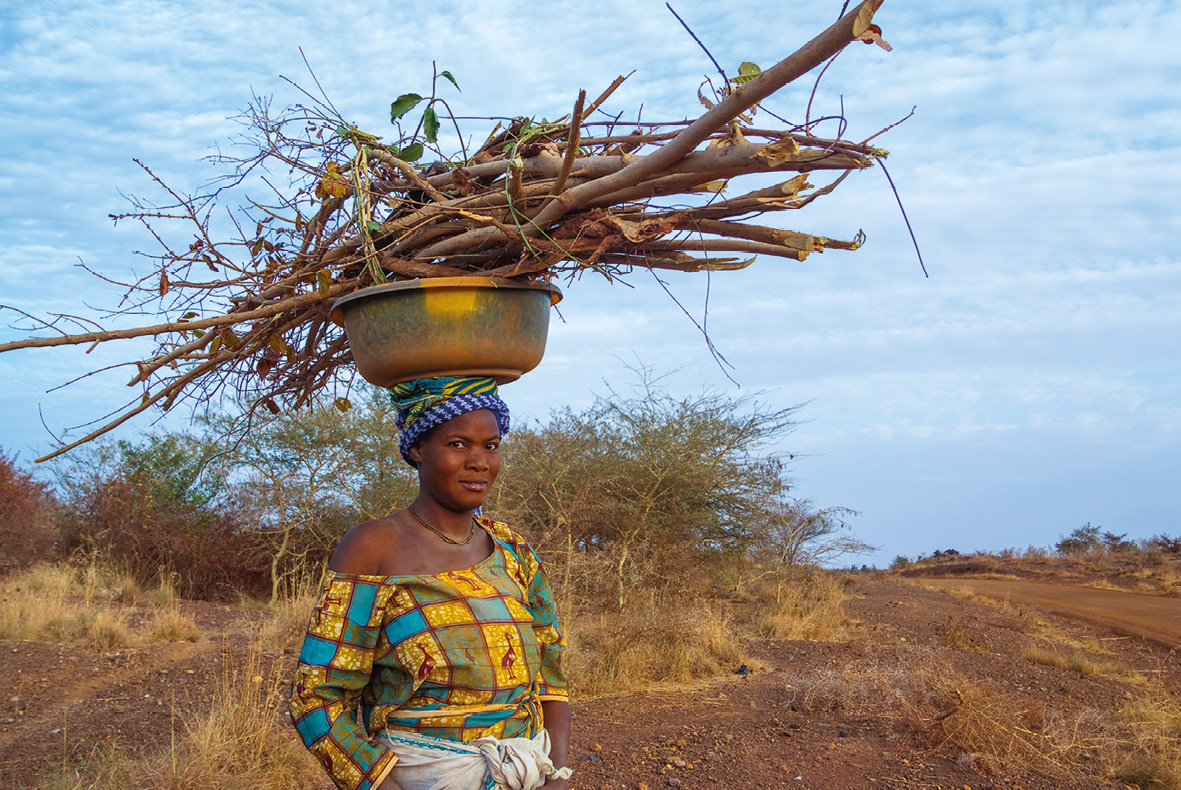
Figure 4.1 (Top) A Mandari fisherman from South Sudan carrying smoked fish to the local market. Photograph by Leonard Tedd/DFID, https://www.flickr.com/photos/dfid/8379215187, CC BY-SA 2.0. (Bottom) A lady from Burkina Faso returns to her village with a bundle of firewood for cooking. Photograph by Jose Navarro, CC BY 4.0.
Material contributions can be subdivided into four subcategories. The first subcategory is energy resources, such as firewood and biofuels. The second is food resources, such as drinking water, bushmeat, and edible fruit. The third is materials, companionship, and labour, which include natural products used to make clothes, ornamental resources used for decorations, and animals used for biomedical research, as pets, and for labour. The fourth is medicinal, biochemical, and genetic resources, which include medicinal plants used to cure ailments, psychoactive fungi used in spiritual ceremonies, and genetic stocks used to improve crops.
Many people, especially those in rural areas, obtain many of the material contributions they need for survival from the surrounding environment. These products, which include bushmeat, perfumes from aromatic plants, and firewood, are often assigned to consumptive use values. In contrast, material contributions that are sold at commercial markets, whether locally or internationally, are assigned to productive use values. Because of material contributions’ importance in sustaining people’s material assets and health, it is important to ensure that these products are sustainably harvested (Box 4.1).
Box 4.1 Research on Hunting Underpins Conservation in Central Africa
1Biological and Environmental Sciences, Faculty of Natural Sciences,
University of Stirling, UK.
2Institut de Recherches en Ecologie Tropicale, CENAREST,
Gros Bouquet, Libreville, Gabon.
3CIFOR, Jalan CIFOR Situ Gede,
Sindang Barang Bogor (Barat) 16115, Indonesia.
k.a.abernethy@stir.ac.uk
A major threat to wildlife in Africa is hunting. Subsistence hunting has been practiced for thousands of years, but new technologies allow hunters to have higher impacts than they had in the past. Improved access routes and vehicles allow hunters to cover more ground and sell to a greater client base, while habitat encroachment from logging or agriculture squeezes wildlife into smaller areas. Growing human populations are pushing the overall demand for wildlife products to a level that the remaining fauna simply cannot support. Yet wild meat is a critically important resource in rural Central Africa, so managing hunting is an important issue for conservation and human welfare (Coad et al., 2010).
Our 20-year research programme looked at hunting in Central Africa to determine how conservation may be most effective. We studied how human communities rely on hunting, impacts of hunting on wildlife and ecosystems, law enforcement challenges, and alternative practices. We found that across Central Africa hunters are in the poorer sections of society and hunt for very similar reasons: food and income. In rural villages, most able-bodied men hunt, but usually < 10% of men make most kills and have disproportionately important impacts on wildlife. These hunters have invested most in equipment and local assets; thus, they have the most to lose and are resistant to regulations or alternatives. The more successful a hunter, the more meat he sells (Coad et al., 2013). Only around 40–60% of hunted meat is consumed directly within the community; smoked or frozen meat can be traded up to 1,000 km away. Even remote villages now trade meat as a commodity to buy supplies such as fuel and medicines.
Under subsistence-driven hunting, studies show that larger-bodied species (> 20 kg) are targeted first. As these decline, smaller species are hunted (Ingram et al., 2015). During this process, the wildlife community changes and, as large predators, browsers, and seed dispersers are lost, ecosystem functioning is compromised (Abernethy et al., 2013).
Commercial hunting often targets illegal trophies, which is only lucrative if hunters have access to clients. These illegal hunters are often recruited directly by the buyer and local people may not necessarily participate, or even benefit at all. If profits are high, hunters can access better weaponry and surveillance than law enforcers, making them difficult and dangerous to apprehend. In the past 20 years, species such as elephants, rhinoceros, lions, and gorillas, have suffered drastic declines that authorities have not been able to combat.
Although wildlife protection laws are generally strong in the region, law enforcement is underfunded and complex. Commercial hunting is regulated but subsistence hunting is allowed, making the identification of illegal hunting difficult as most hunters sell only part of their catch. Alternative livelihood projects have been promoted in the hope of reducing hunting without complex enforcement. However, our review of these projects shows negligible impact, as they have generally been on a small scale and were often unreliable in generating better revenues than hunting (Wicander and Coad, 2015).
Our research shows that the effective regulation of hunting is desperately needed to preserve Central Africa’s ecosystems and the sustainability of rural communities. This will require balancing law enforcement and long-term community outreach with policy interventions—such as lobbying—to change laws or awareness campaigns. A conservation practitioner tasked with trying to manage hunting should ask who hunts, why they hunt, where hunting pressure is greatest, and how hunting affects the local ecosystem in order to determine whether they are tackling a subsistence issue or a commercially-driven one, and from there to decide which strategies could be used and who the interventions will affect. This will help to ensure planning for fair, long-term solutions, which have broad local support and the best chance of success.
4.2 Regulating Services
Regulating services maintain nature’s ability to provide material contributions, usually in indirect ways. For example, our ability to obtain food indirectly depends on a variety of subtle yet important ecosystem processes (e.g. energy cycling), as well as more observable services (e.g. pollination). For that reason, regulation services are sometimes called indirect use values. Regulating services (together with nonmaterial contributions, discussed below) are also sometimes referred to as non-consumptive use values because they provide economic benefits without needing to be collected, harvested, consumed, converted, or destroyed during use.
The economic benefits we gain from regulating services are estimated to be larger than all the different kinds of material contributions together.
The economic benefits we gain from regulating services are estimated to be larger than all the different kinds of material contributions together, especially in areas where ecosystems are intact (Costanza et al., 2014). Even so, these benefits do not always appear in descriptions of national economies because those statistics generally focus on material contributions. Nonetheless, maintaining regulating services is very important. When damaged ecosystems cannot provide these benefits, substitute resources must be found—often at great expense—to avoid economic collapse. In Section 4.2.4, we discuss one such example, by considering the value and replacement costs of water maintenance services obtained from forests.
Regulating services can be subdivided into many different subcategories depending on context, each overlapping to varying degrees with one another. Following is a discussion of some prominent subcategories of regulating services.
4.2.1 Maintaining ecosystem stability
Perhaps the most important indirect contribution we gain from biodiversity is its ability to maintain conditions that enable life on Earth to persist. This principle complements the Gaia hypothesis, which proposes that all the biological, physical, and chemical properties on Earth interact to form a complex, self-regulating superorganism, and that these interactions maintain the conditions and processes necessary for life to persist (Lovelock, 1988).
There are two complementary theories that explain the importance of maintaining a variety of different species if one is to conserve this superorganism (Ehrlich and Walker, 1998). Originally proposed by American ecologist Paul Ehrlich, the rivet-popper hypothesis compares biodiversity to the rivets (some of which may be redundant) that hold an aeroplane together. Just as an aeroplane can only lose so many rivets before it falls apart, so will the progressive loss of species systematically weaken an ecosystem until the entire system collapses. A well-known example of the rivet-popper hypothesis is the mutualistic relationships many plants have with all the various pollinators and seed dispersers (Section 4.2.5), in this context representing the rivets holding the system together. We might not immediately notice the systematic loss of pollinators we are currently experiencing (Gallai et al., 2008; Dirzo et al, 2014), but eventually these losses will catch up with us, perhaps in the form of food insecurity.
Keystone species provide such an outsized contribution to ecosystem functioning that their loss will greatly alter ecosystem composition and functioning.
The species redundancy hypothesis, proposed by African ecologist Brian Walker, holds that biodiversity and ecosystem stability is best maintained not by focussing on preserving individual species, but by preserving redundancy in ecosystem functioning, by ensuring that each ecosystem is composed of a variety of (seemingly redundant) species performing similar roles. In other words, we should not focus our efforts on protecting just one or two important pollinating species, but a variety of them, to ensure that a variety of plants (and hence entire ecosystems) can also continue to survive. In this way, if one pollinator is lost due to an environmental disturbance or disease, the system will not collapse because other pollinating species will be able to compensate for the loss of that one species.
It is important to note that there are some individual species that provide such an outsized contribution to ecosystem functioning that their loss will greatly alter ecosystem composition and functioning. These “pilots” of natural ecosystems are generally known as keystone species (Figure 4.2). The keystone species concept was originally proposed after scientists observed that removing sea stars from intertidal zones allowed their prey (mussels) to increase uncontrollably which, in turn, pushed species, such as sea urchins and other shellfish, away, leaving an overall poorer ecosystem (Paine, 1969). Apex predators, such as lions (Panthera leo, VU) and cheetahs (Acinonyx jubatus, VU), are also keystone species because of their role in keeping herbivore populations under control. If these apex predators were to disappear, increasing herbivore populations would lead to overgrazing, and ultimately also herbivore declines. This top-down control predators exert on herbivores also answers one of modern ecology’s oldest questions: “why is the world green?” (Hairston et al., 1960).
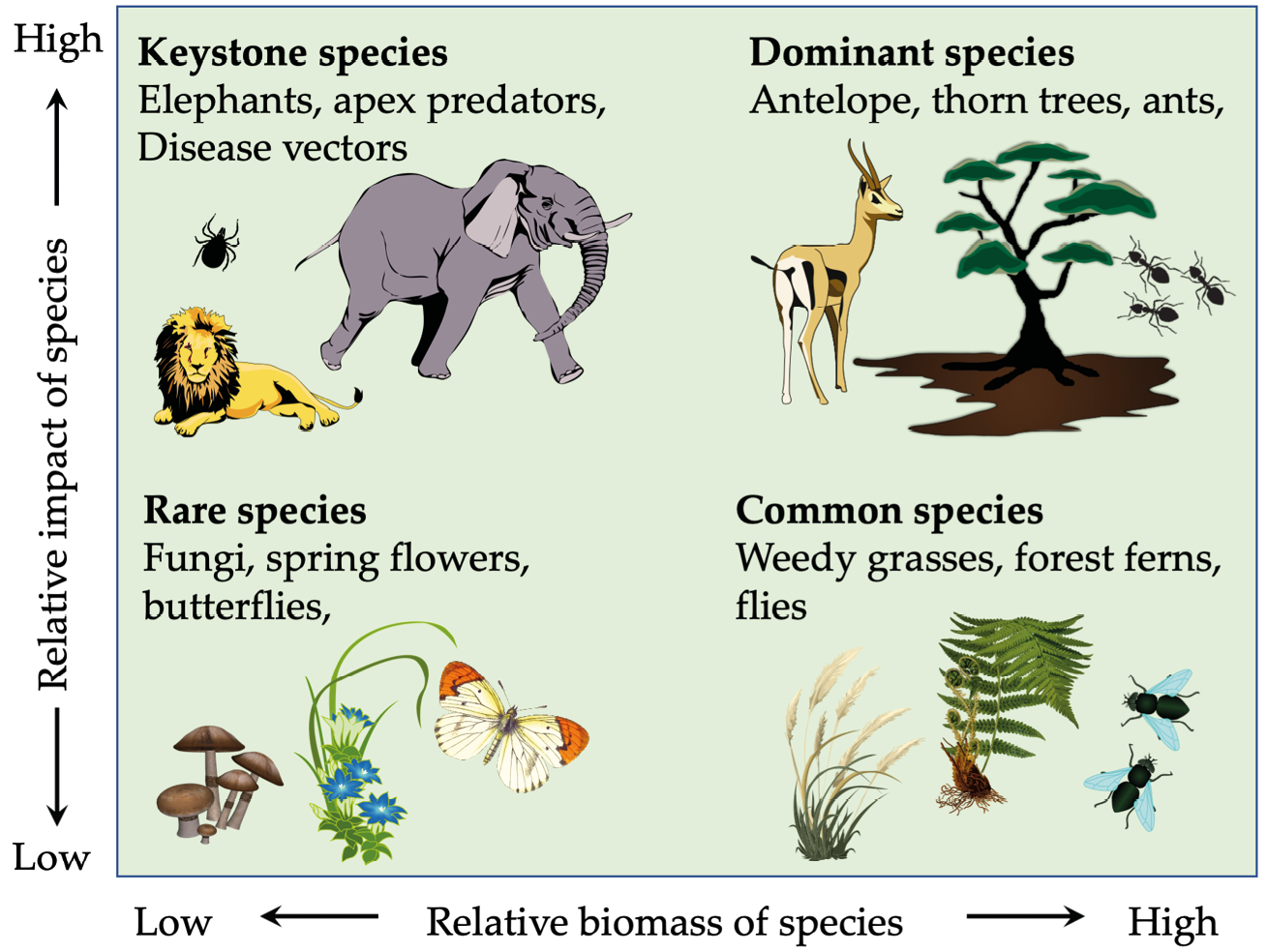
Figure 4.2 Although keystone species constitute only a small proportion of their ecosystem’s overall living biomass, they have such disproportionately important roles that their disappearance would lead to drastic environmental changes. This contrasts with rare species that constitute a low proportion of overall biomass and have a minimal impact on their ecosystems’ organisation. Like keystone species, dominant species have a significant impact on their environment; however, they also make up a large proportion of an area’s living biomass. Common species, in turn, have a relatively minimal impact on their communities despite making up a large proportion of the living biomass. After Power et al., 1996, CC BY 4.0.
An ecosystem engineer is a special type of keystone species that extensively modifies the physical environment, thereby creating and maintaining habitats for other species. Mount-building termites are important ecosystem engineers in many African ecosystems because their activities alter physical, chemical, and biological soil properties (Jouquet et al., 2011), and their massive mounts (some mounts are 10 m high, 20 m across, and may be over 2,000 years old) support distinctive ecological communities and serve as refuges for a large variety of animals and even plants (Loveridge and Moe, 2004; van der Plas et al, 2013). Elephants are also ecosystem engineers; their dramatic foraging habit of pushing over trees provides suitable habitats to countless small animals (Pringle, 2008). Elephants also open up dense vegetation, which allows grasses to thrive, in turn providing food for grazing antelope (Valeix et al., 2011). Holes dug by elephants sometimes make water more accessible, while elephant dung provides food for butterflies and dung beetles and creates an important germination environment for seeds and fungi. But too many elephants can also damage ecosystems by reducing the number of large trees on which other species depend (Cumming et al., 1997). It is important to remember that water is an important limiting resource for elephants (Chamaillé-Jammes et al., 2008), so there is a greater risk for elephants to become overly destructive in areas where humans artificially increase aboveground water availability.
Because so many species depend on ecosystem engineers and other keystone species for survival, their disappearance from an ecosystem can create an extinction cascade—a series of linked extinction events following one another. A related phenomenon known as a trophic cascade describes the situation where one keystone species’ loss has rippling effects at other trophic levels. Some of the best-studied trophic cascades involve apex predators and their role in suppressing prey populations (Estes et al., 2011), but disease pathogens can also be a keystone species that leads to trophic cascades. For example, the introduction of rinderpest from Asia to Africa in the late 1800s caused catastrophic ungulate population declines in East Africa through the early 1900s. With no primary consumers, grasslands were encroached by woody plants; these changes in the primary producer community also increased the intensity and frequency of wildfires, leading to cascading impacts throughout these savannah communities. An extensive vaccination programme finally saw the disease eradicated in the 1960s, allowing ungulate population and grasslands to recover; and wildfires to become less destructive (Holdo et al., 2009).
The loss of keystone species from an ecosystem may create an extinction cascade—a series of linked extinction events following one another.
4.2.2 Maintaining ecosystem productivity
Plants and algae—in this context known as primary producers—use photosynthesis to capture and store energy from sunlight in their living tissue. This ability of ecosystems to generate living biomass, starting with plants trapping the sun’s energy, is known as ecosystem productivity. Primary consumers (i.e. herbivores) can then harvest this captured energy by eating plant material. The energy (and nutrition) obtained from plants enable herbivores to generate their own living biomass, in the form of growth and reproduction, before they, themselves, are consumed by secondary consumers (e.g. carnivores, predators, omnivores). This cycle ends (or starts, depending on one’s perspective) when decomposers and detritivores (e.g. fungi, earthworms, and millipedes) that break down complex plant and animal tissues into simple compounds such as nitrates, and phosphates. These simple compounds are then released into the soil and water, from where primary producers can take them up again.
4.2.3 Climate regulation
Many of us were taught from a young age that plants are the “lungs of the planet” (Figure 4.3) because they convert carbon dioxide (CO2) into breathable oxygen (O2) during photosynthesis. This contribution, whereby plants regulate the atmosphere’s CO2/O2 balance through carbon absorption and storage (termed carbon sequestration) forms part of the atmospheric carbon cycle and plays a major role in regulating global climate patterns. The reduction in plant life through deforestation or other human activities is thus of major concern because of the reduced capacity of plants to sequester atmospheric carbon dioxide, a greenhouse gas that contributes to climate change (Chapter 6). The important role of plant communities in the atmospheric carbon cycle is now even being recognised by global markets. For example, the carbon-storing capacity of the Congo Basin’s forests has an estimated value at over US $2.5 billion per year (Hughes, 2011). As part of the worldwide effort to reduce carbon dioxide emissions and address climate change, industrial countries and corporations have started paying some landowners to preserve and restore ecosystems that store significant amounts of carbon (Section 10.4).
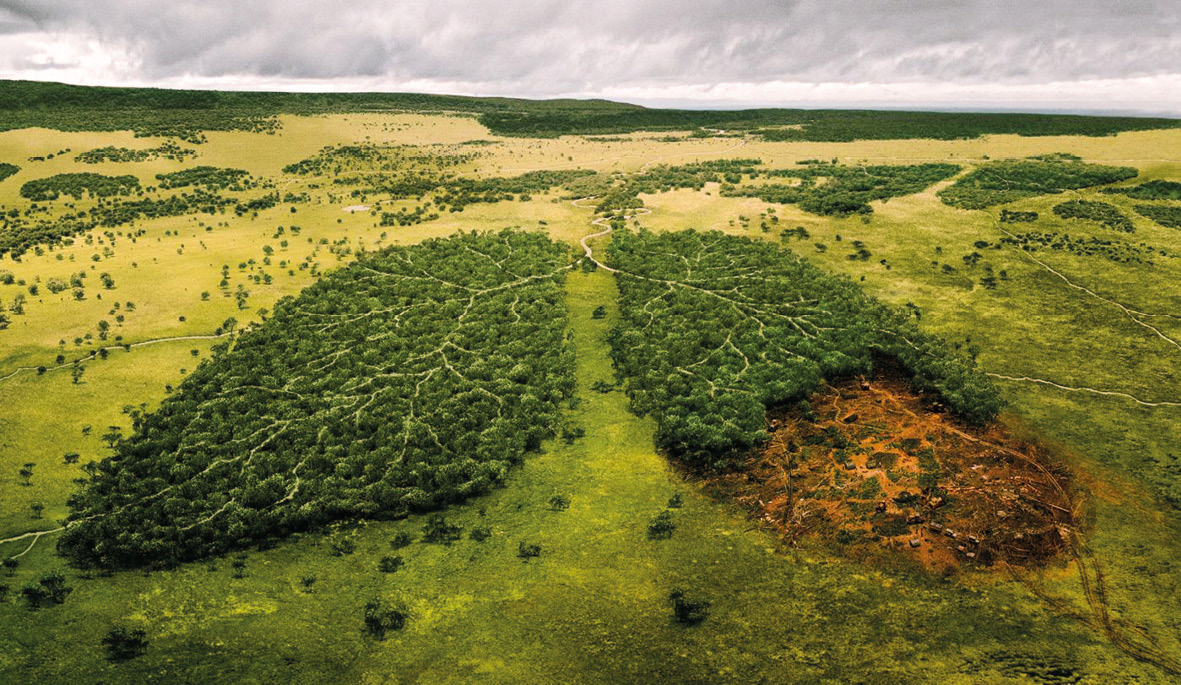
Figure 4.3 A very visual advertisement campaign used emotion and guilt to raise awareness of deforestation. It shows a forest as a pair of lungs, rivers symbolising veins and arteries, and water representing blood. The left lung is healthy, but the right one is partially cut down, symbolic of a cancer, to remind us that ongoing deforestation will increase our own personal discomfort. Image by TBWA\Paris, CC BY 4.0.
Plants are also important in regulating regional climate conditions by influencing both the water cycle via transpiration, and local heating and cooling via solar radiation absorption. For example, forests and other vegetation often absorb more heat than bare soil due to their respective albedos. Because heat rises, heat absorbed by vegetation enables water vapor released by plants via transpiration to rise higher into the atmosphere, where it subsequently condenses and falls as rain. In contrast, the loss of vegetation is often associated with reduced rainfall (Garcia-Carreras and Parker, 2011), which can in turn reduce agricultural productivity and biodiversity (Lawrence and Vandecar, 2015).
Lastly, trees keep local areas cool by providing shade and releasing water vapor into the atmosphere (Morakinyo et al., 2013; Kardan et al., 2015). This cooling effect increases people’s comfort and work efficiency, and reduces the need for fans or air conditioners, leading to higher productivity and cost savings (Balogun et al., 2014; Ogueke et al., 2017). Trees also act as windbreaks, thereby reducing evaporation and erosion in agricultural areas, and reducing the loss of heat from homes and other buildings in cold weather. The value of shade trees is also recognised in agro-ecosystems, as a strategy for coffee and cacao farmers to increase crop yields (Section 14.1.1) and to adapt to increasing temperatures due to climate change (Jaramillo et al., 2011).
4.2.4 Conserving soil and water quality
Wetlands play a prominent role in flood control. They are also very effective in immobilising pathogens and toxic pollutants released into the environment by human activities.
Wetlands play a prominent role in regulating soil and water quality, as well as flood control. During heavy rains, wetlands slow the speed of rushing floodwater, which lowers flood height and reduces erosion. Wetlands also act as natural sponges: they absorb vast amounts of floodwater during heavy rains, which is then released more slowly and evenly afterwards, thereby maintaining water sources used for drinking, irrigation, hydropower generation, and transport. Wetlands are also very effective in breaking down and immobilising pathogens, toxic pollutants, and excess nutrients released into the environment from agricultural activities, sewage, industrial wastes, and pesticides. One study from South Africa found that wetlands were almost 100% effective in preventing further spread of highly toxic organophosphorus pesticides (Schulz and Peall, 2001).
Wetlands are, however, not the only ecosystem that maintain soil and water quality and quality. In fact, maintaining complex and adaptive ecological communities of all kinds are of vital importance in buffering ecosystems against flooding and drought, protecting fertile soils, and maintaining water quality (see also Section 10.2.1). In intact ecosystems, plant foliage and dead leaves intercept rain, which slows the flow of water from upper reaches of catchment areas into streams and rivers; this allows for a slow release of water for days or even weeks after rains have ceased. Soil is anchored in place by plant roots and aerated by soil organisms; this combination increases the soil’s capacity to absorb water and hold nutrients. All these aspects together reduce flooding and limit erosion of fertile topsoil which, in turn, limits loss of essential nutrients that would otherwise occur after heavy rains.
The economic benefits of water quality maintenance services provided by intact plant communities are enormous. In the late 1980s, the New York City administration paid US $1.5 billion to local authorities in rural New York State to protect their water supplies by maintaining forests in the catchment area that surrounded the city’s reservoirs, and by improving agricultural practices in the catchment area. While US $1.5 billion may seem like a lot of money, at the time it was considered a pittance compared to the US $9 billion that the man-made water filtration systems—doing the same job—would have cost over just the first 10 years in operation (NRC, 2000).
A situation very similar to the one in New York is currently playing out in Kenya. The Mau Forest Complex is one of East Africa’s largest montane forests and serves as the principle catchment area for waters that flow into the famed Mara River and Lake Victoria. But large-scale deforestation in the Mau Forest Complex over the past few decades (Figure 4.4) has resulted in reduced water storage, flow regulation, groundwater discharge, and water purification, causing annual economic losses of over US $65 billion to Kenya’s energy, tourism, and agricultural sectors (UNEP, 2012). The situation in Kenya was so severe that the 2008 inauguration of a hydropower station was postponed due to low water levels; this station later achieved only 50% of its production capacity as a result of deforestation in the Mau Complex. To avoid further losses, the Kenyan government initiated a multi-stakeholder taskforce to investigate options to restore the Mau complex’s degraded forests (Prime Minister’s Task Force, 2009). Since then, tens of thousands of trees have been planted to reverse deforestation in the area.
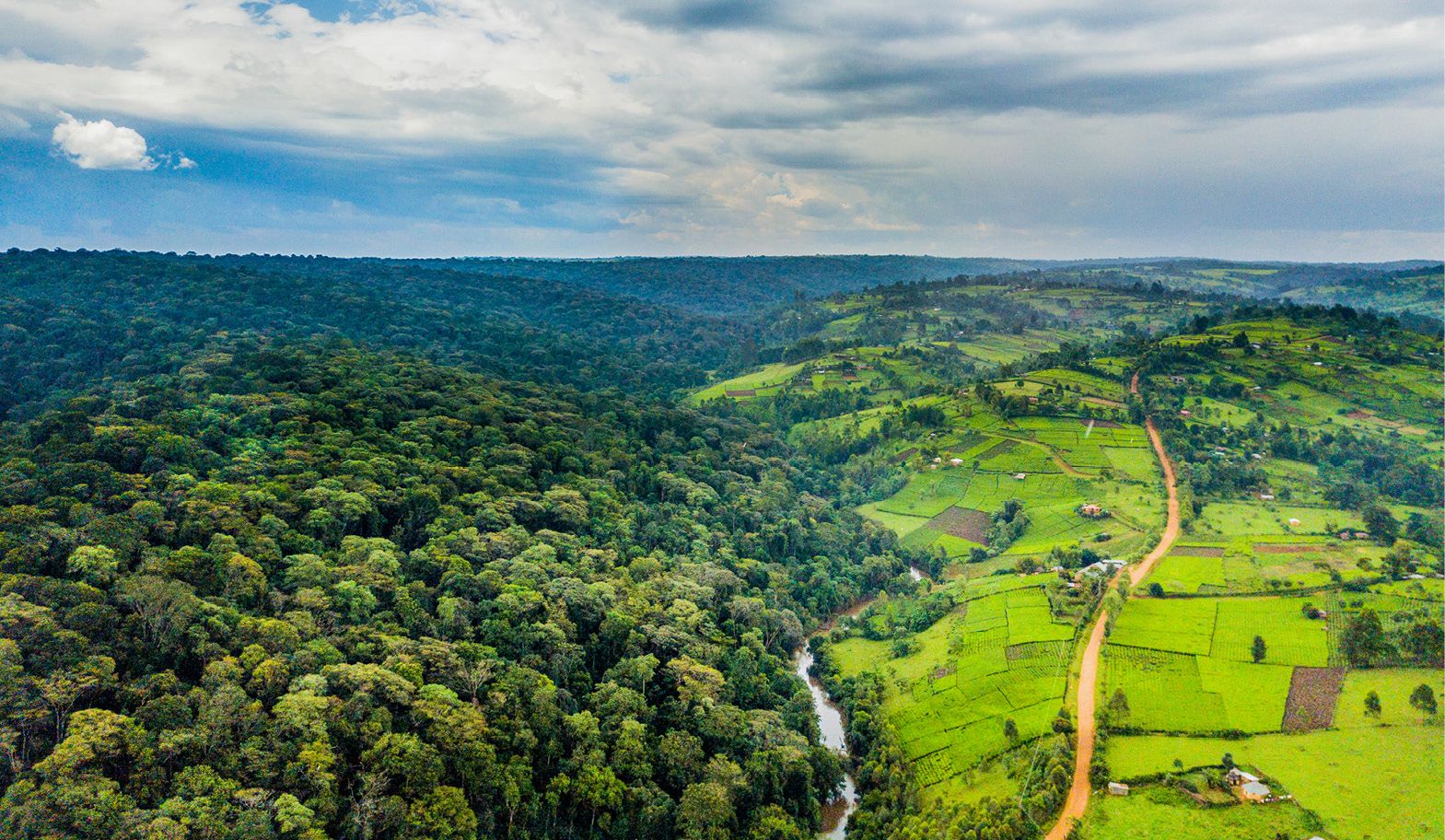
Figure 4.4 Logging, fire, and agriculture reduced the Mau Forest Complex, Kenya’s most important catchment, to a quarter of its original size, in the process damaging the region’s hydroelectric, tea, and tourism industries. Restoration plans are currently underway to reverse the destruction through extensive reforestation projects. Photograph by Patrick Shepherd/CIFOR, https://www.flickr.com/photos/cifor/36978973483, CC BY 4.0.
4.2.5 Pollination and seed dispersal
Pollination describes the transfer of pollen grains from male parts of a flower to female parts to allow fertilization and production of offspring. Some plants can be pollinated by wind, but others require animals to pollinate their flowers; examples include birds, bats, bees, flies, butterflies, and other insects (Figure 4.5). These pollination services are important for the persistence of many wild plants, as well as for many fruit, seed, and vegetable crops that we utilise as food (Box 4.2). Research from The Gambia has shown that management practices that increase the abundance of bats and bees to contribute to increased yields and sweetness of African locust bean (Parkia biglobosa) crops (Lassen et al., 2012). In contrast, work done in Zambia, Mozambique, and Uganda showed that pollinator collapse could increase malnutrition rates by over 50% which, in turn, could increase death rates among children and mothers during childbirth (Ellis et al., 2015). Luckily, many agricultural systems in Africa are still friendly to pollinators (see Box 7.4). Given the dependency on animal-assisted pollination in many agricultural systems, it is critical to maintain or expand pollinator-friendly practices. Our ability to continue benefitting from these services will depend on our ability to maintain and expand on those pollinator-friendly activities.
Box 4.2 Are Wild Pollinators Important in African Agriculture?
Acadia National Park, US National Park Service,
Bar Harbor, ME, USA.
Pollinators and food security are so closely tied to one another they should almost be considered synonymous terms. But when people think of pollination, they often only think of honeybees, which people domesticated more than 8,500 years ago for honey production. However, wild pollinators, which include a variety of insects, birds, and mammals, are often more effective at pollinating than honeybees are. One estimate suggests wild pollinators can double fruit production compared to honeybees (Garibaldi et al., 2013). This is most likely because the morphological and behavioural diversity of wild pollinators allow for more specialised pollination relationships with plants. For example, some wild pollinators have longer proboscis (i.e. insect tongues) that enable them to pollinate deeper flowers (Figure 4.A), something honeybees cannot do. African crops rely even more on wild pollinators than do crops in other areas of the world because it can be difficult to maintain aggressive African honeybee hives and prevent them from being damaged by wild animals (African Pollinators Initiative, 2007).
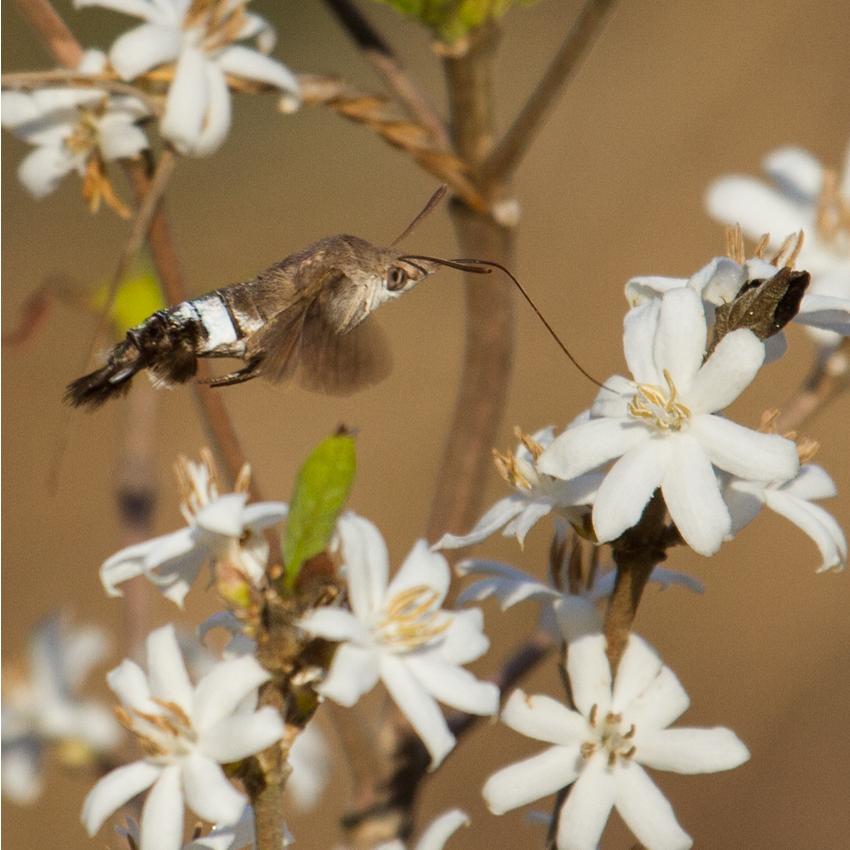
Figure 4.A With their long proboscis, wild pollinators, such as this white barred hawk moth (Leucostrophus alterhirundo) from Mozambique, are highly efficient pollinators. Photograph by Celesta von Chamier, https://www.inaturalist.org/observations/1124702, CC BY 4.0.
Eggplant, papaya, coffee, and palm oil—crops of huge economic and cultural importance—highlight the value of wild pollinators to local and global economies. Eggplants are hermaphroditic; in other words, they can self-pollinate. Even so, pollination from two wild bee species, namely the doubleband carpenter bee (Xylocopa caffra) and a type of sweat bee (Lipotriches rufipes), increase fruit production far beyond that of self-pollination (Gemmil-Herren and Ochieng, 2008). In contrast, papaya trees are dioecious (i.e. they have separate male and female trees) and thus depend on crosspollination (i.e. pollinators take pollen grains from male flowers on one tree to female flowers on another tree) to produce fruit. While a wide variety of wild bees and butterflies visit papaya flowers, only some hawkmoths and skipper butterflies are effective papaya pollinators, probably because they have long proboscises that can penetrate the deep papaya flowers (African Pollinators Initiative, 2007). A healthy and diverse pollinator community also help coffee plants (which relies on a variety of pollinators, Samnegård et al., 2014) and oil palm (which requires cross pollination by specialist oil palm weevils, African Pollinators Initiative, 2007) produce more fruits, thereby increasing their economic value.
Despite their value to natural ecosystems and food security, wild pollinator populations are declining worldwide (Gallai et al., 2008; Dirzo et al., 2014). To avoid losing them forever, it is important to preserve wild pollinators through the conservation and restoration of native ecosystems (Chapter 10), sustainable agricultural practices, such as the reduced use of pesticides and herbicides (Section 14.1.1), and by communicating the value of pollinators to the general public, land managers, and politicians. Additionally, monitoring and research programmes aimed at pollinators could enhance our understanding of their value, ecology, and conservation.
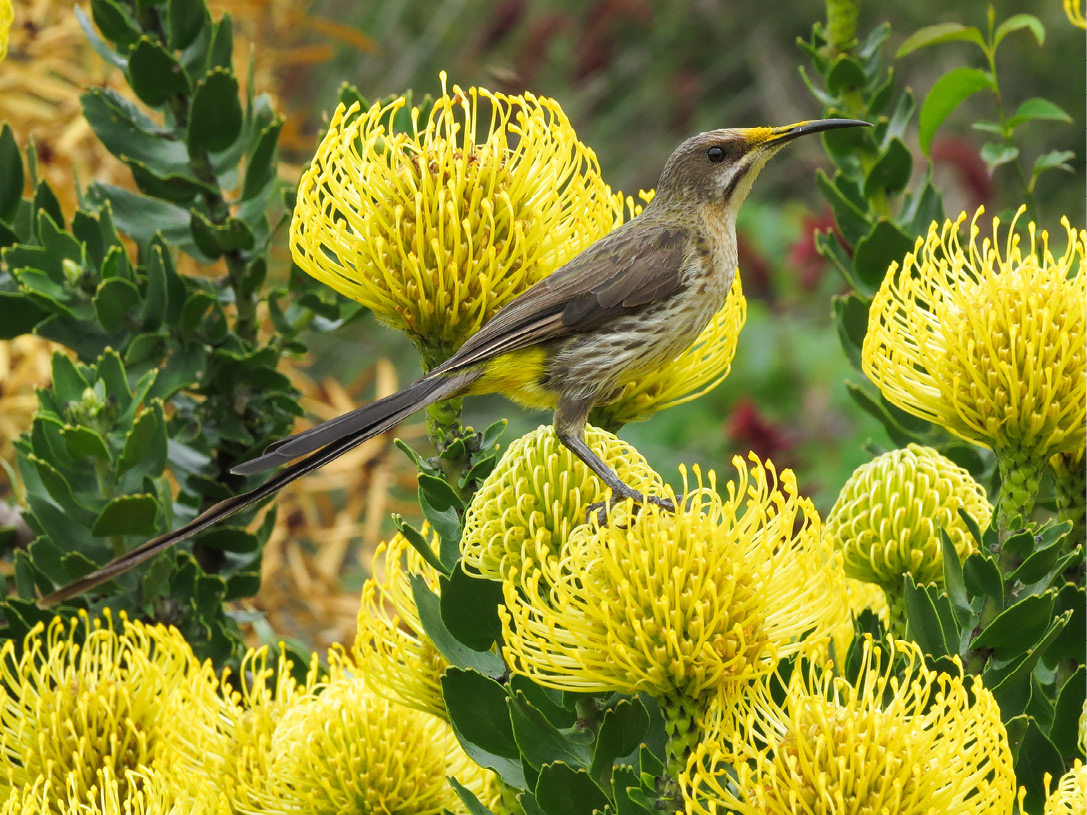
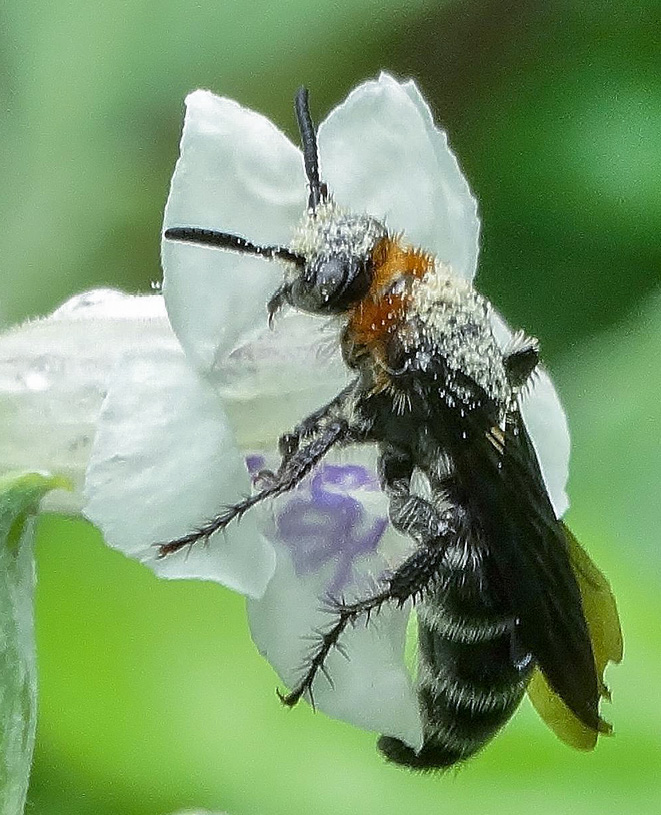
Figure 4.5 (Top) A Cape sugarbird (Promerops cafer, LC) feeding on a pincushion (Leucospermum sp.), and in the process pollinating the plant. Photograph by Rafael Tosi, https://macaulaylibrary.org/asset/118353841, CC BY 4.0. (Bottom) A scoliid wasp (Scoliidae) pollinating a creeping foxglove (Asystasia gangetica) flower. Photograph by Peter Vos, https://www.inaturalist.org/observations/10965989, CC BY 4.0.
Many fruit and seed-bearing plants also depend on a process called seed dispersal to reproduce, colonise vacant habitats, and avoid competing with parent plants for limiting resources. Seed dispersal describes the physical movement of seeds by fruit-eating and seed-eating birds, large herbivores, primates, and a range of other animals away from the parent plant. Due to specialised features, some seeds can stick to animals’ fur, allowing them to be carried along much further distances than wind could, and different directions than water could. Many animals also consume seeds and fruits, providing opportunities for dispersal when the consumer moves off looking for more food, a resting spot, or mates to interact with. For some plants, seed dispersal involves a critical step required for germination, namely seed scarification. One method of scarification involves an animal breaking the seed’s hard coat by biting it. Alternatively, stomach acids may weaken the consumed seed’s hard coat while it passes through the animal’s digestive tract. Without this step, seeds requiring scarification may not be able to germinate; those plants’ persistence thus depends upon the animals that consume them. While the importance of pollination for food security is well known, the importance of seed dispersal should not be underestimated. A study from Côte d’Ivoire found that primates provided necessary seed dispersal services for at least 25 fruiting plant species important to humans (Koné et al., 2008).
4.2.6 Hazard detection and mitigation
When intact, nature is our first line of defence against many natural disasters. Consider, for example, the contribution of mangrove swamps in protecting us from cyclones/hurricanes (van Bochove et al., 2014), or the contribution of wetlands in flood control (Section 5.3.3). In contrast, degrading the natural environment can have severe consequences. For example, a 2010 landslide in Uganda that buried three villages, killing over 300 people and displacing 8,000 more, was attributed to deforestation activities three years earlier (Gorokhovich et al., 2013). To prevent such disasters, and harness all the other contributions of forests, there are numerous projects across Africa working to reverse deforestation (Section 10.3). Unfortunately, Africa’s tropical forests regenerate very slowly—sometimes requiring more than 100 years (Bonnell et al., 2011). It is thus critical to prevent ecosystem degradation in the first place, rather than having to resort to costly restoration projects.
In addition to keeping us safe, biodiversity can also be used to help track environmental changes. Species used for this purpose, called indicator species or environmental monitors are, by definition, associated with unique environmental conditions or sets of ecosystem processes. Tracking changes in their population sizes, distributions, and behaviour of can thus serve as a substitute for expensive detection equipment (Section 10.1). Aquatic filter feeders, such as mussels and clams are particularly useful in this regard because their tissues accumulate chemical pollutants. A study from Senegal’s mangrove swamps detected heavy metal pollution using clams, mussels, and snails after tests barely detected those pollutants in the area’s sediments (Bodin et al., 2013). But even common everyday species can serve as indicator species: for example, conservation authorities around the world are using bird abundances and behaviours to better understand the impact of climate change (http://climatechange.birdlife.org).
Sentinel species are a special type of indicator species that can act as an early warning system for environmental hazards because they are more sensitive to certain conditions than humans are. Lichens are particularly well-known sentinel species. Being sensitive to air pollution and chemicals in rainwater, some lichens cannot survive in polluted areas. Thus, their presence is generally a sign of good air quality, while their absence may signal air pollution (Bako et al., 2008). Another example is seabirds, whose declining populations can serve as an early-warning system for overfishing (Paiva et al., 2015). Some sentinel species can even be used directly for human health purposes. For example, the non-profit NGO APOPO has been taking advantage of the incredibly fine sense of smell of southern giant pouched rats (Cricetomys ansorgei LC)—affectionately called HeroRATs—to detect landmines (Figure 4.6), tuberculosis (Reither et al., 2015), salmonella infections (Mahoney et al., 2014), and even people trapped under collapsed structures (LaLonde et al., 2015).
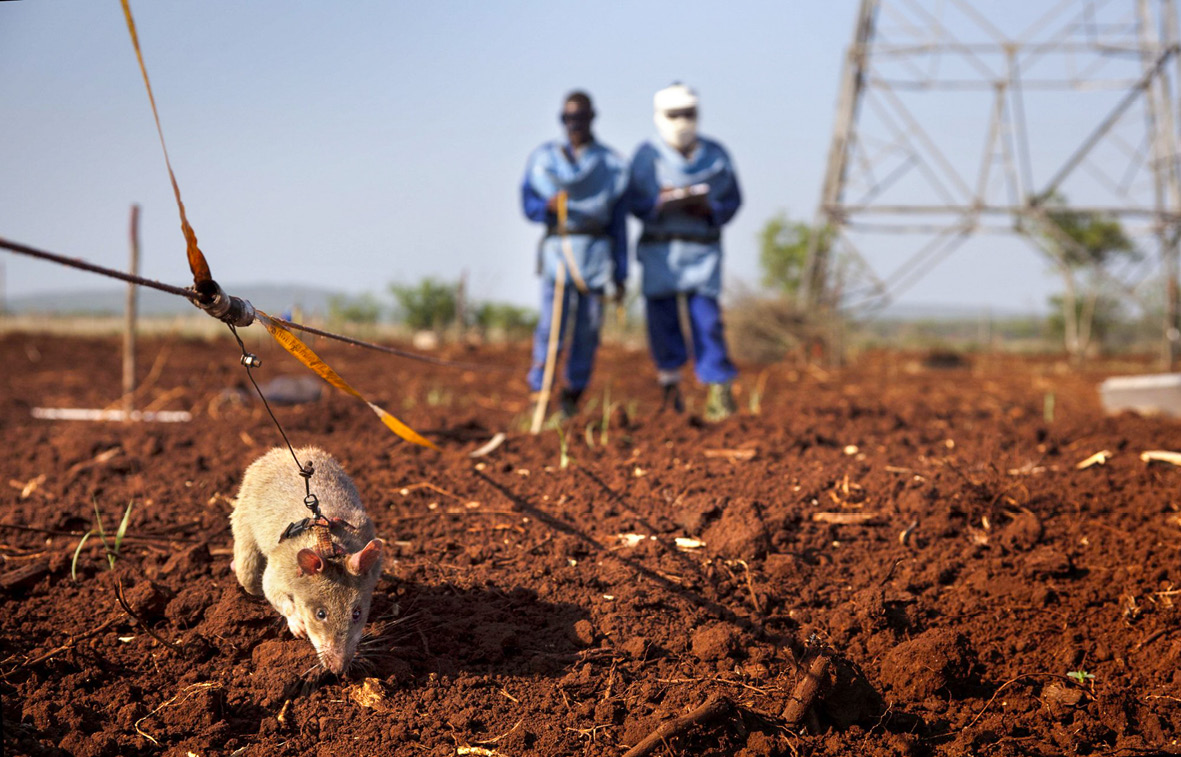
Figure 4.6 With the help of specially trained southern giant pouched rats, over 80,000 landmines and other unexploded remnants of war were found and destroyed in Mozambique starting in 2008; the country was declared landmine free in 2015. Photograph by APOPO, CC BY 4.0.
Lastly, some species can be used to mitigate various sources of pollution. For example, through a process called biosorption, the superior absorption capabilities of some lichens, plants, fungi, and microorganisms offer some of the cheapest and most effective methods for removing toxic heavy metals (Fosso-Kankeu and Mulaba-Bafubiandi, 2014) from the environment. Scientists also recently discovered a plastic-eating fungus (Khan et al., 2017) that may provide a potential solution to plastic pollution.
4.2.7 Pest and disease control
Every day, predators, such as owls and bats, keep us healthy by controlling populations of disease vectors, such as rats and mosquitoes. This process, where predatory (and parasitic) species regulate populations of pests and other nuisance species, is known as biological control, or biocontrol in short (Box 4.3). The use of insectivores (i.e. insect-eating species), such as bats and birds, to control crop pests is well established in traditional farming systems (Abate et al., 2000). But even on commercial crop farms, natural enemies, such as bats and birds, play an important role in keeping pests under control (Taylor et al., 2018). Some plants also play a part in biocontrol efforts: recent research found that some native plants used for intercropping in traditional agricultural systems emit chemical signals that kill and drive pest species away from crops (Khan et al., 2010). With an increasing number of studies illustrating the significant benefits gained from natural pest control systems, enhanced farming practices that facilitate greater ecosystem complexity (Section 14.1.1) will hopefully play a bigger role in food security in future.
Box 4.3 Biological Control Saves the Cassava Crop
Biology Department,
Boston University,
Boston, MA, USA.
As it stands along the farm-plot boundary,
its base appears beautiful like a bride’s feet…
O cassava to whom the bembe drum beats a salute
that never reaches an end…
It is no small service the cassava renders us in this our land
Yoruba Poem (Babalola, 1966)
This traditional song from Nigeria praises the cassava, a South American crop brought to tropical Africa in the 16th century, and upon which millions of Africans have since relied for food and income.
Disaster struck in the 1970s, when an agricultural scientist that brought a new variety of cassava from South America to Africa also accidentally introduced a new pest: the cassava mealybug (Phenacoccus manihoti) (Neuenschwander, 2001). Previously unknown to science, the bug attacked the new shoots of cassava plants, laying its eggs at their tips and stripping them of their leaves. As it spread through Central and West Africa, the mealybug wiped out 80–90% of the productivity of most cassava fields, threatening large parts of Africa with famine.
With so many Africans relying on the cassava as a primary food source, scientists had to find a solution, and quickly. The bug’s waxy coating that protected it from pesticides complicated this effort. With conventional pest-control methods failing, scientists turned to biological control, hoping that introducing a natural predator would counteract the spread of the invasive insect. Researchers searching for the source of the mealybug finally found a candidate in the fields of Paraguay, where cassava, known locally as mandioca, was also an important food staple. Here, investigators discovered that mealybug numbers were kept low by a tiny wasp called Anagyrus lopezi that attack the mealybugs’ eggs and larvae (Figure 4.B). A. lopezi passed laboratory tests for host specificity—it fed and bred exclusively on cassava mealybugs and would not attack other African insects. And so, the International Institute of Tropical Agriculture (IITA) began field tests using the wasp as a biological control agent.
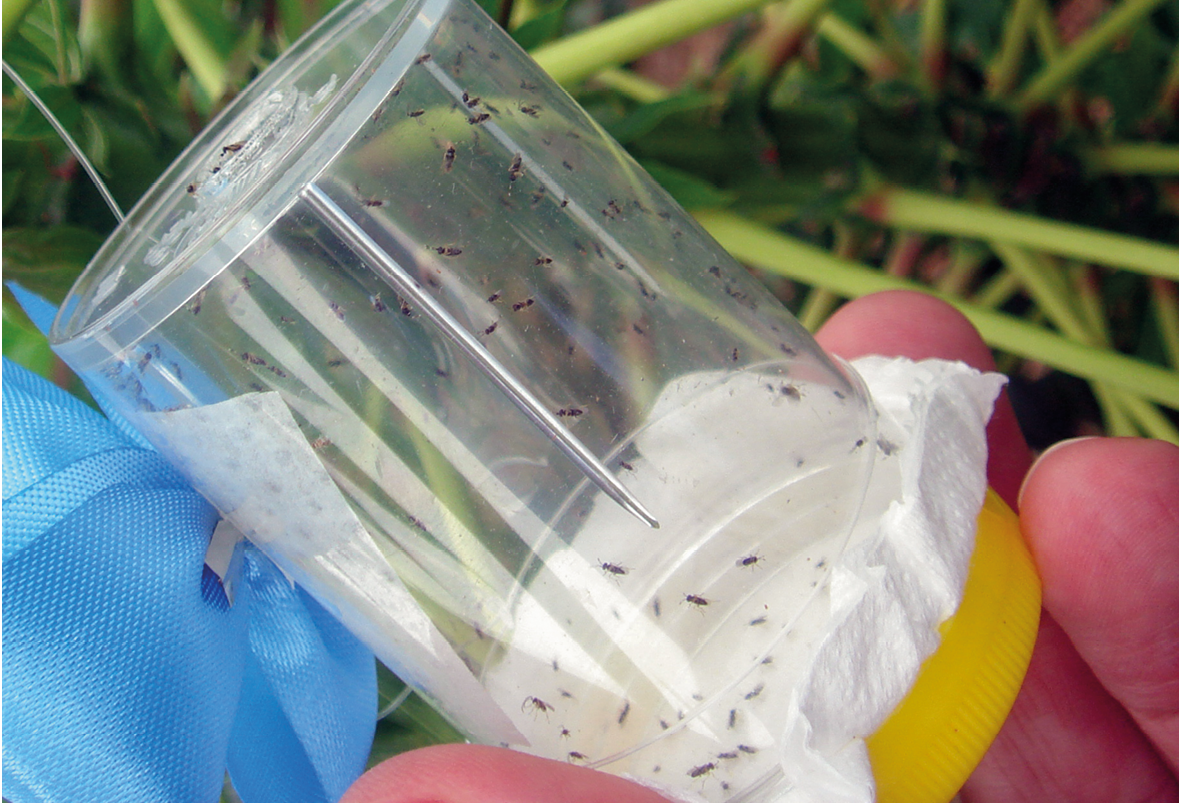
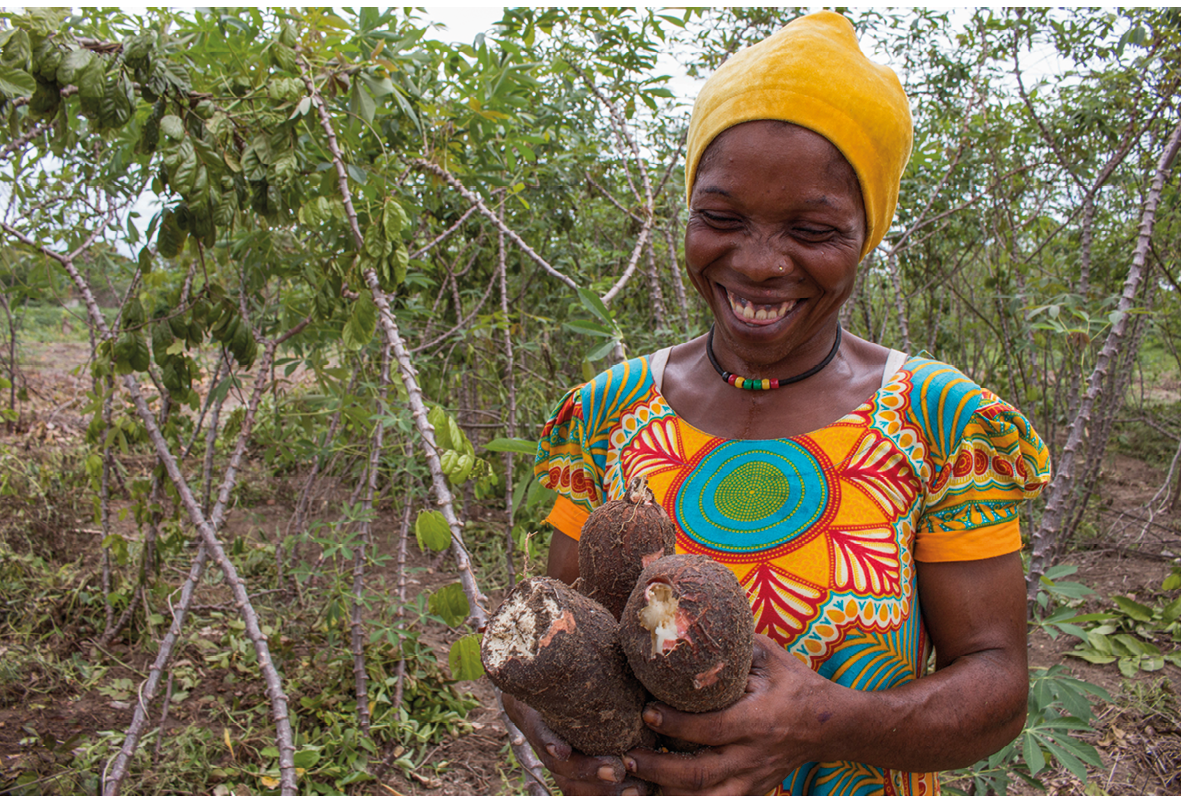
Figure 4.B (Top) A vial containing the parasitic wasp Anagyrus lopezi at a biocontrol release site. Photograph by Rod Lefroy/CIAT, https://www.flickr.com/photos/ciat/4809242082, CC BY 2.0. (Bottom) A cassava farmer from Tanzania smiles broadly, very happy with her crop. Photograph by Holly Holmes/CGIAR RTB, https://www.flickr.com/photos/129099219@N03/33324350781, CC BY 2.0.
Results were astounding; the quick-spreading Paraguayan wasp reduced crop losses by an impressive 95% (Neuenschwander, 2001), all without the danger of pollution and poisoning associated with traditional pesticides. While identifying and introducing the biocontrol agent required significant resources, estimates suggest gains of 370–740 times the original investment, depending on the region considered (Zeddies et al., 2009), making it well worth the cost. Today, A. lopezi is found everywhere where the cassava mealybug survives in Africa. Bolstered by this success, the IITA has subsequently expanded its biological control programmes to fight tropical pests on crops, such as cowpeas, maize, and bananas.
In 2008, the cassava mealybug was discovered in Southeast Asia, where it repeated the damage inflicted in Africa (Graziosi et al., 2016). Scientists are now replicating Africa’s biocontrol efforts to reduce crop failure in Vietnam, Thailand, Cambodia, and China. In conjunction with a number of local parasites, they hope that A. lopezi will halt the spread of the mealybug before it reaches even larger fields in India (Parsa et al., 2012). The control of the cassava mealybug is certainly one case where biological control was able to achieve great success.
Most Africans are familiar with scavengers, such as jackals and vultures, that work as nature’s clean-up crew, picking at food scraps left in the field by large predators. Together with the range of flesh-eating insects, detritivores, and decomposers, scavengers play a crucial role in keeping us healthy by sanitising the environment (O’Bryan et al., 2018). While it is all too easy to take these activities for granted, some people actively welcome these services. For example, in northern Ethiopia, spotted hyenas (Crocuta crocuta, LC) are tolerated in urban settlements because they consume livestock carcasses and sometimes even human corpses, which pose a disease risk (Yirga et al., 2015).
Recent experiences have shown that without proper care, the sanitary services provided by wildlife can collapse over a very short time. For example, during what is known as the Asian vulture crisis of the 1990s, vulture populations in India, Pakistan, and Nepal declined precipitously in a matter of years from secondary poisoning after eating carcasses of dead animals treated with the anti-inflammatory drug diclofenac. With nothing else available to remove carcasses of dead animals as efficiently as vultures, rotting flesh contaminated drinking water and allowed populations of rats and feral dogs (Canis familiaris) to proliferate. While vultures have stomach acids which kill pathogens, dogs and rats do not and thus became major pathogen vectors, spreading deadly diseases such as rabies, anthrax, and plague. The estimated healthcare costs in the face of Asia’s vulture crisis amounted to over US $1 billion per year (Markandya et al., 2008). Today, Africa is facing its own vulture crisis. But instead of one threat, Africa’s vultures face a multitude of human-made threats, making solving this crisis much more complex (Box 4.4).
Scavengers such as vultures and jackals are nature’s clean-up crew; they keep us healthy by sanitising the environment.
Box 4.4 Conservation Lessons from the Asian and African Vulture Crises
Department of Biological Sciences, University of Eswatini,
Kwaluseni, Eswatini.
ara@uniswa.sz
A common perception among laypeople and conservationists alike is the idea of safety in numbers for wildlife species. After all, is a widely distributed and abundant species not safe from the threats of extinction? The answer is a firm no! As the collapse of central Asia’s vulture populations (Oaks et al., 2004) demonstrates, species numbering in the millions can disappear in the space of just a few years.
The Asian vulture crisis shares some similarities with the demise of the passenger pigeon (Ectopistes migratorius, EX) in North America a century ago. This pigeon was once the most abundant bird on Earth; yet, despite numbering in the billions, it was driven to extinction in a short span of time, primarily due to hunting over a 20-year period in the late 1800s. In the case of Asian vultures, the threat was not hunting, but rather a nonsteroidal anti-inflammatory drug (NSAID)—diclofenac—which is fed to sick cattle and then ingested by vultures when they feed on dead livestock. As diclofenac is deadly toxic to vultures, the widespread use of this treatment on the Indian subcontinent (which includes India, Nepal, and Pakistan) has seen catastrophic vulture population declines. With one of Asia’s major natural trash disposal systems gone, the area experienced a human health crisis from widespread drinking water contamination and increased incidence of diseases carried by ubiquitous and increasing rat and feral dog populations (Markandya et al., 2008).
The Asian vulture crisis is instructive on several grounds. First, it took a long time to detect and confirm the vulture declines because regular and standardised monitoring of the three affected vulture species had not been conducted. Second, the extent of the decline was extreme, with vulture numbers declining by over 95% within a decade. Third, the declines were due to a single threat—contamination from diclofenac, which were subsequently found to be deadly-poisonous to vultures (Oaks et al., 2004). Thanks to the concerted efforts of conservationists and politicians, and the rapid reactions of the governments of India, Pakistan, and Nepal, diclofenac was removed from the market in 2006. Vulture populations in Asia have since stabilised, with even a cautious suggestion of an increase.
Now, Africa faces its own vulture crisis (Ogada et al., 2015). However, in contrast to the Asian crisis, Africa’s crisis involves a greater number of species, and spans a larger geographical area. Importantly, it also includes a greater number of threats, including poisoning, harvesting for traditional medicine and for food, and electrocution following contact with power lines. Many vultures also die when they scavenge on poisoned carcasses meant to kill problem predators (Figure 4.C). To this list of lethal causes, one should also add the universal threats of habitat loss and persecution of birds of prey.
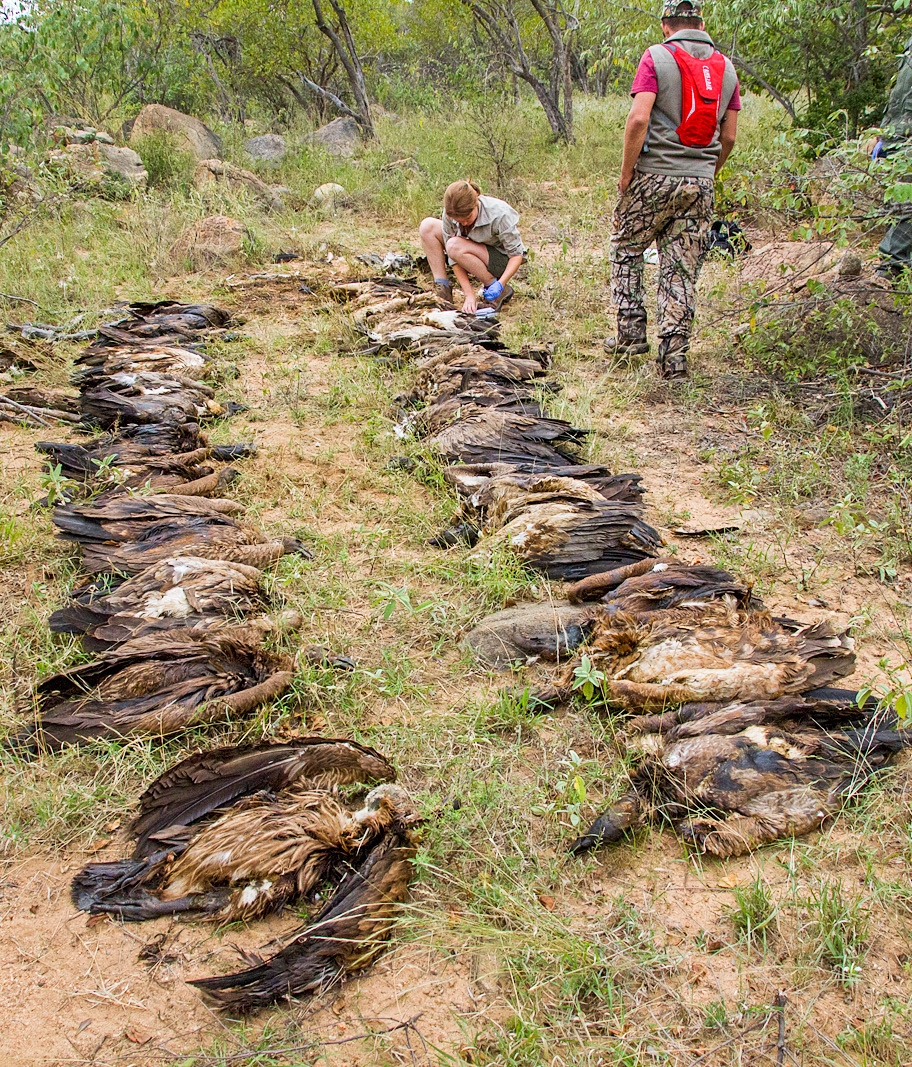
Figure 4.C Conservation biologists inspect several white-backed vultures that were poisoned at South Africa’s Kruger National Park. Poachers across Africa intentionally kill vultures for traditional medicine, and unintentionally kill them while setting traps for large predators. Photograph by Andre Botha, CC BY 4.0.
Thanks to long-term monitoring, the collapse of African vulture populations has been well documented. Of the 95 vulture populations being monitored, 89% were either extirpated or experienced severe declines. Across eight study species, the mean rate of decline is estimated at 4.6% per year (i.e. one out of 20 birds that are dying per year are not being replaced). The charismatic Rüppell’s vulture (Gyps rueppellii, CR) has declined by 85% across its range; consequently, this species is now considered highly threatened by the IUCN, as are the hooded vulture (Necrosyrtes monachus, CR), white-headed vulture (Trigonoceps occipitalis, CR), and African white-backed vulture (Gyps africanus, CR). Only slightly better off, at least for now, are the lappet-faced vulture (Torgos tracheliotos, EN) and Egyptian vulture (Neophron percnopterus, EN), the Cape vulture (Gyps coprotheres, VU), and the bearded vulture (Gypaetus barbatus, NT).
The collapse of Africa’s vulture populations is cause for serious concern among conservation biologists, wildlife and livestock managers, and public health officials. Unlike in Asia, however, workable solutions to Africa’s vulture crisis have not yet been found. This may be due to the multitude of threats, and the complexity of the problem exacerbated by the involvement of individual poachers, local communities, and government structures across more than 40 countries. If conservationists and governments can work together, as they did in Asia, then perhaps Africa’s vultures and the ecosystem services that they provide can still be saved.
4.3 Nonmaterial Contributions
Nonmaterial contributions from nature, also called cultural services, include the subjective and psychological aspects of nature that influence our perceptions about quality of life. These contributions can be divided into three subcategories: inspiration and learning support, supporting psychological and physical experiences, and supporting individual and group identities.
4.3.1 Inspiration and learning support
Nature has inspired artists and writers throughout history. Consequently, many books, television programmes, movies, and websites produced for entertainment purposes are based on natural themes. This infusion of nature into popular culture is worth billions of dollars per year. To take one example, the 1994 Disney blockbuster The Lion King, based on the lives of a variety of African savannah animals, generated revenues estimated at just under US $1 billion from theatre attendances alone. It was so successful that three movie sequels, an animated television series, and several video games and books followed. A musical based on The Lion King movie plot continues to be a top-earning title in box-office history for both stage productions and films.
Movies featuring stunning natural landscapes and charismatic wildlife often increase the desire of moviegoers to visit natural areas where they can see these landscapes and animals first-hand. But it can also raise awareness of environmental issues in new audiences. While many documentaries are created with this purpose in mind, such benefits can also extend to blockbuster movies meant for broader audiences (Silk et al., 2018). For example, Disney’s Happy Feet (2006) highlighted the threat of overfishing and plastic pollution to penguins; Avatar (2009) raised awareness of habitat loss and overharvesting; and The Jungle Book (2016) exposed audiences to the plight of pangolins. Such exposure can even lead to environmentally conscious behavioural changes. For example, moviegoers were willing to donate 50% more money to climate mitigation after watching the apocalyptic movie The Day After Tomorrow (2004) (Balmford et al., 2004). Perhaps, in part, due to the influence of environmentally-orientated movies, an increasing number of movie stars (and other celebrities) have started using their stardom as a platform from where they promote biodiversity conservation efforts in Africa (Duthie et al. 2017; see also https://wildfor.life).
Scientists and engineers often seek inspiration from nature for new technologies or to solve innovation challenges.
Scientists and engineers also sometimes turn to nature to seek inspiration for new technologies or to solve innovation challenges. For example, the water-vapor collecting capacity of the racing stripe darkling beetle (Stenocara gracilipes) from the Namib Desert in Namibia (Figure 4.7) inspired engineers who developed self-filling water bottles (Clark, 2012), irrigation systems to overcome drought conditions (Scott, 2011), fog-free windows and mirrors (Parker and Lawrence, 2011), and methods for controlling condensation and frost on aircraft surfaces (Boreyko et al., 2016). While these and other scientific endeavours, collectively known as biomimicry, provide many social and economic benefits, their primary value comes from new knowledge, improved education, and enriched human experiences.
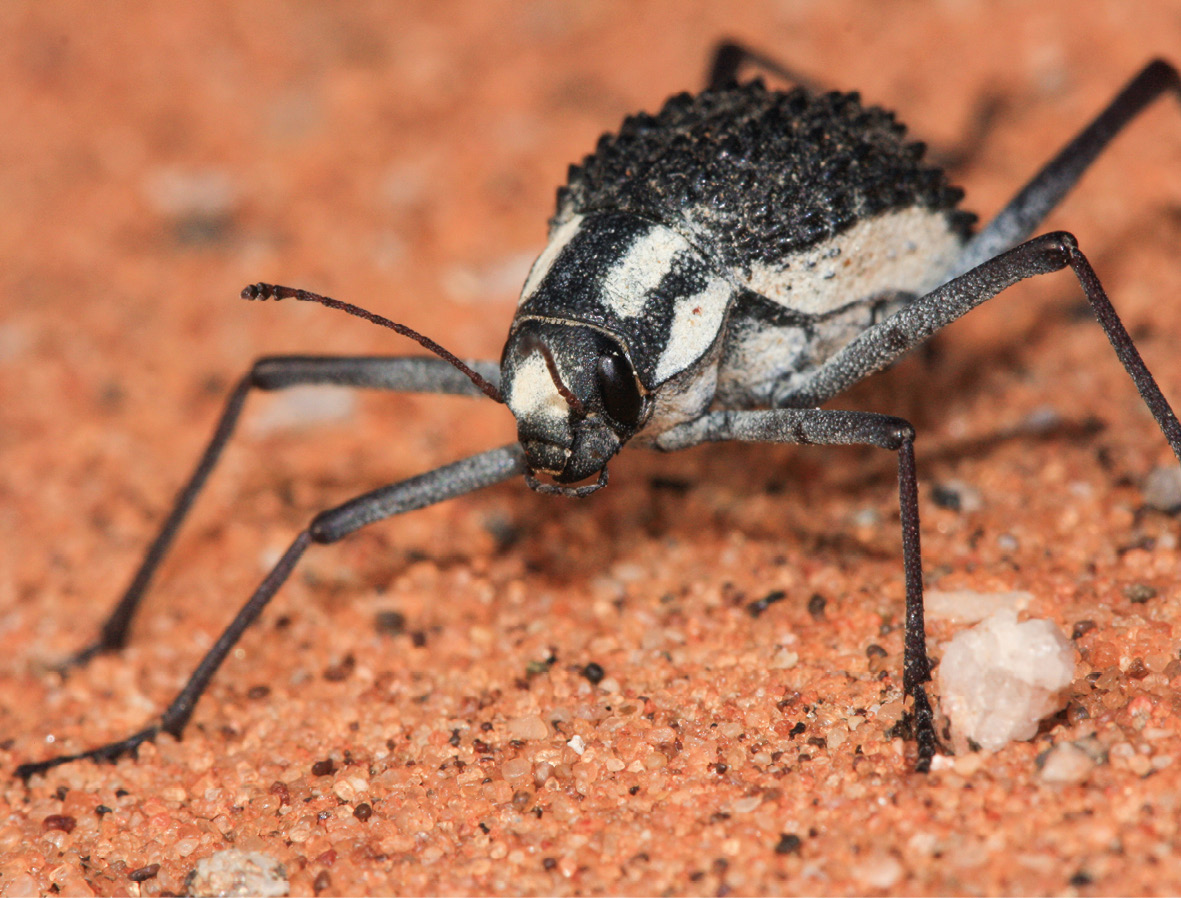
Figure 4.7 The racing stripe darkling beetle is endemic to one of the world’s most arid regions, Namibia’s Namib Desert. To survive, it collects water from early-morning fog with the bumps on its back. In a classic case of biomimicry, creative entrepreneurs are copying these features to create self-filling water bottles and fog-free windows. Photograph by Alex Rebelo, https://www.inaturalist.org/observations/11086737, CC BY 4.0.
4.3.2 Supporting psychological and physical experiences
While the economic benefits gained from nature incentivises biodiversity protection, many people believe that the aesthetic values of nature provide an even greater incentive for conservation. This principle rests on the fact that nearly everyone enjoys wildlife and landscapes aesthetically. Even city-dwellers who are superficially removed from nature find a sense of relief and well-being when they have opportunities to come in close contact with the natural world. But what if dragonflies and butterflies disappeared? What if our favourite sports team’s mascot ceased to exist in nature? What if there were no more forests filled with bird flocks or monkey troops?
The intangible but desirable aesthetic values people attach to certain aspects of nature are known as amenity values. Amenity values are becoming increasingly important in many local and national economies throughout Africa, in the form ecotourism. At any one time, there are millions of tourists traveling and spending money across Africa to see particular species or to experience unique ecosystems. This includes scuba divers approaching a coral reef (Figure 4.8), birdwatchers visiting a rare species’ stakeout, and people on a safari to view the many flagship species for which Africa’s savannahs are so well known. Ecotourism has long been a major industry in southern and East Africa. For example, ecotourism generated over US $1 billion in annual revenue in the Cape Floristic Region more than a decade ago (Turpie et al., 2003), and has accounted for over 15% of Kenya’s gross domestic product (GDP) at times (WWF and BSI, 2006). Ecotourism is also becoming increasingly important in other parts of Africa. For example, since overcoming periods of social unrest, Burundi, Rwanda, and Uganda have created profitable local industries charging tourists high fees to visit habituated populations of mountain gorillas (Gorilla beringei beringei, EN). Also, in South Africa, some bird guides earn an average of US $362 per month by showing tourists the unique birds their local area has to offer (Biggs et al., 2011).
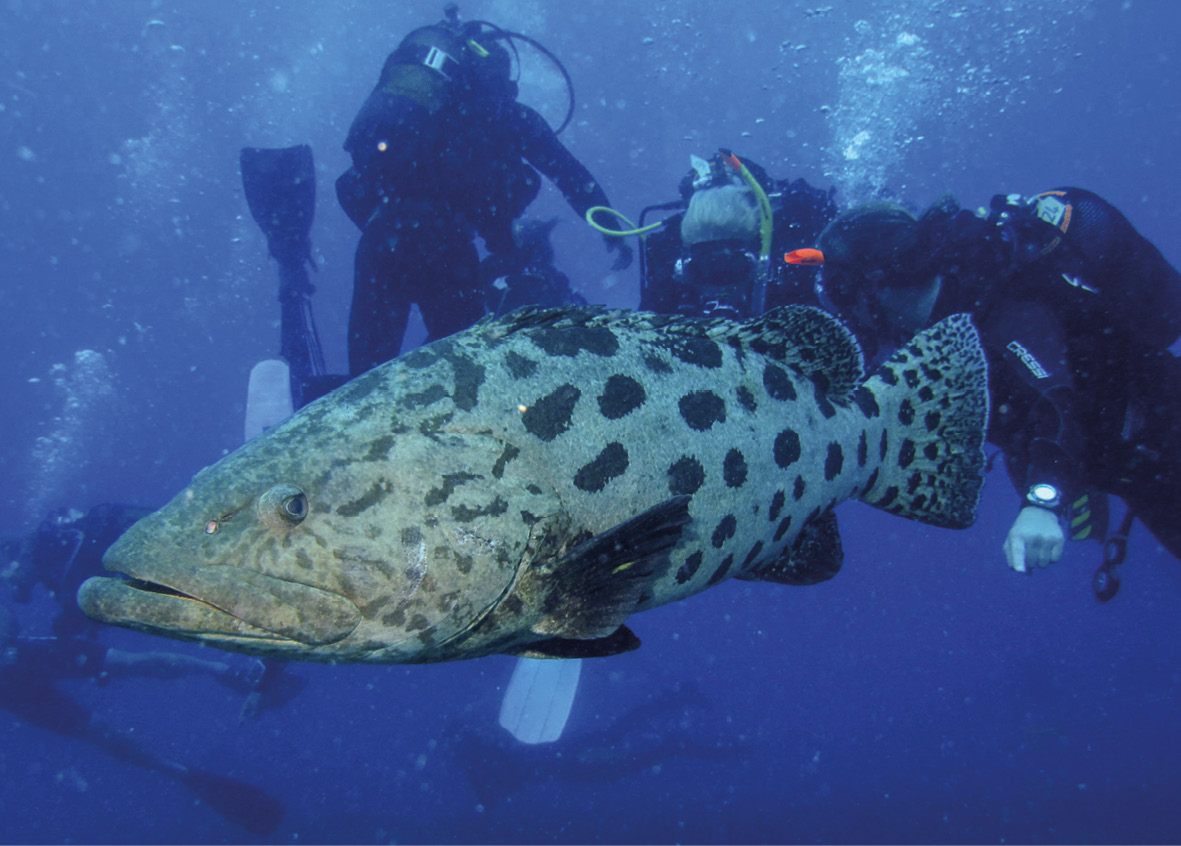
Figure 4.8 Scuba divers on vacation at Ponta do Ouro, Mozambique, appreciating a large potato bass (Epinephelus tukula, LC). The income to be gained from ecotourism activities often outweighs the profits from unsustainable harvesting, and thus provides a strong economic justification for biodiversity conservation. Photograph by Derek Keats, https://www.flickr.com/photos/dkeats/36684179721, CC BY 2.0.
In recent years, volunteer-based ecotourism has emerged as a lucrative industry that combines ecotourism with learning opportunities. These organisations offer aspiring conservationists and citizen scientists hands-on experience while bringing financial and other logistical support to rural and protected areas. Many wildlife sanctuaries and conservation NGOs also offer volunteer opportunities and field courses that combine conservation action with local community outreach and education programmes. The research done by professional scientists and citizen science volunteers can be used in locally-relevant educational materials. Biological field stations (Section 13.1.5) often host these activities; the stations can also provide training and jobs for local community members.
The revenue and jobs generated by ecotourism provides a strong and immediate justification to protect biodiversity and restore areas that have been degraded.
The revenue and jobs generated by ecotourism provides a strong and immediate justification to protect areas rich with biodiversity or to restore areas that have been degraded. Ecotourism can even be integrated directly in plans for future development, protection, and restoration. One such example is integrated conservation and development projects (ICDPs, Section 14.3), which provide models for how empowered rural communities can successfully establish accommodation, develop expertise in nature guiding, and sell local handicrafts at curio stores to obtain multiple stable income streams. The revenue obtained from ecotourism also allows local people to move away from unsustainable hunting, fishing, or grazing practices towards lifestyles that can be maintained in the long term.
Still, many of Africa’s ecotourism resources remain under-utilised. To use one example, only a few locations in Africa cater to people who enjoy the thrill of swimming with sharks in their natural habitat. Beyond removing fear and instilling a healthy respect for sharks, this industry also plays an important role in conservation by showing how living sharks bring greater economic benefit than a once-off catch. For example, shark diving at just one location in South Africa is estimated at US $4.4 million annually (Hara et al., 2003); similar industries in the Maldives (Cagua et al., 2014) and Palau (Vianna et al., 2012) generate even more revenue. Presenting unique recreational experiences and a growing global ecotourism sector, more and more African countries will hopefully explore these and other opportunities soon. It is worth noting that the long-term effects of shark diving operations are largely unknown, particularly as it relates to possible behavioural changes from using bait to attract sharks to people, and an active area of current research (Gallagher and Huveneers, 2018).
Although ecotourism can provide many valuable conservation and economic benefits (Thiel et al., 2014), care must always be taken that these activities abide by accepted ethical standards (Hayward et al., 2012). It is also worth remembering that wildlife ecotourism is often geared towards wealthy western markets, making it prohibitively costly to the people who live near facilities, and are most vulnerable to factors such as human-wildlife conflict. As such, it is important to consider what portion of the generated funds are invested locally versus reserved to enrich well-compensated shareholders in the far-away capital. Are local people given opportunities to further their training and education, and to advance their careers within ecotourism organisations? Unfortunately, in many areas of Africa, local people continue to receive only the smallest percentage of money spent by tourists. Similarly, even though national parks themselves may receive large numbers of foreign visitors, governments continue to use only a small percentage of the generated funds on park management (Lindsey et al., 2014; Balmford et al., 2015).
4.3.3 Supporting individual and group identities
Many people care deeply about biodiversity. The thought of a charismatic animal or a special landscape (Figure 4.9) may elicit a strong emotional response, which leads to a desire to protect plants, animals, and natural places. For some people, this desire is associated with a hope to someday see those unique species or landscapes in person. Others do not expect or even desire to see these species and landscape themselves, yet they value their existence. In either case, these individuals recognise the existence values of wildlife and nature—the benefit people receive from simply knowing that an ecosystem or species exists. Bequest values (also known as beneficiary values) is a component of existence values, defined as the perceived benefit people receive from preserving a natural resource or species for future generations.
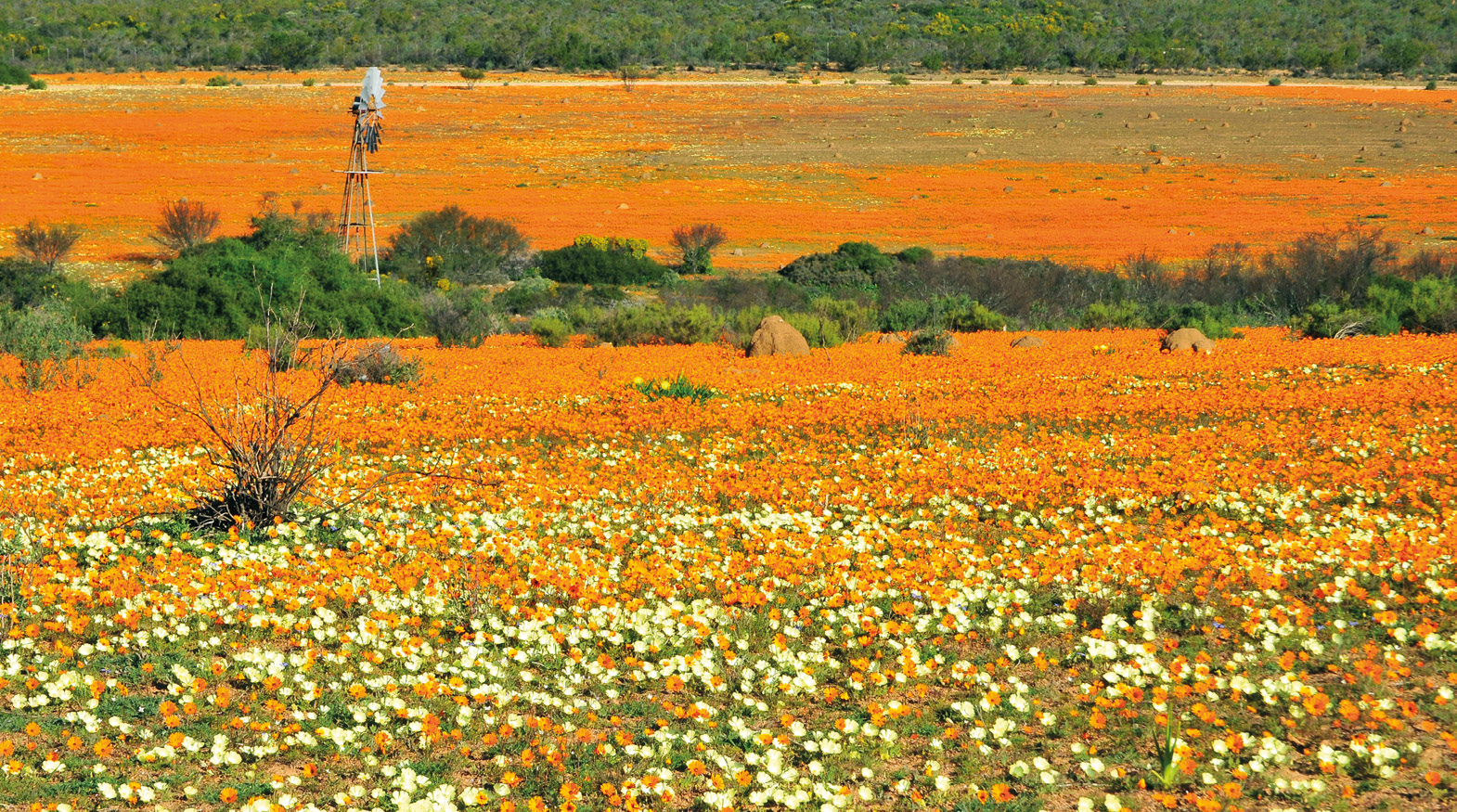
Figure 4.9 Each year, after the first spring rains, South Africa’s Namaqua National Park comes alive with a rich tapestry of colour, attracting wildflower enthusiasts from all over the world to this otherwise barren semi-desert landscape. Photograph by LBM1948, https://commons.wikimedia.org/wiki/File:Sur%C3%A1frica,_Namaqualand_02.jpg, CC BY-SA 4.0.
The desire to ensure the protection of biodiversity has prompted a wide range of people to establish, join, or otherwise contribute to conservation organisations. For many people involved in these organisations, their participation stems from the ethical premise that wildlife are equal to human life, and that biodiversity conservation offers genuine and long-lasting well-being. This environmental philosophy is often described as deep ecology, the ethical premise that species and biodiversity have a right to exist independent of their possible benefits to humans, and that humans have an inherent responsibility to protect species and biodiversity (see also Section 1.4). Deep ecology holds that social structures (including politics, economics, technology, and ideology) must change radically to reduce the destruction of Earth’s biodiversity and to enhance people’s quality of life. It emphasises and prioritises the natural environment, aesthetics, religion, and culture, rather than material consumption. Although the ethical appreciation of biodiversity is similar in deep ecology and conservation biology, deep ecology includes broader goals for personal, social, and political change.
Biodiversity forms the basis of spiritual, celebratory, and other social-cohesion experiences for many people.
Biodiversity also forms the basis of spiritual, celebratory, and other social-cohesion experiences for many people. It ensures people experience a sense of place and belonging, reminds them of childhood experiences, and gives a sense of connection when they experience natural sights, sounds and smells. This is especially true for Africans, many of whom attach deeply-held spiritual, cultural, and symbolic values to the environment. Even the money of most, perhaps all, African countries features aspects of nature, as if to add a little extra (if only symbolic) value to those coins and bills. All these factors play a major role in people’s sense of who we are—our identity.
4.4 The Long-Term View: Option Values
The option values of biodiversity describe nature’s potential to provide currently unknown or unrealised benefits at some point in the future. For example, while many species may not currently have any realised material contributions, a small number of taxa may have enormous potential to support new industries or prevent major agricultural crops from collapsing. For this reason, scientists continuously search for species with hidden uses: entomologists search for insects that can control pest species, microbiologists search for bacteria useful in biochemical manufacturing, and agricultural scientists search for genetic varieties of plants that can produce more food to feed a growing human population. As fears of antibiotic resistance become reality, archaea (widespread single-celled microorganisms with no nucleus which are also thought to be the oldest life forms on Earth) may be used to develop new classes of antibiotic medicine (Metcalf et al., 2014). Some researchers also hope that studying primates—the likely original source of diseases such as HIV/AIDS, Ebola, and malaria (Martin et al., 2005)—may allow us to one day find cures for these diseases. It is worth noting that the effectiveness of using animals to study human diseases remains highly controversial (Archibald and Clotworthy, 2007; Festing and Wilkinson, 2007; Rollin, 2007), and that many people believe that the suffering and death of animals during biomedical research is unethical.
This continuous search for valuable or useful natural products, called bioprospecting, has already contributed a great amount to global economic development, and is expected to become even more important in the coming decades. This is particularly true in the rush to find replacements for climate sensitive crops that may be threatened by climate change. For example, researchers hope that the genetic diversity in wild coffee populations can act as an insurance policy in case our warming planet damages currently popular commercial strains (Davis et al., 2012). There is also much hope that plants from Africa will lead to new medical treatments, for diseases such as malaria, cancer, and high blood pressure (Gurib-Fakim, 2017). It is for reasons such as these that losing even small portions of expansive ecosystems concerns scientists. The extinction of even one valuable species or gene can represent a tremendous loss to humanity, even if many other species are preserved.
Bioprospecting, the search for valuable or useful natural products, has already contributed greatly to global economic development.
4.5 Environmental Economics
It should be clear from reading this chapter that the well-being of people around the world is fundamentally linked to opportunities for biodiversity to survive and prosper. That means that when we destroy an ecosystem or let a species go extinct, we also put at risk our own ability to survive and prosper. To fully account for these risks, decisions that negatively affect biodiversity must account for all the costs and benefits (hidden or otherwise), including the impacts on ecosystem services, before the decision is implemented.
One of the most popular methods for accounting for potential harm to biodiversity, especially when weighing public policy and commercial decisions, is to attach market (or monetary) values to the ecosystem services. For some ecosystem services, it is rather straightforward to estimate a market value. For example, how much would it cost to replace a natural pollination service with hand pollination by farm workers? But for many services, estimating a monetary value is much more difficult. For example, how do we calculate the value of the Congo Basin’s carbon stocks? Where do we even start to estimate the value of breathing clean air, or knowing that dolphins exists?
Environmental economics strengthen arguments for biodiversity protection by examining the contribution of ecosystem services to global economies.
To examine these kinds of complex questions, conservation biologists look to a sub-discipline within economics called environmental economics. Environmental economics broadly examines the contribution of ecosystem services to global economies. An important component of this examination involves estimating the market value of all the different ecosystem services we benefit from, but it also includes studying the environmental costs of economic transactions, environmental policies, and other decisions that impact the environment.
4.5.1 Placing a price on the natural world
Approximating the market values of ecosystem services is no small feat, in part because nature’s contributions to people vary by location and perspective (Díaz et al., 2018). There also continue to be technical (e.g. Kling et al., 2012) and ethical (e.g. McCauley, 2006; Silvertown, 2015) disagreements about the need and methods used to translate nature’s services into monetary terms. Nevertheless, including such estimates has become a widely accepted norm in economics models and conservation activities (Guerry et al., 2015). To accomplish this task, economists rely on seven main methods to estimate the market values of ecosystem services (Farber et al., 2002):
- Market value: The price a person is willing to pay for a specific product or service. For example, how much is a person willing to pay for a bundle of firewood at a local market?
- Avoidance cost: The cost society avoids paying because a specific ecosystem service exists. For example, how much does society avoid paying for water filtration service otherwise provided by a region’s forests and wetlands?
- Replacement cost: The cost society would have incurred if a specific ecosystem service had to be replaced. For example, how much would society have to pay in extra healthcare costs and in clean-up costs for diseased carcasses to replace the sanitation services provided by vultures?
- Factor income: The additional income generated by the enhancement of an ecosystem service. For example, how much would a reduction in water pollution increase the income of fishermen through healthier fish populations?
- Travel cost: The additional travel cost a person is willing to pay to experience an ecosystem service otherwise not available to them. For example, how much extra is a person willing to pay for transport to participate in recreational activities at a cleaner lake?
- Hedonic pricing: The additional expense a person is willing to pay to experience an ecosystem service. For example, how much extra is someone willing to pay for a house with an ocean view, compared to an inland house?
- Contingent valuation: The additional expenses a person is willing to pay for an alternative hypothetical scenario. For example, how much is someone willing to pay for cleaner air, or the right to catch more fish?
The combined value of all of Earth’s ecosystem services may be double the current value of the global economy.
Using a combination of these methods, a range of ecosystem services have been valued in recent years. For example, the services offered by pollinating insects around the world have been valued at US $153 billion per year (Gallai et al., 2008). In just South Africa’s Western Cape Province, free pollination services provided by wild insects to the local fruit industry, valued at US $500 million, has been estimated at nearly US $360 million per year (Allsopp et al., 2008). The replacement cost of tropical forests is also increasingly appreciated in carbon sequestration markets, where heavy greenhouse gas emitters pay huge sums of money to conserve forests to become more carbon neutral (Section 10.4). For example, the United Nations Environmental Programme (UNEP) has estimated that their forests are worth 4.2 times more intact than the value that could be earned through logging; the value of just Kenya’s remaining Mau forest, if left intact, is estimated at US $1.3 billion per year (UNEP, 2012). One ambitious study estimated the value of all of Earth’s ecosystem services at US $145 trillion annually (Costanza et al., 2014), which is almost double the current US $78 trillion value of the global economy. By comparing the value of ecosystem services over time, Costanza et al. (2014) also estimated that we are losing US $4.3–20.2 trillion per year in ecosystem services through land degradation.
4.5.2 Environmental economics’ biggest contributions
Since its development, environmental economics has contributed to conservation biology in several very important ways. Perhaps the most important contribution is that it has enabled conservation biologists to better communicate the value of ecosystem goods and services to audiences like government officials and business leaders, who often base decisions on economic considerations. By doing this, environmental economics has also focussed our attention on the wide range of goods and services that biodiversity provides and has elevated these topics into corridors where they were not previously discussed. These efforts have already paid dividends; in 2012, several Africa countries signed the Gaborone Declaration, a pledge to integrate the value of ecosystem services into their economies.
Environmental economics enable us to better communicate the value of biodiversity to those who base decisions on economic considerations.
Environmental economics also enabled conservation biologists to better account for environmental impacts of environmental damaging activities. In doing so, the field highlighted how activities that appear profitable are running at an economic loss when properly accounting for otherwise ignored environmental (and social) damages. While such calculations have traditionally focussed on imbalances in overharvesting of material contributions (see negative externalities, Section 4.5.3), recent developments have also started accounting for damages inflicted on regulating services and nonmaterial contributions, such as the loss of nature’s contribution to climate regulation (Auffhammer et al., 2017; Hsiang et al., 2017).
4.5.3 Environmental economics’ biggest challenges
Despite all the direct and indirect contributions of environmental economics to biodiversity conservation, there are also several challenges facing the field. Some of these challenges relate to methodological complexities of valuing ecosystem services, but many challenges also have their roots in governance failures. Following is a discussion of the most important challenges facing environmental economics.
Accounting for negative externalities
Modern economics is built on the principle of voluntary transactions—that is, a transaction occurs only when it benefits all the stakeholders involved. However, environmental (and social) harm often arises when some hidden costs are passed on to people not directly involved in the transactions. The unregulated use of open-access resources—resources such as water, air, and fish populations that are freely used by many different groups of people—provide many opportunities for this kind of abuse. Consider a company that dumps chemical waste into a river instead of properly disposing of it. While the company may benefit from this cost-cutting measure, people further downstream bear the environmental and social costs of the company’s “free” waste disposal by having to contend with polluted drinking water, loss of swimming and other recreational opportunities, and loss of fish as a safe food source. Damage inflicted on rivers and other open-access resources also represent a classic example of the tragedy of the commons—while some people initially benefit from abusing the “free” ecosystem services, those values are gradually lost to all of society, including those who abused it (NRC, 2002).
Because negative externalities allow a small number of people to benefit at the expense of the rest of society, they often lead to market failures.
The hidden costs of economic transactions that are passed on to people not directly involved are generally known as negative externalities (Figure 4.10). Because negative externalities allow a small number of people to benefit at the expense of the rest of society, they often lead to market failures, characterised by transactions that do not lead to optimal outcomes for all stakeholders. Governments may correct for these kinds of market failures by imposing taxes on activities that are harmful to the environment. Carbon taxes imposed on greenhouse gas emitters (see climate change, Chapter 6) is a common example. But many times, governance structures fail, or even exacerbate, the impact of negative externalities, by artificially maintaining destructive activities with tax incentives, direct payments, and price regulations. For example, subsidies give foreign fishing fleets operating off Africa a competitive advantage over local fisherman and artificially inflate their profitability despite declining fish populations (Brashares et al., 2004; Sumaila and Pauly, 2006; Mallory, 2013). The financial incentives governments provide to maintain destructive activities are more often referred to as perverse subsidies (Myers and Kent, 2001). The size of perverse subsidies is often very large, regularly dwarfing conservation spending. For example, US $26 billion in subsidies were provided to the Africa’s fossil fuel industry just in 2015 (Whitley and van der Burg, 2015), compared to just US $381 million spent annually to secure Africa’s protected areas with lions (Lindsey et al., 2018).
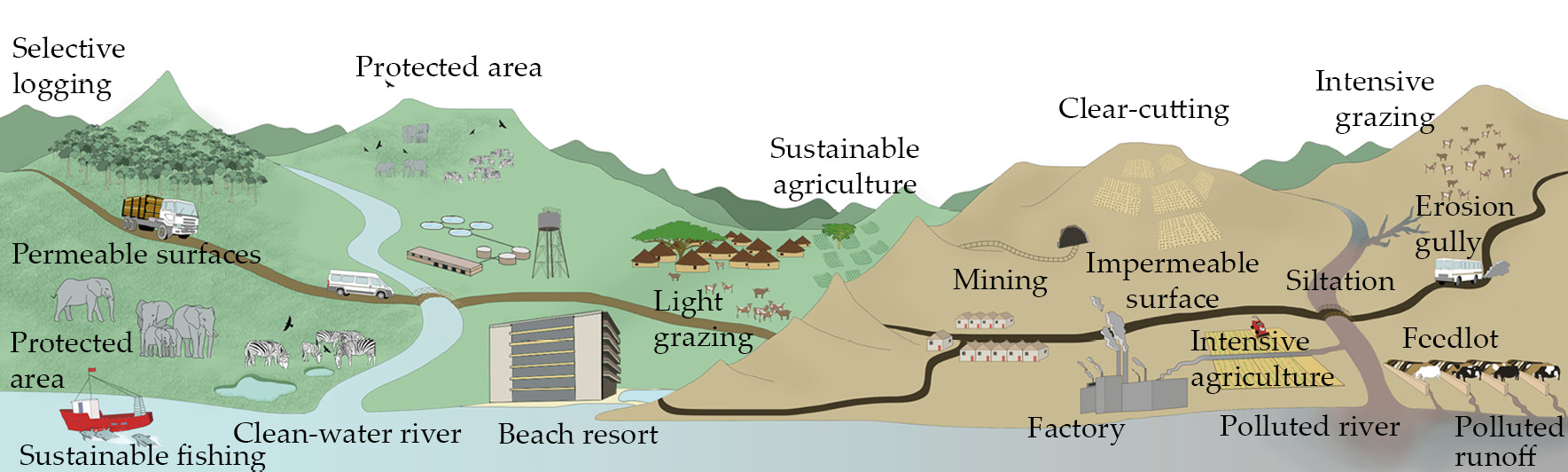
Figure 4.10 Politicians, developers, and industries all too often fail to account for negative externalities (right side of figure) when they consider the contribution of destructive economic activities to society. Accounting for these negative externalities—and redistributing perverse subsidies to activities that provide public benefits (left side of figure)—will help us transition to more sustainable lifestyles. CC BY 4.0.
There are many reasons why governance structures continue to fail nature and allow market failures to occur. For example, due to the prevailing mindset of pursuing economic growth at all costs, politicians, developers, and industries often skew their cost-benefit analyses by prioritising the short-term benefits gained from destructive sectors over long-term societal well-being and sustainability. Another factor is intense lobbying by industries benefitting from perverse subsidies, which leads to corruption and other questionable decisions. Solving these challenges will rely on a society that prioritises economic development (Section 15.1) and establishes structures (i.e. passing and enforcing environmental laws, Chapter 12) that fully account for negative externalities.
Determining ownership
Another problem that plagues environmental economists and other stakeholders is deciding who owns the commercial rights to biodiversity. Imagine a biochemist from a wealthy country traveling in a rural part of West Africa. The biochemist falls sick, but luckily local villagers help the chemist get better with the aid of a traditional healing plant. Once back home, the biochemist scientifically demonstrates that this plant can be used to synthesise a new effective medicine. Do the profits from this new medicine belong to the biochemist, the organisation that sponsored his/her trip, or the local people in the area who helped the biochemist?
Scientists, economists, politicians, and others are currently debating who owns the commercial rights to the world’s biodiversity.
In the past, corporations and scientists (generally from wealthier countries) travelled extensively (often to poorer countries in the tropics) to collect species from which commercially valuable products might be obtained. These new products were then sold, but all profits were kept by the corporations while the people in the poorer source countries received little to no financial benefit. One such example is the production of palm oil, of which Malaysia and Indonesia currently contribute 85% of the global vegetable oil supply. This industry is entirely dependent on the oil palm (Elaeis guineensis, LC), and its specialist pollinator, the oil palm weevil (Elaeidobius kamerunicus), both imported from West Africa. Yet, West Africa have seen little benefit from the profits palm oil generated in Southeast Asia (Mbugua, 2017). (Note this exploitation goes multiple ways; for example, South America has also seen little benefit from profits generated from cacao production in West Africa.)
To combat this unfair exploitation, called biopiracy, many developing countries now require scientists and corporations to obtain permits before they can collect biological material for commercial or research purposes. Also, at the international level, nearly 100 countries have agreed to the fair sharing of benefits arising from the use of biological resources, through the Nagoya Protocol (see Section 12.2.1 for further discussion on international laws). Through these and similar laws and agreements, the hope is that a greater portion of the profits gained from biodiversity will be allocated to people who protect biodiversity and who live in the areas from where it is extracted.
A more inclusive approach
The valuation of ecosystem services has traditionally relied on generalised principles of economics and natural sciences. While this focus enabled scientists to develop broadly applicable themes and metrics in ecosystem evaluation, it also neglected the role of context and culture in understanding nature’s role in people’s lives. Many people have also remained uneasy about commodifying nature (i.e. giving it a market value), because some of the most important contributions of biodiversity are not easily converted into monetary metrics. Consequently, many feared that the transactional approach to ecosystem services would lead to social inequity concerns and alienate people offended by the idea that nature’s metaphysical properties must compete against commercial interests.
To address these concerns, the valuation and classification of ecosystem services are currently undergoing several major transformations. Prominently, the UN’s most recent classification scheme (Díaz et al., 2018) has given a more prominent voice to a wider range of stakeholders, including the social sciences, and recognises the importance of culture and context in nature’s contributions to people. This exciting area of research is actively developing, and readers are encouraged to track developments and reactions associated with 2019 IPBES Global Assessment at https://www.ipbes.net/news/ipbes-global-assessment-preview.
4.6 Summary
- People value biodiversity in many ways. The reasons vary from person to person, and from region to region. But generally, nature’s contributions to people, also called ecosystem services, are divided into three overlapping and interdependent categories, namely material contributions, regulating services, and nonmaterial contributions.
- Material contributions include benefits people get from consuming natural resources (e.g. drinking water or burning wood for cooking) or using natural resources in production and trade (e.g. timber to build homes or other structures).
- Biodiversity also provides a large variety of regulating services that enable people to benefit from nature’s material contributions. Some of these contributions include ecosystem productivity, water and soil protection, climate regulation, pollination, seed dispersal, and disaster prevention and detection.
- People attach nonmaterial values to biodiversity which are difficult to quantify, and thus to account for, in modern economic systems. These values include support for inspiration and learning, support for psychological and physical experiences, and support for personal and group identities.
- Environmental economics studies the implications of economic transactions, environmental policies, and other decisions that impact the environment. This field has highlighted how damage to the environment, such as pollution caused by industry, are not always fully considered when making political and development decisions, leading to unsustainable economic practices and market failure. Accounting for negative externalities and perverse subsidies can help policymakers design incentives that promote sustainable practices.
4.7 Topics for Discussion
- Think of a recent infrastructure development near where you live, such as a recently-built road or dam. Try to come up with a list of ecosystem services that were damaged by this development. Who carries the costs of these lost services? Do you think the benefits from the development were worth the costs? Explain your answer.
- Do individual organisms, populations, species, and biological communities have rights? What about physical features such as lakes, rivers, and mountains? While explaining your answer, also think about where we should draw the line of moral responsibility in how we care for nature.
- A European botanist on holiday visits your area. During a short hike, you show this botanist a plant used as a traditional treatment for malaria. The botanist takes samples of this plant back to Europe, where subsequent testing shows that it can be used to develop an effective anti-malarial drug. Who do you think should receive the profits from this new drug? The botanist who undertook the trip, and you because you showed the botanist the plant? What about the organisation that funded the drug’s development, and the scientists who synthesised the new drug? What about all the people who educated you and your family in the plant’s value? If the profits belong to multiple entities, how should it be divided?
- More than a decade ago, the shark ecotourism industry at Gansbaai, South Africa, was estimated at US $4.4 million annually (Hara et al., 2003)—it has been increasing ever since. There are an estimated 900 great white sharks (Carcharodon carcharias, VU) living in Gansbaai (Towner et al., 2013). Assuming the average white shark lives for 70 years (Hamady et al., 2014), what is the value of each shark at Gansbaai? Can you find (or estimate) the price that a single shark sold for food would obtain on the world market? How do these values compare? What do you think is the best use of the sharks?
4.8 Suggested Readings
Allsopp, M.H., W.J. de Lange, and R. Veldtman. 2008. Valuing insect pollination services with cost of replacement. PLoS ONE 3: e3128. https://doi.org/10.1371/journal.pone.0003128 A study estimating the economic value of local pollination services.
Costanza, R., R. de Groot, P. Sutton, et al. 2014. Changes in the global value of ecosystem services. Global Environmental Change 26: 152–58. https://doi.org/10.1016/j.gloenvcha.2014.04.002 An attempt to value all ecosystem services.
Farber, S.C., R. Costanza, and A.M. Wilson. 2002. Economic and ecological concepts for valuing ecosystem services. Ecological Economics 41: 375–92. https://doi.org/10.1016/S0921-8009(02)00088-5 Methods for estimating the value of ecosystem services.
Isbell, F., V. Calcagno, A. Hector, et al. 2011. High plant diversity is needed to maintain ecosystem services. Nature 477: 199–202. https://doi.org/10.1038/nature10282 Maintaining ecosystem services requires protecting a diversity of species.
Koné, I., J.E. Lambert, J. Refisch, et al. 2008. Primate seed dispersal and its potential role in maintaining useful tree species in the Taï region, Côte d’Ivoire: Implications for the conservation of forest fragments. Tropical Conservation Science 1: 293–306. https://doi.org/10.1177%2F194008290800100309 Maintaining primate populations is important also for humans who rely on forest resources.
Markandya, A., T. Taylor, A. Longo, et al. 2008. Counting the cost of vulture decline—an appraisal of the human health and other benefits of vultures in India. Ecological Economics 67: 194-204. https://doi.org/10.1016/j.ecolecon.2008.04.020 A study illustrating the value of vultures.
Naidoo, R., B. Fisher, A. Manica, et al. 2016. Estimating economic losses to tourism in Africa from the illegal killing of elephants. Nature Communications 7: 13379. https://doi.org/10.1038/ncomms13379 Africa loses US $25 million annually from elephant poaching.
Peterson, G.D., Z.V. Harmackova, M. Meacham, et al. 2018. Welcoming different perspectives in IPBES: “Nature’s contributions to people” and “Ecosystem services”. Ecology and Society 23: 39. https://doi.org/10.5751/ES-10134-230139 Addressing shortcomings of the ecosystem services concept
Schleicher, J., M. Schaafsma, N.D. Burgess, et al. 2018. Poorer without it? The neglected role of the natural environment in poverty and wellbeing. Sustainable Development 25: 83–98. https://doi.org/10.1002/sd.1692 The environment and human well-being are intricately linked.
Bibliography
Abate, T., A. van Huis, and J.K.O. Ampofo. 2000. Pest management strategies in traditional agriculture: An African perspective. Annual Review of Entomology 45: 631–59. https://doi.org/10.1146/annurev.ento.45.1.631
Abernethy, K.A., L. Coad, G. Taylor, et al. 2013. Extent and ecological consequences of hunting in Central African rainforests in the twenty-first century. Philosophical Transactions of the Royal Society B: 368: 20120303. https://doi.org/10.1098/rstb.2012.0303
African Pollinators Initiative. 2007. Crops, Browse and Pollinators in Africa: An Initial Stock-taking (Rome: FAO). http://www.fao.org/3/a-a1504e.pdf
Allsopp, M.H., W.J. de Lange, and R. Veldtman. 2008. Valuing insect pollination services with cost of replacement. PloS ONE 3: e3128. https://doi.org/10.1371/journal.pone.0003128
Archibald, K., and M. Clotworthy. 2007. Comment on ‘The ethics of animal research’ by Festing and Wilkinson. EMBO Reports 8: 794–96. https://doi.org/10.1038/sj.embor.7401057
Auffhammer, M., P. Baylis, and C.H. Hausman. 2017. Climate change is projected to have severe impacts on the frequency and intensity of peak electricity demand across the United States. Proceedings of the National Academy of Sciences 114: 1886–91. https://doi.org/10.1073/pnas.1613193114
Babalola, S.A. 1966. The Content and Form of Yoruba Ijala. Oxford Library of African Literature (Oxford: Oxford University Press).
Bako, S.P., S. Afolabi, and I.I. Funtua. 2008. Spatial distribution and heavy metal content of some bryophytes and lichens in relation to air pollution in Nigeria’s Guinea Savanna. International Journal of Environment and Pollution 33: 195–206. https://doi.org/10.1504/IJEP.2008.019393
Balmford, A., A. Manica, L. Airey, et al. 2004. Hollywood, climate change, and the public. Science 305: 1713. https://doi.org/10.1126/science.305.5691.1713b
Balmford, A., J.M. Green, M. Anderson, et al. 2015. Walk on the wild side: Estimating the global magnitude of visits to protected areas. PloS Biology 13: e1002074. https://doi.org/10.1371/journal.pbio.1002074
Balogun, A.A., T.E. Morakinyo, and O.B. Adegun. 2014. Effect of tree-shading on energy demand of two similar buildings. Energy and Buildings 81: 305–15. https://doi.org/10.1016/j.enbuild.2014.05.046
Benjaminsen, T. 2008. Does supply-induced scarcity drive violent conflicts in the African Sahel? The case of the Tuareg rebellion in northern Mali. Journal of Peace Research 45: 819–36. https://doi.org/10.1177/0022343308096158
Biggs, D., J. Turpie, C. Fabricius, et al. 2011. The value of avitourism for conservation and job creation—an analysis from South Africa. Conservation and Society 9: 80–90. https://doi.org/10.4103/0972-4923.79198
Bodin, N., R. N’Gom-Kâ, S. Kâ, et al. 2013. Assessment of trace metal contamination in mangrove ecosystems from Senegal, West Africa. Chemosphere 90: 150–57. https://doi.org/10.1016/j.chemosphere.2012.06.019
Bonnell, T.R., R. Reyna-Hurtado, and C.A. Chapman. 2011. Post-logging recovery time is longer than expected in an East African tropical forest. Forest Ecology and Management 261: 855–64. https://doi.org/10.1016/j.foreco.2010.12.016
Boreyko, J.B., R.R. Hansen, K.R. Murphy, et al. 2016. Controlling condensation and frost growth with chemical micropatterns. Scientific Reports 6: 19131. https://doi.org/10.1038/srep19131
Brashares J.S., C.W. Epps, and C.J. Stoner. 2010. Ecological and conservation implications of mesopredator release. In: Trophic Cascades, ed. By J. Terborgh and J. Estes (Washington: Island Press).
Brashares, J.S., P. Arcese, M.K. Sam, et al. 2004. Bushmeat hunting, wildlife declines, and fish supply in West Africa. Science 306: 1180–83. https://doi.org/10.1126/science.1102425
Brito, J.C., S.M. Durant, N. Pettorelli, et al. 2018. Armed conflicts and wildlife decline: Challenges and recommendations for effective conservation policy in the Sahara-Sahel. Conservation Letters 11: e12446. http://doi.org/10.1111/conl.12446
Cagua E.F., N. Collins, J. Hancock, et al. 2014. Whale shark economics: A valuation of wildlife tourism in South Ari Atoll, Maldives. PeerJ 2: e515. https://doi.org/10.7717/peerj.515
Chamaillé-Jammes, S., H. Fritz, M. Valeix, et al. 2008. Resource variability, aggregation and direct density dependence in an open context: The local regulation of an African elephant population. Journal of Animal Ecology 77: 135–44. https://doi.org/10.1111/j.1365-2656.2007.01307.x
Clark, L. 2012. This self-filling water bottle mimics a desert beetle. Wired Magazine. http://wrd.cm/1OcZRnF
Coad, L., J. Schleicher, E.J. Milner-Gulland, et al. 2013. Social and ecological change over a decade in a village hunting system, central Gabon. Conservation Biology 27: 270–80. https://doi.org/10.1111/cobi.12012
Coad, L., K. Abernethy, A. Balmford, et al. 2010. Distribution and use of income from bushmeat in a rural village, Central Gabon: Bushmeat income in Gabon. Conservation Biology 24: 1510–18. https://doi.org/10.1111/j.1523-1739.2010.01525.x
Costanza, R., R. de Groot, P. Sutton, et al. 2014. Changes in the global value of ecosystem services. Global Environmental Change 26: 152–58. https://doi.org/10.1016/j.gloenvcha.2014.04.002
Cumming, D.H.M., M.B. Fenton, I.L. Rautenbach, et al. 1997. Elephants, woodlands and biodiversity in southern Africa. South African Journal of Science 93: 231–36.
Daskin, J.H., and R.M. Pringle. 2018. Warfare and wildlife declines in Africa’s protected areas. Nature 553: 328–32. https://doi.org/10.1038/nature25194
Davis A.P., T.W. Gole, S. Baena, et al. 2012. The impact of climate change on indigenous arabica coffee (Coffea arabica): Predicting future trends and identifying priorities. PloS ONE 7: e47981. https://doi.org/10.1371/journal.pone.0047981
Diamond, J. 2011. Collapse: How Societies Choose to Fail or Succeed (London: Penguin Books).
Díaz, S., U. Pascual, M. Stenseke, et al. 2018. Assessing nature’s contributions to people. Science 359: 270–72. https://doi.org/10.1126/science.aap8826
Dirzo, R., H.S. Young, M. Galetti, et al., 2014. Defaunation in the Anthropocene. Science 345: 401–06. https://doi.org/10.1126/science.1251817
Duthie, E., D. Veríssimo, A. Keane, et al. 2017. The effectiveness of celebrities in conservation marketing. PloS ONE 12: e0180027. https://doi.org/10.1371/journal.pone.0180027
Ehrlich, P., and B. Walker. 1998. Rivets and redundancy. BioScience 48: 387–88. https://doi.org/10.2307/1313377
Ellis A.M., S.S. Myers, and T.H. Ricketts. 2015. Do pollinators contribute to nutritional health? PloS ONE 10: e114805. https://doi.org/10.1371/journal.pone.0114805
Estes, J.A., J. Terborgh, J.S. Brashares, et al. 2011. Trophic downgrading of planet Earth. Science 333: 301–06 https://doi.org/10.1126/science.1205106
Farber, S.C., R. Costanza, and A.M. Wilson. 2002. Economic and ecological concepts for valuing ecosystem services. Ecological Economics 41: 375–92. https://doi.org/10.1016/S0921-8009(02)00088-5
Festing, S., and R. Wilkinson. 2007. The ethics of animal research. EMBO Reports 8: 526–30. https://doi.org/10.1038/sj.embor.7400993
Fosso-Kankeu, E., and A.F. Mulaba-Bafubiandi. 2014. Implication of plants and microbial metalloproteins in the bioremediation of polluted waters: A review. Physics and Chemistry of the Earth 67: 242–52. https://doi.org/10.1016/j.pce.2013.09.018
Gallagher, A.J., and C.P.M. Huveneers. 2018. Emerging challenges to shark-diving tourism. Marine Policy 96: 9–12. https://doi.org/10.1016/j.marpol.2018.07.009
Gallai, N., J.M. Salles, J. Settele, et al. 2009. Economic valuation of the vulnerability of world agriculture confronted with pollinator decline. Ecological Economics 68: 810–21. https://doi.org/10.1016/j.ecolecon.2008.06.014
Garcia-Carreras, L., and D.J. Parker. 2011. How does local tropical deforestation affect rainfall? Geophysical Research Letters 38: L19802. https://doi.org/10.1029/2011GL049099
Garibaldi, L.A., I. Steffan-Dewenter, R. Winfree, et al. 2013. Wild pollinators enhance fruit set of crops regardless of honey bee abundance. Science 339: 1608–11. https://doi.org/10.1126/science.1230200
Gemmill-Herren, B., and A.O. Ochieng. 2008. Role of native bees and natural habitats in eggplant (Solanum melongena) pollination in Kenya. Agriculture, Ecosystems and Environment 127: 31–36. https://doi.org/10.1016/j.agee.2008.02.002
Gleick, P.H. 2014. Water, drought, climate change, and conflict in Syria. Weather, Climate, and Society 6: 331–40. https://doi.org/10.1175/WCAS-D-13-00059.1
Gorokhovich, Y., S. Doocy, F. Walyawula, et al. 2013. Landslides in Bududa, eastern Uganda: Preliminary assessment and proposed solutions. In: Landslide Science and Practice, ed. by C. Margottini et al. (Berlin: Springer). https://doi.org/10.1007/978-3-642-31325-7
Graziosi, I., N. Minato, E. Alvarez, et al. 2016. Emerging pests and diseases of South‐east Asian cassava: A comprehensive evaluation of geographic priorities, management options and research needs. Pest Management Science 72: 1071–89. https://doi.org/10.1002/ps.4250
Guerry, A.D., S. Polasky, J. Lubchenco, et al. 2015. Natural capital and ecosystem services informing decisions: From promise to practice. Proceedings of the National Academy of Sciences 112: 7348–55. https://doi.org/10.1073/pnas.1503751112
Gurib-Fakim, A. 2017. Capitalize on African biodiversity. Nature 548: 7. https://doi.org/10.1038/548007a
Hairston, N.G., F.E. Smith, and L.B. Slobodkin. 1960. Community structure, population control, and competition. American Naturalist 94: 421–25. https://doi.org/10.1086/282146
Hamady, L.L., L.J. Natanson, G.B. Skomal, et al. 2014. Vertebral bomb radiocarbon suggests extreme longevity in white sharks. PloS ONE 9: e84006. https://doi.org/10.1371/journal.pone.0084006
Hanson, T., T.M. Brooks, G.A. Da Fonseca, et al. 2009. Warfare in biodiversity hotspots. Conservation Biology 23: 578–87. https://doi.org/10.1111/j.1523-1739.2009.01166.x
Hara, M., I. Maharaj, and L. Pithers. 2003. Marine-based tourism in Gansbaai: A socio-economic study (Cape Town: DEAT).
Hayward, M.W., M.J. Somers, G.I. Kerley, et al. 2012. Animal ethics and ecotourism. South African Journal of Wildlife Research 42: iii–v. https://doi.org/10.3957/056.042.0207
Holdo, R.M., A.R.E. Sinclair, A.P. Dobson, et al. 2009. A disease-mediated trophic cascade in the Serengeti and its implications for ecosystem C. PloS Biology 7: e1000210. https://doi.org/10.1371/journal.pbio.1000210
Hsiang, S., R. Kopp, A. Jina, et al. 2017. Estimating economic damage from climate change in the United States. Science 356: 1362–69. http://doi.org/10.1126/science.aal4369
Hughes, N.J. 2011. The economic value of Congo Basin protected areas goods and services. Journal of Sustainable Development 4: 130–42
Ingram, D., L.M. Coad, B. Collen, et al. 2015. Indicators for wild animal offtake: Methods and case study for African mammals and birds. Ecology and Society 20: 40. http://doi.org/10.5751/ES-07823-200340
Jaramillo J., E. Muchugu, F.E. Vega, et al. 2011. Some like it hot: The influence and implications of climate change on coffee berry borer (Hypothenemus hampei) and coffee production in East Africa. PloS ONE 6: e24528. https://doi.org/10.1371/journal.pone.0024528
Jouquet, P., S. Traoré, C. Choosai, et al. 2011. Influence of termites on ecosystem functioning. Ecosystem services provided by termites. European Journal of Soil Biology 47: 215–22. https://doi.org/10.1016/j.ejsobi.2011.05.005
Kardan, O., P. Gozdyra, B. Misic, et al. 2015. Neighborhood greenspace and health in a large urban center. Scientific Reports 5: 11610. https://doi.org/10.1038/srep11610
Khan, S., S. Nadir, Z.U. Shah, et al. 2017. Biodegradation of polyester polyurethane by Aspergillus tubingensis. Environmental Pollution 225: 469–80. https://doi.org/10.1016/j.envpol.2017.03.012
Khan, Z.R., C.A. Midega, T.J. Bruce, et al. 2010. Exploiting phytochemicals for developing a ‘push–pull’crop protection strategy for cereal farmers in Africa. Journal of Experimental Botany 61: 4185–96. https://doi.org/10.1093/jxb/erq229
Kling, C.L., D J. Phaneuf, and J. Zhao. 2012. From Exxon to BP: Has some number become better than no number? Journal of Economic Perspectives 26: 3–26. https://doi.org/10.1257/jep.26.4.3
Koné, I., J.E. Lambert, J. Refisch, et al. 2008. Primate seed dispersal and its potential role in maintaining useful tree species in the Taï region, Côte-d’Ivoire: Implications for the conservation of forest fragments. Tropical Conservation Science 1: 293–306. https://doi.org/10.1177/194008290800100309
LaLonde, K.B., A. Mahoney, T.L. Edwards, et al. 2015. Training pouched rats to find people. Journal of Applied Behavior Analysis 48: 1–10. https://doi.org/10.1002/jaba.181
Lassen, K.M., A. Ræbild, H. Hansen, et al. 2012. Bats and bees are pollinating Parkia biglobosa in The Gambia. Agroforestry Systems 85: 465–75. https://doi.org/10.1007/s10457-011-9409-0
Lawrence, D., and K. Vandecar. 2015. Effects of tropical deforestation on climate and agriculture. Nature Climate Change 5: 27–36. https://doi.org/10.1038/nclimate2430
Lindsey P.A., V.R. Nyirenda, J.L. Barnes, et al. 2014. Underperformance of African protected area networks and the case for new conservation models: Insights from Zambia. PloS ONE 9: e94109. https://doi.org/10.1371/journal.pone.0094109
Lindsey, P.A., J.R.B. Miller, L.S. Petracca, et al. 2018. More than $1 billion needed annually to secure Africa’s protected areas with lions. Proceedings of the National Academy of Sciences: E10788-E10796. https://doi.org/10.1073/pnas.1805048115
Lovelock, J. 1988. The Ages of Gaia: A Bibliography of Our Living Earth (Oxford: Oxford University Press).
Loveridge, J.P., and S.R. Moe. 2004. Termitaria as browsing hotspots for African megaherbivores in miombo woodland. Journal of Tropical Ecology 20: 337–43. https://doi.org/10.1017/S0266467403001202
Mahoney, A., T.L. Edwards, K.B. LaLonde, et al. 2014a. Pouched rats’ (Cricetomys gambianus) detection of Salmonella in horse feces. Journal of Veterinary Behavior 9: 124–26. https://doi.org/10.1016/j.jveb.2014.02.001
Mallory, T.G. 2013. China’s distant water fishing industry: Evolving policies and implications. Marine Policy 38: 99–108. https://doi.org/10.1016/j.marpol.2012.05.024
Markakis, J. 1995. Environmental Degradation and Social Conflict in the Horn of Africa. In: Environmental Crisis: Regional Conflicts and Ways of Cooperation, ed. by K.R. Spillman and G. Bächler (Zurich: Center for Security Studies; Berne: Swiss Peace Foundation). http://www.css.ethz.ch/content/dam/ethz/special-interest/gess/cis/center-for-securities-studies/pdfs/Environmental_Crisis_1995.pdf
Markandya, A., T. Taylor, A. Longo, et al. 2008. Counting the cost of vulture decline—an appraisal of the human health and other benefits of vultures in India. Ecological Economics 67: 194–204. https://doi.org/10.1016/j.ecolecon.2008.04.020
Martin, M.J., J.C. Rayner, P. Gagneux, et al. 2005. Evolution of human-chimpanzee differences in malaria susceptibility: Relationship to human genetic loss of N-glycolylneuraminic acid. Proceedings of the National Academy of Sciences 102: 12819–24. https://doi.org/10.1073/pnas.0503819102
Mbugua, S. 2017. Conservation leaders in Africa call for a crackdown on biopiracy. Mongabay https://news.mongabay.com/2017/10/conservation-leaders-in-africa-call-for-a-crackdown-on-biopiracy
McCauley, D.J. 2006. Selling out on nature. Nature 443: 27–28. https://doi.org/10.1038/443027a
Metcalf, J.A., L.J. Funkhouser-Jones, K. Brileya, et al. 2014. Antibacterial gene transfer across the tree of life. Elife 3: e04266. https://doi.org/10.7554/eLife.04266
Morakinyo, T.E., A.A. Balogun, and O.B. Adegun. 2013. Comparing the effect of trees on thermal conditions of two typical urban buildings. Urban Climate 3: 76–93. http://doi.org/10.1016/j.uclim.2013.04.002
Myers, N., and J. Kent. 2001 Perverse Subsidies: How Tax Dollars Can Undercut the Environment and the Economy (Washington: Island Press).
Nackoney, J., G. Molinario, P. Potapov, et al. 2014. Impacts of civil conflict on primary forest habitat in northern Democratic Republic of the Congo, 1990–2010. Biological Conservation 170: 321–28. https://doi.org/10.1016/j.biocon.2013.12.033.
Neuenschwander, P. 2001. Biological control of the cassava mealybug in Africa: A review. Biological Control 21: 214–29. https://doi.org/10.1006/bcon.2001.0937
NRC (National Research Council). 2000. Watershed Management for Potable Water Supply: Assessing the New York City Strategy (Washington: National Academy Press). https://doi.org/10.17226/9677
NRC (National Research Council). 2002. The drama of the commons (Washington: National Academies Press). https://doi.org/10.17226/10287
O’Bryan, C.J., A.R. Braczkowski, H.L. Beyer, et al. 2018. The contribution of predators and scavengers to human well-being. Nature Ecology and Evolution 2: 229–36. https://doi.org/10.1038/s41559-017-0421-2
Oaks, J.L., M. Gilbert, M.Z. Virani, et al. 2004. Diclofenac residues as the cause of vulture population decline in Pakistan. Nature 427: 630–33. https://doi.org/10.1038/nature02317
Ogada, D., P. Shaw, R.L. Beyers, et al. 2015. Another continental vulture crisis: Africa’s vultures collapsing toward extinction. Conservation Letters 9: 98–97. https://doi.org/10.1111/conl.12182
Ogueke, N.V., A.F. Nwakanma, T. Ngharamike, et al. 2017. Energy-saving potentials of some local trees. Energy Efficiency 10: 171–81.
Paine, R.T. 1969. A note on trophic complexity and community stability. American Naturalist 103: 91–93. https://doi.org/10.1086/282586
Paiva, V.H., P. Geraldes, I. Rodrigues, T. Melo, et al. 2015. The foraging ecology of the endangered Cape Verde shearwater, a sentinel species for marine conservation off West Africa. PloS ONE 10: e0139390. https://doi.org/10.1371/journal.pone.0139390
Parker, A.R., and C.R. Lawrence. 2001. Water capture by a desert beetle. Nature 414: 33–34. https://doi.org/10.1038/35102108
Parsa, S., T. Kondo, and A. Winotai. 2012. The cassava mealybug (Phenacoccus manihoti) in Asia: First records, potential distribution, and an identification key. PloS ONE 7: e47675. https://doi.org/10.1371/journal.pone.0047675
Prime Minister’s Task Force. 2009. Report of the Prime Minister’s Task Force on the conservation of the Mau Forests Complex (Nairobi: Prime Minister’s Task Force). https://www.kws.go.ke/file/1448/download?token=vy_ga198
Pringle, R.M. 2008. Elephants as agents of habitat creation for small vertebrates at the patch scale. Ecology 89: 26–33. https://doi.org/10.1890/07-0776.1
Reither K., L. Jugheli, T.R. Glass, et al. 2015. Evaluation of giant African pouched rats for detection of pulmonary tuberculosis in patients from a high-endemic setting. PloS ONE 10: e0135877. https://doi.org/10.1371/journal.pone.0135877
Rollin, B.E. 2007. Animal research: A moral science. EMBO Reports 8: 521–25. https://doi.org/10.1038/sj.embor.7400996
Samnegård, U., P.A. Hambäck, S. Nemomissa, et al. 2014. Dominance of the semi-wild honeybee as coffee pollinator across a gradient of shade-tree structure in Ethiopia. Journal of Tropical Ecology 30: 401–08. https://doi.org/10.1017/S0266467414000327
Schulz, R., and S.K.C. Peall. 2001. Effectiveness of a constructed wetland for retention of nonpoint-source pesticide pollution in the Lourens River catchment, South Africa. Environmental Science and Technology 35: 422–26. https://doi.org/10.1021/es0001198
Scott, K. 2011. Airdrop water harvester wins 2011 James Dyson Award. Wired Magazine. http://www.wired.co.uk/article/dyson-award-2011-winners
Silk, M.J., S.L. Crowley, A.J. Woodhead, et al. 2018. Considering connections between Hollywood and biodiversity conservation. Conservation Biology 31: 597–606. https://doi.org/10.1111/cobi.13030
Silvertown, J. 2015 Have ecosystem services been oversold? Trends in Ecology and Evolution 30: 641–48. http://doi.org/10.1016/j.tree.2015.08.007
Sumaila, U.R., A.S. Khan, A.J. Dyck, et al. 2010. A bottom-up re-estimation of global fisheries subsidies. Journal of Bioeconomics 12: 201–25. https://doi.org/10.1007/s10818-010-9091-8
Taylor, P.J., I. Grass, A.J. Alberts, et al. 2018. Economic value of bat predation services—a review and new estimates from macadamia orchards. Ecosystem Services 30C: 372–81. https://doi.org/10.1016/j.ecoser.2017.11.015
Thiel, M., M.A. Penna-Díaz, G. Luna-Jorquera, et al. 2014. Citizen scientists and marine research: Volunteer participants, their contributions, and projection for the future. Oceanography and Marine Biology 52: 257–314. https://doi.org/10.1201/b17143
Towner, A.V., M.A. Wcisel, R.R. Reisinger, et al. 2013. Gauging the threat: The first population estimate for white sharks in South Africa using photo identification and automated software. PloS ONE 8: e66035. https://doi.org/10.1371/journal.pone.0066035
Turpie, J.K., B.J. Heydenrych, and S.J. Lamberth. 2003. Economic value of terrestrial and marine biodiversity in the Cape Floristic Region: Implications for defining effective and socially optimal conservation strategies. Biological Conservation 112: 233–51. https://doi.org/10.1016/S0006-3207(02)00398-1
UNEP. 2012. The role and contribution of montane forests and related ecosystem services to the Kenyan economy (Nairobi: UNEP). http://wedocs.unep.org/handle/20.500.11822/8513
Valeix, M., H. Fritz, R. Sabatier, et al. 2011. Elephant-induced structural changes in the vegetation and habitat selection by large herbivores in an African savanna. Biological Conservation 144: 902–12. http://doi.org/10.1016/j.biocon.2010.10.029
van Bochove, J., E. Sullivan, and T. Nakamura. 2014. The Importance of Mangroves to People: A Call to Action (Cambridge: UNEP). http://wedocs.unep.org/handle/20.500.11822/9300
van der Plas, F., R. Howison, J. Reinders, et al. 2013. Functional traits of trees on and off termite mounds: understanding the origin of biotically‐driven heterogeneity in savannas. Journal of Vegetation Science 24: 227–38. https://doi.org/10.1111/j.1654-1103.2012.01459.x
Vianna, G.M.S., M.G. Meekan, D.J. Pannell, et al. 2012. Socio-economic value and community benefits from shark-diving tourism in Palau: A sustainable use of reef shark populations. Biological Conservation 145: 267–77. https://doi.org/10.1016/j.biocon.2011.11.022
Whitley S., and L. van der Burg, 2015. Fossil fuel subsidy reform in sub-Saharan Africa: from rhetoric to reality (London and Washington: New Climate Economy). https://newclimateeconomy.report/workingpapers/workingpaper/fossil-fuel-subsidy-reform-in-sub-saharan-africa-from-rhetoric-to-reality-2
Wicander, S., and L.M. Coad. 2015. Learning our Lessons: A Review of Alternative Livelihood Projects in Central Africa (Gland: IUCN). https://www.iucn.org/content/learning-our-lessons-review-alternative-livelihood-projects-central-africa
WWF, and BSI. 2006. Poverty and environmental issues: Governance institutions, institutional frameworks and opportunities for communities (Nairobi: UNEP). https://www.unpei.org/sites/default/files/e_library_documents/kenya-poverty-environment-issues.pdf
Yirga, G., H. Leirs, H.H. de Iongh, et al. 2015. Spotted hyena (Crocuta crocuta) concentrate around urban waste dumps across Tigray, northern Ethiopia. Wildlife Research 42: 563–69. https://doi.org/10.1071/WR14228
Zeddies, J., R.P. Schaab, P. Neuenschwander, et al. 2001. Economics of biological control of cassava mealybug in Africa. Agricultural Economics 24: 209–19. https://doi.org/10.1111/j.1574-0862.2001.tb00024.x