5. The Scramble for Space
© 2019 J.W. Wilson and R.B. Primack, CC BY 4.0 https://doi.org/10.11647/OBP.0177.05
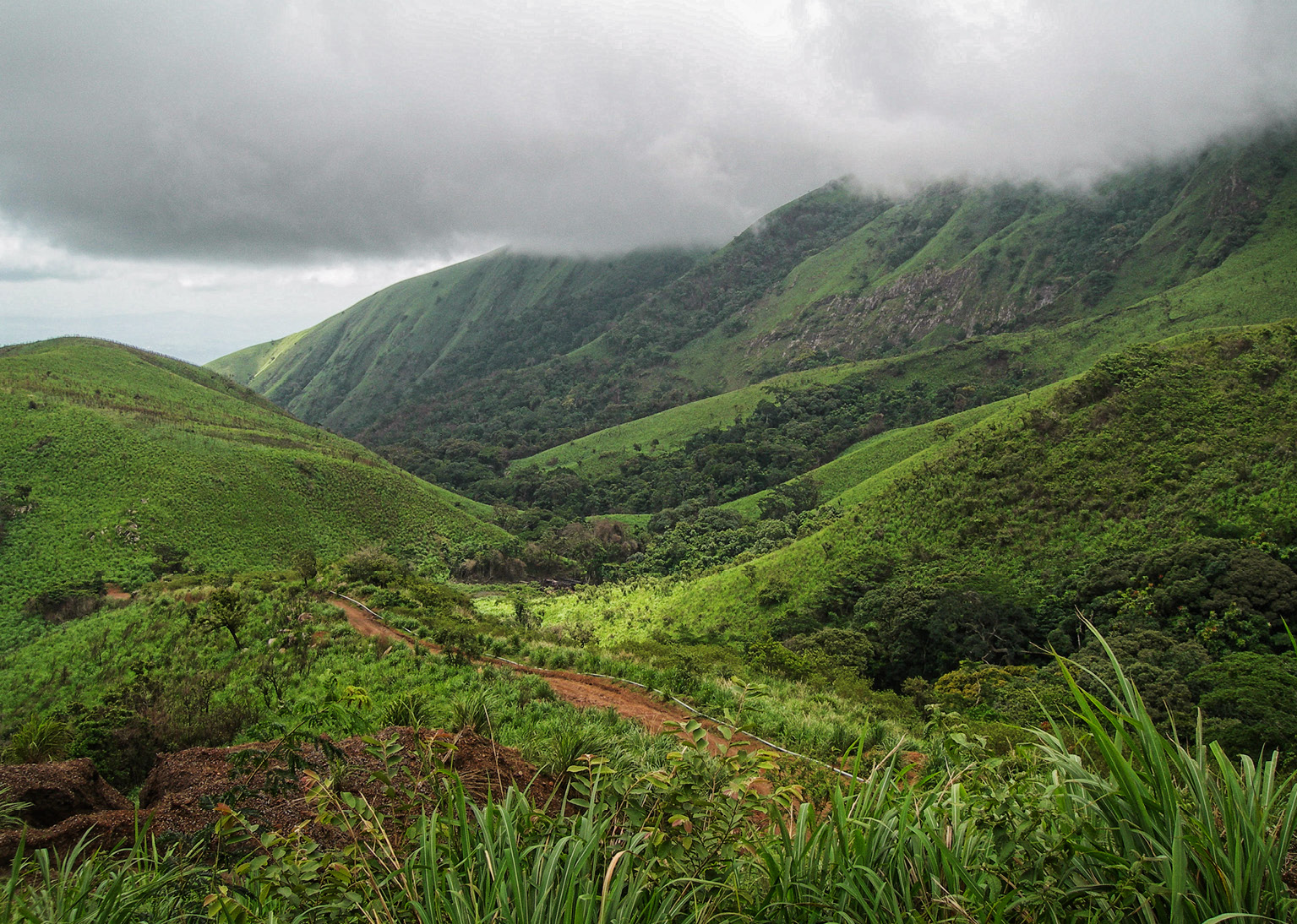
Mount Nimba Strict Nature Reserve, which straddles the border region of Guinea and Côte d’Ivoire, is a World Heritage Site situated in the Guinean Forest of West Africa Biodiversity Hotspot. The ecosystem, which supports chimpanzees that use stone tools, is threatened by iron mining, agriculture, and deforestation. Photograph by Guy Debonnet, http://whc.unesco.org/en/documents/123989, CC BY-SA 3.0 IGO.
The right to shelter, food, and association are basic human needs recognised in many international charters and country constitutions. Like humans, wildlife also needs areas where they can find protection, nourishment, and mates to have any hope of survival. The area where a species can survive and meet their basic needs is known as its habitat. It is often useful to think of a habitat as a multi-dimensional space, characterised by suitable levels of many different environmental variables. Some species, including humans, are highly tolerant of changes in their environmental conditions; consequently, such generalist species find it relatively easy to move to a new area in the unfortunate event that their “home” is destroyed. In contrast, specialist species—those that can only survive within a narrow range of environmental conditions—often do not have anywhere else to go when their habitat is lost, and consequently they go extinct.
In a world where intact natural ecosystems are increasingly being altered by the activities of an ever-increasing human population and its consumptive needs, habitat loss has emerged as the number one threat facing biodiversity today. The expansion of human activity causes massive disturbances to natural ecosystems by altering, degrading, and outright destroying wildlife habitats. A number of specialist species have already been pushed to extinction. But even generalist species are increasingly falling victim to habitat loss: pushed out of their shrinking habitats, they come into conflict with humans while trying to meet their needs near urban centres and on agricultural land. Eventually our own lives will suffer, whether through lost ecosystem services, or sorrow for all the wonderful landscapes and species that have disappeared under our watch. In this chapter we delve into the causes and consequences of this increased competition for space between man and wildlife.
5.1 What is Habitat Loss?
Habitat loss is defined as the outright destruction of natural ecosystems, an inevitable consequence of expanding human populations and human activities. The theory of island biogeography (MacArthur and Wilson, 1967) offers a good explanation for why habitat loss drives species extinctions. Using oceanic islands as a model system, one of the theory’s main predictions is that large islands have more species than small ones because they can accommodate more individuals, which causes those species to be better buffered against extinctions (Section 8.7). Empirical evidence offers strong support for this observation, also known as the species-area relationship. For example, large African islands generally hold more bird species than small islands (Figure 5.1). In addition, 62 of the 79 (63%) Sub-Saharan Africa’s species that went extinct over the past few centuries (IUCN, 2019) have been confined to oceanic islands, rather than the continental mainland which in effect functions like one very big island.
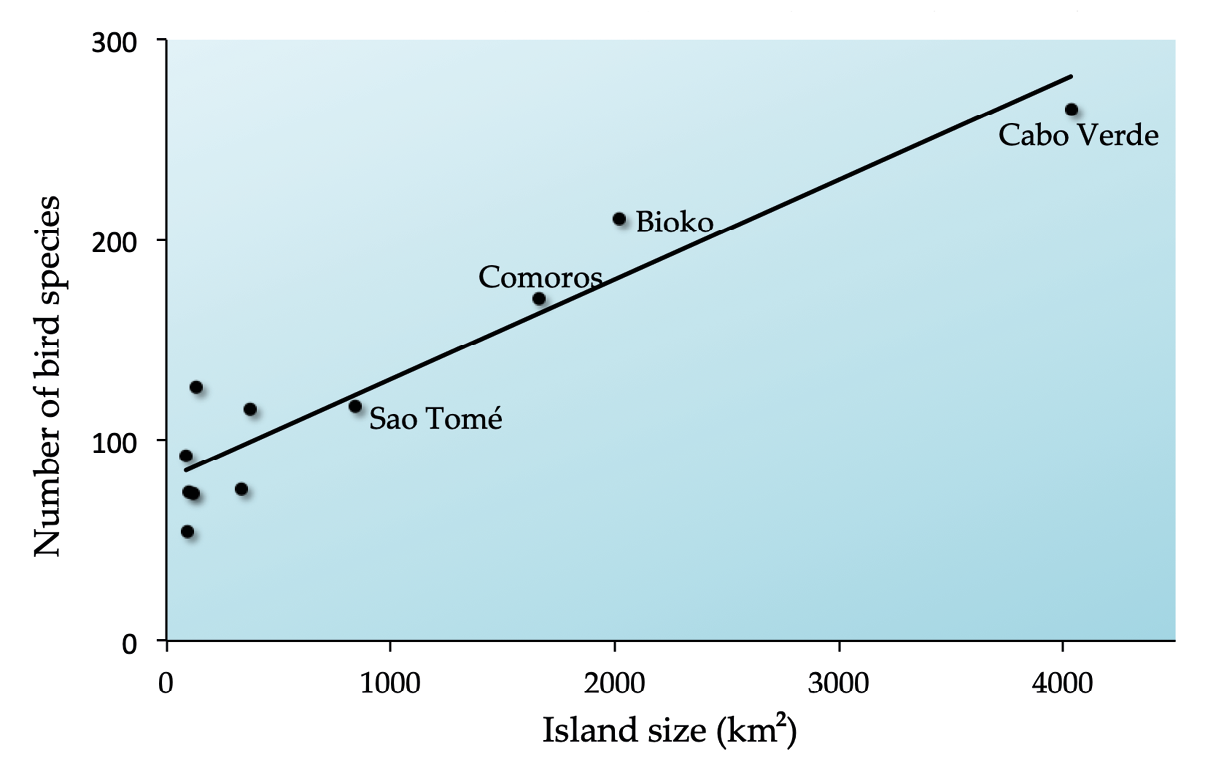
Figure 5.1 Area size greatly influences species richness, as evidenced by the bird species richness on several prominent volcanic islands around Africa. This observation, known as the species-area relationship, explains why habitat loss is so devastating to biodiversity—the more we reduce the amount of habitat left for species to live in, the more extinctions we will see in the coming years. Source: Avibase (https://avibase.bsc-eoc.org), following BirdLife International 2018 taxonomy, CC BY 4.0
The species-area relationship underpins much of conservation biology today. By applying the relationship’s principles to “islands” of suitable habitat surrounded by a “sea” of damaged or unsuitable habitat (the “matrix”), conservation biologists know that conserving large areas of suitable habitat is much more effective in protecting biodiversity (Box 5.1). This is especially true when trying to protect species that have large home ranges, and/or occur in low densities: they can only live in habitat patches that are large enough to maintain viable populations (Chapter 9 discusses the relationship between population size and population viability in more detail). Observations of extirpations in differently sized habitat patches support this application. For example, researchers have found that nearly 50% of Ghana’s forest bird species are sensitive to habitat size, with 25% of species never found in forest patches smaller than 0.1 km2 (Beier et al., 2002). One Ghanaian species that seems particularly sensitive to habitat patch size is the icterine greenbul (Phyllastrephus icterinus, LC); due to habitat loss, this once-common species decreased by 90% during one study’s 15-year period (Arcilla et al., 2015).
Box 5.1 The Importance of Liberia’s Forest Network to the Survival of the Pygmy Hippopotamus
1Fauna & Flora International,
Harmon Compound, Congo Town,
Monrovia, Liberia.
2Fauna & Flora International,
Cambridge, UK.
mary.molokwu@fauna-flora.org , kathryn.phillips@fauna-flora.org
Remaining populations of the pygmy hippopotamus (Choeropsis liberiensis, EN) are found predominately within transboundary West African rainforests spanning Côte d’Ivoire, Guinea, Liberia, and Sierra Leone (Ransom et al., 2015). Liberia contains the largest intact blocks (over 40%) of this Upper Guinean rainforest, a Global 200 ecoregion (Olson et al., 2002). An elusive animal, little is known about the pygmy hippo’s distribution, population status, and ecology. Pygmy hippo numbers are currently estimated at fewer than 2,500 individuals across its range, with the expectation of further decline as a result of agricultural expansion, logging, development, and hunting (Ransom et al., 2015).
Within Liberia, pygmy hippopotamus populations are found in the major forest blocks of the southeast and northwest (Figure 5.A), which are separated by an area of degraded land with high human densities (FFI and FDA, 2013). The southeastern forest block is made up of several large chunks of national, communal, and protected forests fragmented by logging routes and concessions. Although populations are well documented within protected areas, recent reports indicate that populations also exist outside formally protected forests (Hillers et al., 2017). The establishment and management of forest corridors linking key habitats is therefore a conservation priority.
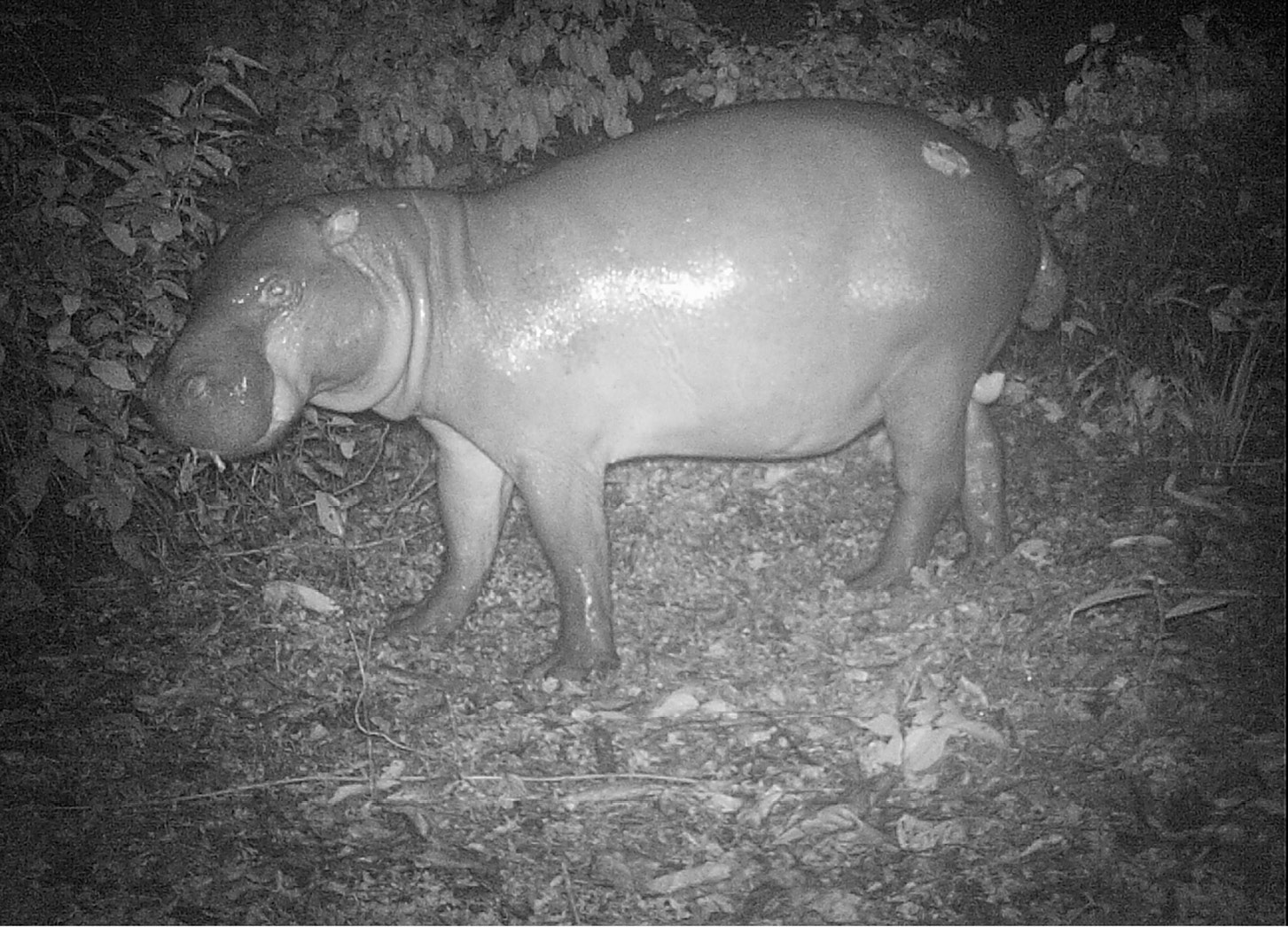
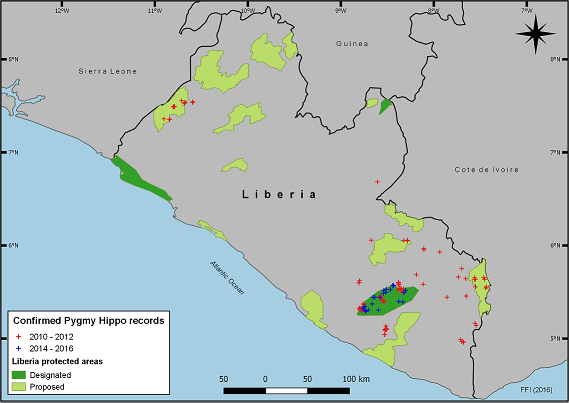
Figure 5.A (Top) Night-time camera trap image of the pygmy hippopotamus taken in Sapo National Park, Liberia. Photograph by FFI, CC BY 4.0. (Bottom) Distribution of pygmy hippopotamus in Liberia based on confirmed records from 2010-2016. Map by Benedictus Freeman/FFI, CC BY 4.0.
In the last few years, huge investment in agriculture, logging, and mining has increased pressure on forests for conversion and from increased human settlements and access roads. Weak law enforcement in Liberia’s protected areas and limited operational capacity has led to increased incursion of illegal activities, such as poaching and mining in these critical habitats. Sapo National Park, Liberia’s only national park and second largest in West Africa after Taï National Park in Côte d’Ivoire, is believed to be a stronghold for the species. However, pygmy hippo numbers remain low at an average encounter rate of 0.12 individuals/km from 2007–2009 (Vogt, 2011) to 0.15 individuals/km (2014–2016 data), i.e. one per 7–8 km, much lower than records from Taï (Vogt, 2011). Sapo National Park has historically suffered from—and continues to suffer from—mining and hunting pressures; hundreds of illegal miners who were evacuated after the civil crises of 2002–2007 and in 2010–2011 and again during the 2014-2015 Ebola crisis, reoccupied a large section of the park, where hunting signs (trails, camps, gun shells) were encountered almost every kilometre of walking within the park’s 1,804 km2 area. The miners have once again been removed from the park, this time with local community support.
Recent efforts to save Liberia’s declining forests have brought about an increase in activities by national and international NGOs and the formation of transboundary collaboration initiatives. For example, an agreement has been established between the Liberian and Sierra Leonean governments, creating the Gola Transboundary Peace Park and significant progress has been made in the development of the Taï-Grebo-Krahn-Sapo Transboundary Forest Complex with Côte d’Ivoire. The Liberian and Guinean government have also commenced a bilateral agreement for the conservation and sustainable management of the Ziama-Wonegizi-Wologizi Transboundary Forest Landscape. Agricultural investments have also evolved to promote public-private partnerships in green-growth and community-based forest protection initiatives. Notable support has also come from the Government of Norway to help Liberia fully halt deforestation by 2020.
Fauna & Flora International (FFI) has worked in Liberia since 1997 focusing on the pygmy hippopotamus as a flagship species. These efforts have contributed to increased knowledge of the species in Liberia, including recording (in collaboration with the Zoological Society of London, ZSL) the first footage of the species in Liberia. FFI also developed a pygmy hippopotamus national action plan and will be revising the regional conservation strategy for the pygmy hippo in 2019. FFI has also established monitoring programmes and a training and research centre at Sapo National Park. FFI’s capacity building programme saw the development of the first conservation biology curriculum for Liberia’s premier university and engagement of close to 1,000 children in a conservation education programme focused on the pygmy hippopotamus. Effective transboundary and protected area law enforcement will be key towards safeguarding and increasing remaining pygmy hippopotamus numbers, whilst awareness raising, collaborative forest management and national/regional policies to reduce deforestation will be needed to secure habitats for pygmy hippopotamus populations to thrive.
It is important to understand that species living in ecosystems that are not conspicuously destroyed may also experience the effects of habitat loss, and hence suffer population declines. This is because habitat loss often manifests itself, at least initially, through less visible but equally threatening habitat degradation. For example, disturbances such as overgrazing do not immediately change the organisation of dominant plants and other structural features of an ecological community. First, barely noticeable, a few sensitive habitat specialists disappear, being unable to cope with high levels of grazing. Soon, invasive species that can tolerate trampling start occupying the niches left open by the extirpated sensitive species. Eventually, when livestock eat the last remaining edible morsels of palatable plants not choked out by invasive species, all that is left of the once productive grassland is a field full of dense, unpalatable, invasive shrubbery.
5.1.1 What is habitat fragmentation?
As governments and industries implement measures to accelerate economic growth, ecosystems that formerly covered large, continuous swathes of land are being increasingly subdivided into smaller parcels by roads, farm fields, towns, and other human constructs. A recent study estimated that roads have divided the African continent into more than 50,000 individual units of land; the median unit size was an alarming 6.75 km2 (Ibisch et al., 2016). This process, known as habitat fragmentation, divides once large and widespread wildlife populations—many already suffering from habitat loss—into several increasingly smaller subpopulations. Habitat fragmentation thereby hastens extinctions, as each of these fragmented subpopulations are more exposed to a range of deleterious genetic effects (Section 8.7) than the previously large and connected population.
Habitat fragmentation creates small and isolated subpopulations that have fewer opportunities to find food, water, shelter, and mates.
As if they are victims of double jeopardy, habitat fragmentation also impedes these smaller subpopulations’ dispersal and colonisation abilities. Most species, especially those that occur in low densities, have large home ranges and/or live in ephemeral habitats, and must be able to move freely across the landscape to find shelter, food, water, and mates. A recent global review found that habitat fragmentation has already reduced the average distance of animal movements by two-thirds—from 22 km to 7 km—over the past few decades (Tucker et al., 2018). If they cannot move freely, these individuals cannot fulfil their needs and are at risk of extinction. Habitat interior specialists are particularly vulnerable to habitat fragmentation, as they are often reluctant to disperse over degraded or cleared areas, even if only a few metres wide (Blake et al., 2008; van der Hoeven et al., 2010). And yet, many habitat specialists face barriers much larger than a few metres. This includes Cameroon’s few remaining drill (Mandrillus leucophaeus, EN) populations, which are facing extinction because individuals are reluctant or unable to disperse over agricultural land that stretches over hundreds of metres (Morgan et al., 2013).
Physical barriers that impede the ability of wildlife to move freely across the landscape also represent a form of habitat fragmentation. Dispersal impeded by human-constructed barriers, such as railways; dams; water-filled ditches; roads; and fences (Figure 5.2), can have disastrous consequences for biodiversity. Consider, for example, Africa’s seasonal drylands. These areas were historically characterised by vast herds of migratory herbivores constantly moving from one area to another after fresh pasture. But as land management systems changed over time, the construction of roads and erection of fences to mark property boundaries impeded the ability of these herds to move freely after the resources they needed to stay alive (Durant et al., 2015; Hopcraft et al., 2015; Stabach et al., 2016). Restricted to only small parts of their range, these once-migratory animals were forced to overgraze the areas they already exploited, leading to extensive population declines. Through this process, Africa has already lost seven mass migrations, each involving millions of animals (Harris et al., 2009). Considering the economic stimulus provided by tourists visiting East Africa’s famous Serengeti-Mara herbivore migration each year, the loss of these seven mass migrations have come at a huge cost to economies elsewhere. Luckily, through diligent conservation efforts, all of Africa’s once-migratory herbivores have managed to persist in small and scattered populations throughout their range (Hoffmann et al., 2015). Section 11.3.1 discusses how some herbivore populations are reverting to their old migration routes after fence removals.
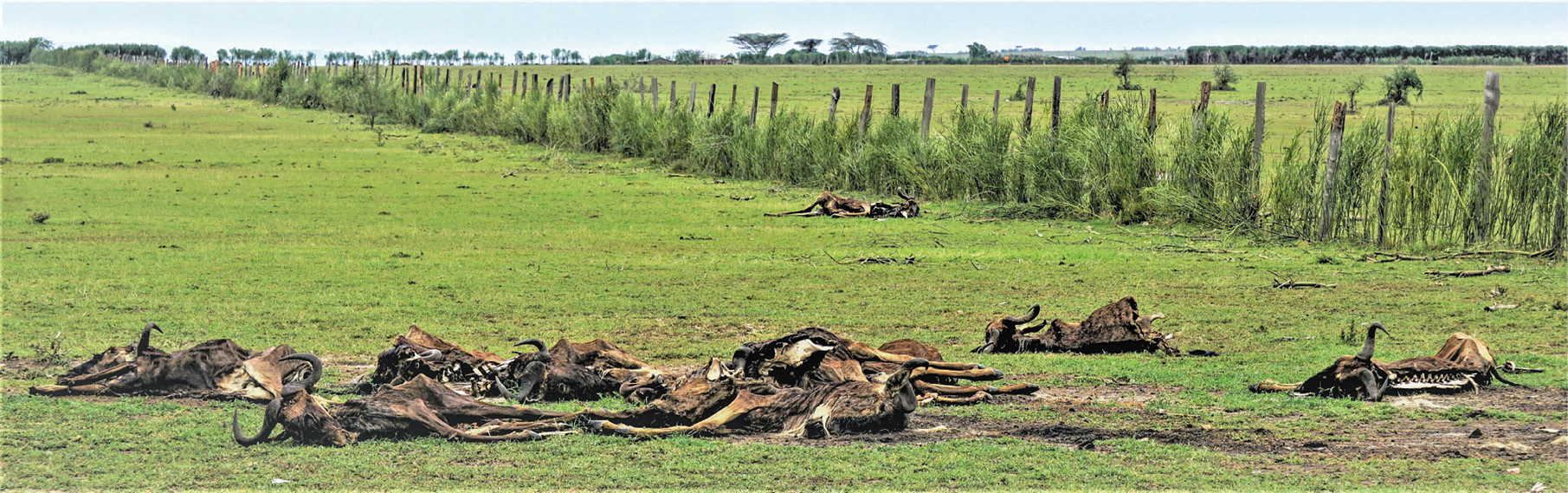
Figure 5.2 Common wildebeest (Connochaetes taurinus, LC) at Kenya’s Maasai Mara that died after a fence stopped them from continuing their migration. Photograph by Teklehaymanot G. Weldemichel, CC BY 4.0.
Habitat loss and habitat fragmentation may even threaten the survival of species that are not as obviously dependent on large-scale movements for survival. As discussed in Section 4.2.5, many plants cannot persist without seed dispersal. Unfortunately, many seed dispersers, including forest primates (Estrada et al., 2017) as well as frugivorous birds, such as parrots, orioles, turacos, and hornbills (Lehouck et al., 2009), are sensitive to habitat fragmentation. In one of the few studies looking at this issue in Africa, researchers found that valuable timber trees in Tanzania’s East Usambara Mountains are being extirpated as forest fragments become too small to support viable populations of fruit-eating birds (Cordeiro et al., 2009). The loss of these important seed dispersers will therefore have knock-on effects on the plants that depend on them for survival. Eventually, if enough seed dispersers, or perhaps even a single keystone species, disappear because of habitat fragmentation, entire ecosystems may eventually collapse.
5.1.2 What are edge effects?
Edge effects are closely associated with, and exacerbate, the negative effects of habitat loss and fragmentation by altering environmental conditions in the habitat interiors. Dense woodlands, thickets, and forests are especially vulnerable to edge effects. Imagine a tropical forest, especially its large trees forming a continuous leafy canopy. These continuous canopies regulate the microclimate of a forest’s understory by blocking sunlight and wind and maintaining humidity during the day, but also trapping heat rising from the forest floor at night. When the forest’s trees are felled, the continuous canopy is fragmented, which in turn compromises the canopy’s ability to regulate the forest’s microclimate. Cleared areas, as well as forested areas directly adjacent to the cleared areas, will consequently be sunnier, warmer, windier, and dryer during the day, and cooler at night; these climatic changes also disturb nutrient cycles and biomass balances (Haddad et al., 2015). All of these changes further reduce the size of the forest patch to be smaller than the remaining canopy might indicate (Figure 5.3) as the new conditions prevent forest specialists such as shade-loving mosses, seedlings of late-successional trees, and humidity-sensitive amphibians from living in forest edges, leaving them with less interior forest habitat for which they must compete. Importantly, these microclimatic changes can penetrate a forest patch over much greater distances than one might expect. For instance, some forest birds in Uganda are sensitive to edge effects as far as 500 m from cleared areas (Dale et al., 2000).
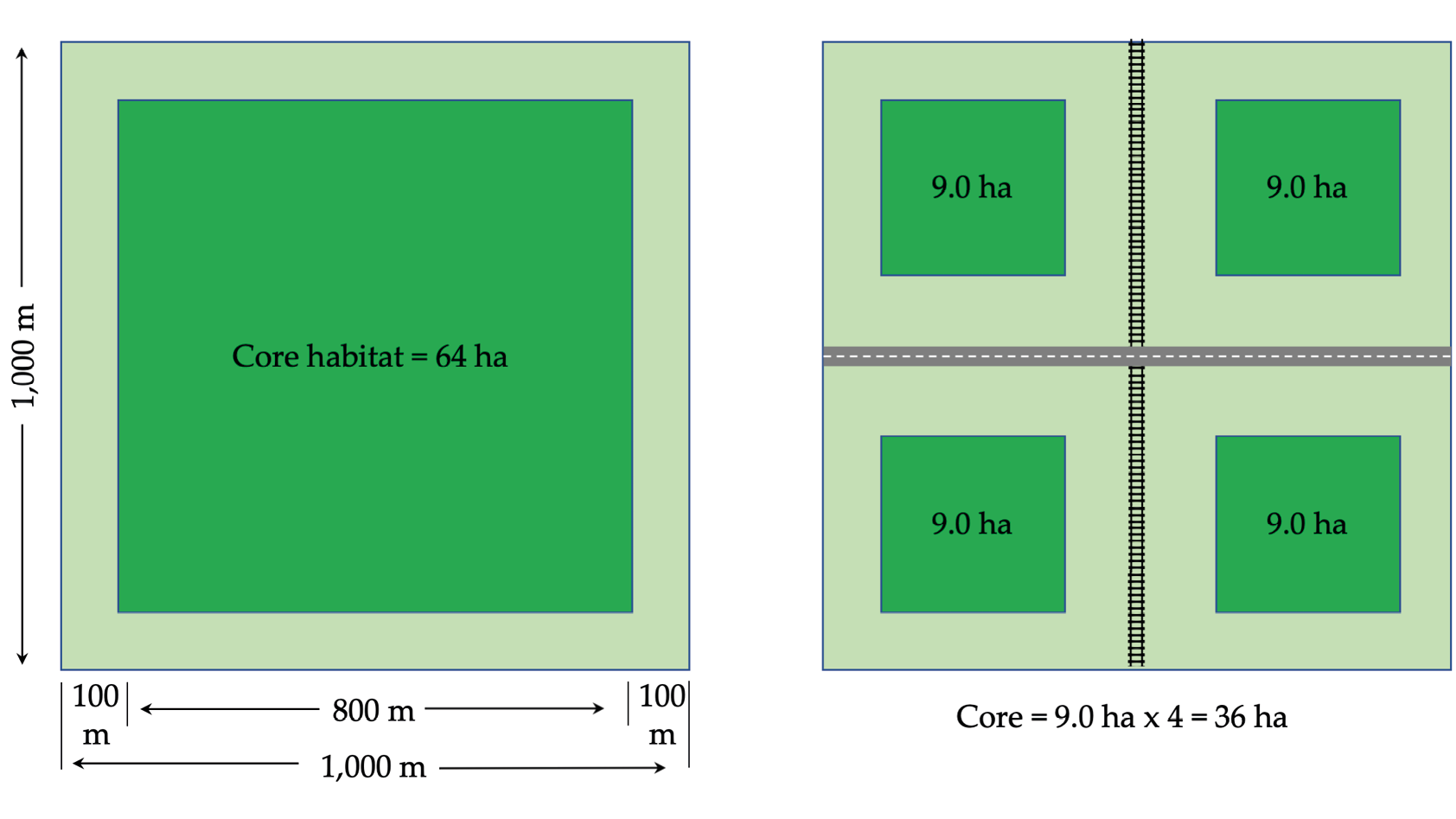
Figure 5.3 An illustration showing how habitat fragmentation and edge effects reduce habitat area. (A) A 100-ha forest patch, where edge effects (grey) penetrate 100 m into the forest: approximately 64 ha of the forest is still core habitat suitable for forest interior species. (B) The same 100-ha forest patch now bisected by a road and a railway. Although the road and railway take up very little area, it increases the patch’s perimeter: area ratio. The resulting edge effects leave more than half of the forest unsuitable for interior species. After Primack, 2012, CC BY 4.0.
Edge effects also create several additional threats to the forest species already suffering from altered microclimates. Notably, disturbed edge conditions present a favourable environment for colonisation by fast growing and fast reproducing invasive species. (Threats posed by invasive species are discussed in more detail in Section 7.4). Those forest species that are not displaced by the altered microclimates and invasive species also face elevated predation risk. That is because trees that have died due to altered edge conditions provide suitable perches with clear views from which predatory birds can hunt (Sedláček et al., 2014). The degraded forest edge, sometimes resembling a savannah structure, also provides opportunities for woodland species such as snakes to enter the forests, pushing the remaining forest species even deeper into the forest (Freedman et al., 2009). For this reason, forest edge communities generally consist of widespread generalist species and invasive species, while specialist species that can hang on are, literally and figuratively, living on the edge.
Edge effects beget further edge effects in a positive feedback loop leading to a rapidly disappearing ecosystem.
The most devastating impact of edge effects is that edge effects beget further edge effects in a positive feedback loop leading to a rapidly disappearing ecosystem. First, expanding invasive (and generalist) species populations at habitat edges can easily overwhelm more sensitive habitat specialists. As habitat specialists are displaced at the contact zones, microclimatic conditions change, which allows for invasions even deeper into the fragmented habitat patch. In this way, invasions systematically penetrate deeper and deeper into the forest as microclimates are disturbed, habitat specialists are displaced, and new contact zones are created. The forest plants that die in the process also increase fuel loads, which, combined with drier and windier edge conditions, create an environment increasingly favourable for fire disturbance. Whether from lightning strikes or human activities, subsequent fires burn hotter and over a larger area (van Wilgen et al., 2007), disturbing and destroying more and more habitat each time. Through these mechanisms, edge effects can degrade entire ecosystems over time, harming both the native species and human livelihoods that depend on those areas.
5.2 Drivers of Habitat Loss and Fragmentation
At present, Africa’s biggest driver of habitat loss is agriculture (Potapov et al., 2017). African farmers have always cleared lands to meet their subsistence needs. Much of this clearing was traditionally and historically done in the form of slash-and-burn agriculture (also called shifting cultivation, Figure 5.4). To prepare land for crops, smallholder farmers would first cut down trees to clear the land and to obtain fuel wood. The remaining vegetation would then be burned away to release carbon and other nutrients, which increases land fertility. Farmers would grow crops on these cleared areas for two or three seasons. Then soil fertility would diminish, crop production would decline, and the farmers would abandon the area and clear new land, giving the natural ecosystem on the abandoned land time to regenerate.
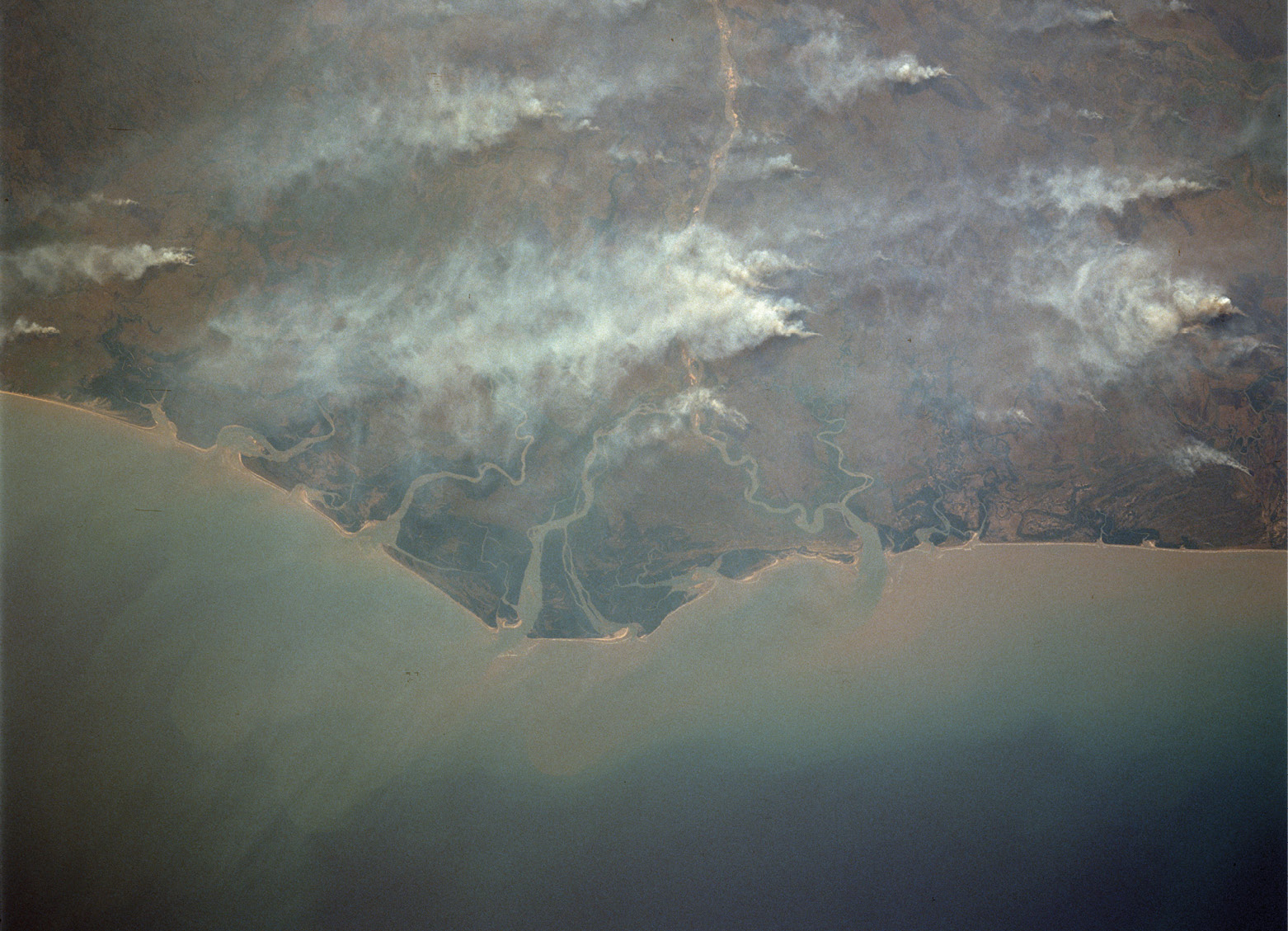
Figure 5.4 On a cloudless day, multiple fires raging in Mozambique’s Zambezi River delta region can be seen from the International Space Station. Slash-and-burn techniques are often used to clear natural ecosystems for grazing and crops. Overly frequent fires, however, do not allow for ecosystem recovery, and are devastating to fire-sensitive ecosystems, such as tropical forests; instead of recovery, every fire creeps deeper and deeper into the forest until the entire ecosystem has been degraded. Image by NASA, https://commons.wikimedia.org/wiki/File:Zambezi_delta.jpg, CC0.
Medical and technological advances, and the arrival of colonists, saw Africa’s human population grow considerably since the 1800s. Feeding and accommodating the activities of this growing human population saw an increasing number of natural ecosystems replaced by agricultural land, and less area given the time to regenerate. An increasing number of people also started abandoning their rural subsistence lifestyles for cities in search of jobs, financial freedom, and an easier life. As urbanisation increased (i.e. more people moved to cities) and competition for jobs intensified, an increasing number of city dwellers became dependent on collecting charcoal for cooking and cultivating cash crops, such as yams and cassava (Rudel, 2013). This saw even more natural ecosystems converted, particularly on the outskirts of cities. In the meantime, the remaining rural population became increasingly sedentary due to changing land tenure systems, which forced them into unsustainable farming practices as competition for land increased. These factors not only increased rates of habitat loss, but also changed the nutrient content in the soil which, in turn, reduce the land’s ability to regenerate and to produce food (Drechsel et al., 2001; Wallenfang et al., 2015) which, in turn, leads to even more land clearing for agriculture.
While land clearing for smallholder agricultural needs continues to be an important driver of habitat loss (Tyukavina et al., 2018), its impact is increasingly dwarfed by the demands of commercial interests (Austin et al., 2017). The impact of land grabbing is of particular concern. Foreign companies from Asia and other parts of the world have acquired millions of hectares of land across Africa to stake a claim on the continent’s rich natural resources, and to produce food and biofuels for their own people (von Braun and Meinzen-Dick, 2009). The foreign stakeholders, who often strike these land deals through loan agreements at the governmental level (i.e. with little to no local input), typically prioritise their own needs and profits over local interests with little care for the environment. These deals thus often end with a country saddled with debt they struggle to repay, and environmental damage that will take generations to reverse. Moreover, the foreign companies often employ migrant labourers with fewer protections and rights, compared to local peoples. In the process, while a modest number of local people may benefit from job creation, technology investment, and infrastructure development, a large number of local people become disenfranchised and displaced from the lands that previously supported their livelihoods. These foreign investments are a type of neocolonialism for their resemblance to Africa’s earlier colonial era. They not only drive large-scale habitat loss, but in many instances also leave local people impoverished and desolate (Koohafkan et al., 2011).
The impacts of land clearing for smallholder farms are increasingly dwarfed by the outsized demands of commercial interests.
To understand the impact of land grabbing on Africa’s natural environment, one simply needs to consider their scale. For example, Chinese bioenergy producers recently secured over 48,000 km2 of land in the DRC and Zambia (Smaller et al., 2012). Another deal, between the Ethiopian government and companies from India and Saudi Arabia, saw 5,000 km2 of land (including sections of Gambella National Park) earmarked for commercial agriculture. At the time, this Ethiopian deal threatened both the second largest mammal migration on Earth (Ykhanbai et al., 2014) and the livelihoods of the local pastoralist Anuak community (Abbink, 2011). Fortunately, the Ethiopian government and developers were responsive to concerns raised by conservationists and human rights advocates, and agreed to set some areas aside for conservation, while also putting measures in place to maintain free movement of animals and pastoralists.
Infrastructure developments are also becoming an important driver of habitat loss. Offering access to previously unexploited areas, roads are perhaps the single biggest driver of habitat loss facing Africa’s last remaining wildernesses (Figure 5.5). As prominent tropical biologist Bill Laurance eloquently noted, “Roads usually open a Pandora’s Box of environmental problems—such as illegal fires, deforestation, overhunting and gold mining” (Laurance et al., 2014). A vast, growing body of literature from Africa supports these claims. For instance, research in the Congo Basin has shown how deforestation generally occurs within 2 km from roads (Mertens and Lambin, 1997)—more roads thus mean more deforestation. Roads also facilitate other drivers of forest loss, including the spread of invasive species, human settlements, fire, and pollution (Kalwij et al., 2008; Potapov et al., 2017). Providing access points for hunters, roads also facilitate unsustainable hunting; a recent review found that the wildlife reductions due to hunting could be detected as far as 40 km from the nearest road (Benítez-López et al., 2017).
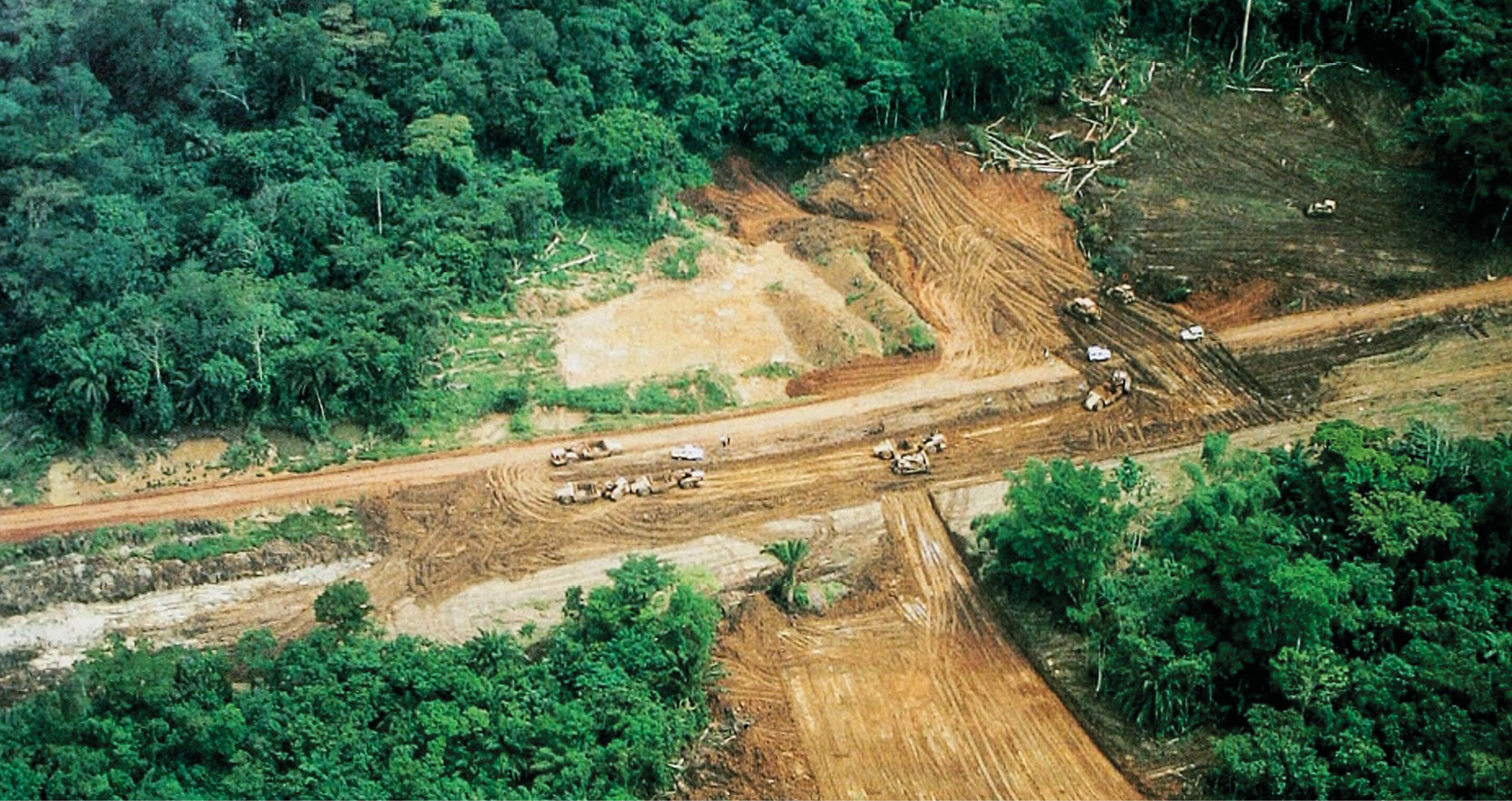
Figure 5.5 New road developments, such as this one in the Congo Basin, represent one of the most immediate threats to biodiversity conservation. Road development provides access to previously unexploited areas, allowing more areas to be hunted, logged, farmed, and settled; increased human activity also exposes these areas to invasive species and pollution. Photograph by Charles Doumenge, https://www.flickr.com/photos/internetarchivebookimages/20689353531, CC0.
5.3 Habitat Loss’ Impact on Africa’s Ecosystems
5.3.1 Tropical forests
Occupying about 7% of all land surfaces, tropical forests are estimated to contain over 50% of the world’s terrestrial species (Corlett and Primack, 2010). Due to these high levels of biodiversity, the complexity of biological interactions in tropical forests is unparalleled in other ecosystems, and consequently also their importance to humans. On a local scale, the timber and non-timber products from tropical forests sustain the traditions (Box 5.2), livelihoods, and financial well-being of millions of Africans. Tropical forests also have regional importance including protecting catchment areas (Section 4.2.4) and moderating climate (Section 4.2.3). Lastly, as reservoirs of carbon, tropical forests play a globally important role in mitigating the negative effects of anthropogenic climate change (Section 10.4), and with 17% of Earth’s tropical forests, Africa plays a globally important role in tropical forest conservation efforts.
Box 5.2 The Conservation and Exploitation of East African Plants
College of Agricultural and Environmental Sciences, Makerere University,
Kampala, Uganda.
jtabuti@caes.mak.ac.ug
Ethnobotany, as a scientific discipline, studies the relationships between people and plants: how people affect the survival and distribution of plants, and how plants influence human behaviour and cultures. For instance, local cuisines are shaped by available plant species, and people cultivate species that they consider useful. Conservation of plant diversity can be aided in many ways by recognising the importance of plants to people’s livelihood and spiritual practices.
The people of East Africa identify and use a great many plant species that are essential for their well-being (Tabuti, 2006). Native plants are used for food, for construction, to treat the diseases of both people and livestock, and in numerous other ways. Some of the most important species include White‘s ginger (Mondia whitei) and red stinkwood (Prunus africana, VU) for medicine, African teak (Milicia excels, NT) for timber, shea tree (Vitellaria paradoxa, VU) for food and cosmetics, and African sandalwood (Osyris lanceolate, LC) as a source of fragrant oil.
Some plant species (and sometimes entire ecosystems, such as forests) are valued for religious or cultural reasons. The plants or forest areas themselves are considered sacred, the site of a deity or spirit, with certain rituals performed using those special plant species or the habitats they occupy. These sacred sites and species are protected by local taboos. For example, the powderbark gardenia (Gardenia ternifolia) is not harvested for firewood among the Balamogi people of Uganda because it is believed to bring bad luck. Among the Mijikenda people of Kenya, sacred forests known as Kaya are protected because people believe that the forests are inhabited by spirits and are places of prayer and held as a source of ritual power. Cutting down trees, grazing livestock, and farming are prohibited within the Kaya. One protective belief holds that cutting a tree in the Kaya with a machete can result in the machete rebounding and causing injury to the woodcutter. Another belief is that food cooked using wood from these sacred forests can cause sickness, and that a dwelling built with timber drawn from the forest will collapse. Consequently, more than 50 Kaya—ranging in size from 0.3 to 3 km2 and home to 187 plants, 48 birds, and 45 butterfly species—have enjoyed unofficial protection due to religious and cultural beliefs.
Today, however, the plants and their natural communities on which people rely for their well-being are being threatened. By far the greatest threat is land use change and habitat conversion to agriculture to grow food for a growing population. Changing cultural and spiritual values in East Africa, as well as social and economic pressures, are threatening the existence of even sacred forests. For instance, the coronation site of the Paramount Chief of the Balamogi in Uganda was previously protected as a sacred forest by local lore, but it has now been cut down and converted into gardens by local people who no longer follow ancient traditions. Harvesting of plant species, such as the red stinkwood and East African sandalwood, for international markets is also a significant threat no longer held at bay by cultural norms.
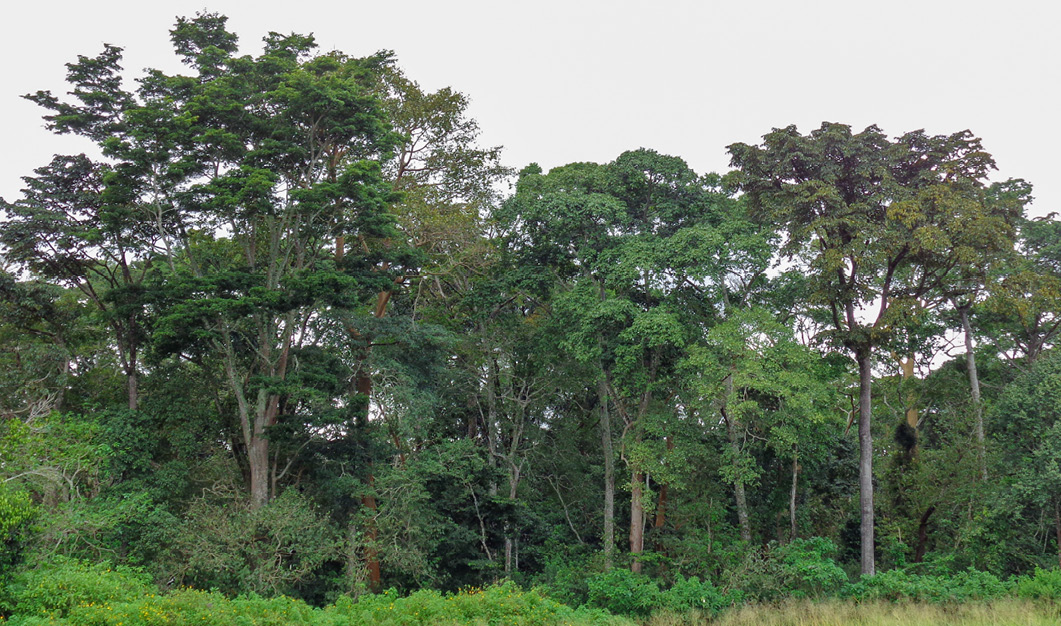
Figure 5.B The edge of the Budongo Forest Reserve, Uganda, where researchers collaborate with local communities to refine methods for the sustainable utilisation of tropical plant products. Photograph by John Tabuti, CC BY 4.0.
Thankfully, several species continue to be actively protected by local communities and governments. According to Greger (2012), traditional healers aid conservation by replanting around 50% of the medicinal plant species that they consider to be important to their practice. For the relationship between people and plants to survive, scientific conservation and local tradition must work together. An example of such collaboration is on display in Uganda’s Budongo Forest Reserve (Figure 5.B), where researchers at the Budongo Conservation Field Station are working with local communities to refine methods for sustainable management and utilisation of the region’s local plants.
Despite the importance of tropical forests, their destruction has become synonymous with the rapid loss of biodiversity (Figure 5.6). Africa had already lost over 65% of its original tropical forests by 1990 (Sayer, 1992); human activities destroyed an additional 308,000 km2 (an area larger than Italy) between 1990 and 2010 (Achard et al., 2014). Losses were particularly severe in Burundi, Benin, and Mozambique, with each country holding less than 5% of its original forest cover (Sayer, 1992). Retaining about half of its original forest cover, the DRC is relatively better off, but current deforestation rates in this country are currently second highest globally (Weisse and Goldman, 2019). Current deforestation rates are so severe in Equatorial Guinea that this country will lose all its forests within the next 20 years if current trends hold (Potapov et al., 2017). Despite these alarming trends—the destruction continues nonstop, particularly in Ghana and Côte d’Ivoire, which saw a 60% and 26% rise in forest loss (the highest rise globally), respectively, between 2017 and 2018 (Weisse and Goldman, 2019). Across Africa, logging is currently the dominant driver of tropical forest loss (causing 77% of total losses over the past decade), followed by agriculture (Potapov et al., 2017).
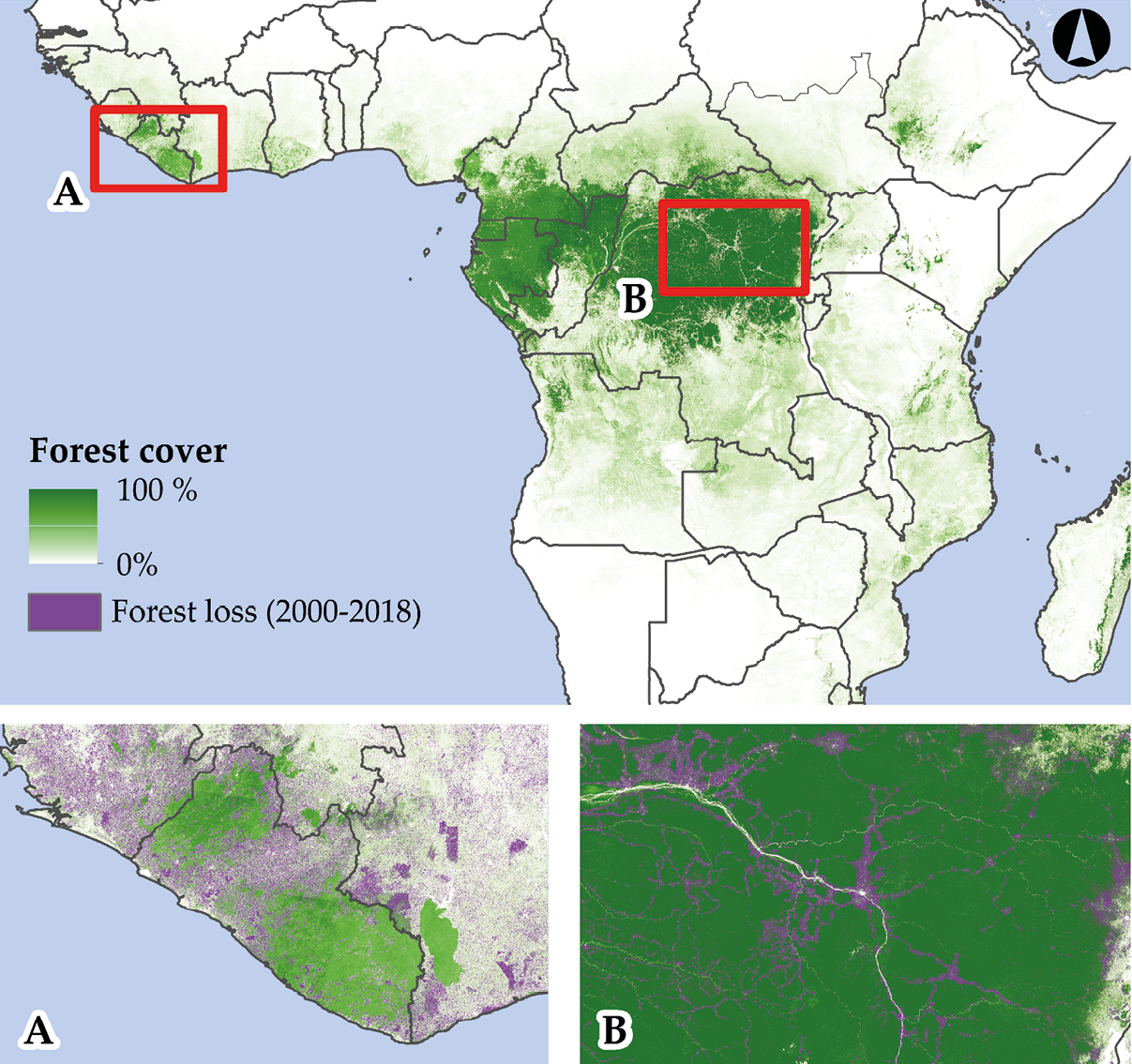
Figure 5.6 The extent of Sub-Saharan Africa’s tropical forests in 2018, and the extent of tropical forests loss (A) around Liberia and (B) in the north-eastern part of the Congo Basin between 2000 and 2018. Note in (A) how deforestation follows country borders, and in (B) how deforestation follows road networks. Source: Hansen et al., 2013. Map by Johnny Wilson, CC BY 4.0.
5.3.2 Rivers and deltas
Due to our dependence on freshwater, humans have always preferred to live near rivers, streams, and lakes. Consequently, these aquatic environments have been destroyed at a scale at least equal to that of terrestrial environments. Rivers have taken a particularly hard hit from human activities, being polluted by industries and dammed to ensure a reliable, year-round supply of water for consumption and irrigation, and to generate hydroelectricity.
Dam construction holds several negative consequences for biodiversity and people. Aquatic organisms that cannot survive the altered river conditions downstream (reduced flow and dissolved oxygen, higher temperatures, and increased turbidity) are most vulnerable. For example, a study from South Africa found that native macroinvertebrate populations (often a good indicator of water quality) were reduced by 50%, and some insect orders virtually extirpated following dam construction (Bredenhand and Samways, 2009). Dams also displace aquatic organisms upsteam. In one well-studied example, back flooding of Mozambique’s Massingir Dam facilitated river substrate changes and the spread of invasive species, which in turn forced sharptooth catfish (Clarias gariepinus, LC), tiger fish (Hydrocynus vittatus, LC), and Nile crocodiles (Crocodylus niloticus, LC) to change their diet. Increased stress levels due to these dietary and environmental changes leave the affected animals susceptible to pansteatitis (a condition where body fat becomes inflamed), leading to mass wildlife mortality events in South Africa’s Kruger National Park (Woodborne et al., 2012). Lastly, dams reduce connectivity in freshwater ecosystems, preventing freshwater organisms from exchanging genetic material, migrating between upsteam and downstream areas, and adapting to changing conditions. For example, in West Africa, the damming of the Senegal River blocked the annual migration path for African river prawns (Macrobrachium vollenhoveni, LC), a major predator of snails which host schistosomiasis (bilharzia). Once the dam was completed, prawn populations collapsed, leading to a schistosomiasis epidemic in villages upstream from the dam (Sokolow et al., 2015).
Damming rivers harms biodiversity and people both upsteam and downstream from these developments.
Terrestrial ecosystems also suffer from dam construction. Of concern is the direct loss of riverine and palustrine ecosystems downstream from the dam due to reduced waterflow. For example, construction of Nigeria’s Kainji Dam in the Niger River caused the drying of large wetlands and floodplains downstream, in the process displacing nearly 400,000 people who depended on the river’s now-compromised seasonal flood cycles (Drijver and Marchand, 1985). Flooding of upland areas next to dammed rivers also displaces terrestrial wildlife and people. For example, construction of Mali’s Manalati Dam flooded 430 km2 of savannah and 120 km2 of forest, which fractured the migration routes of the region’s nomadic pastoralists, leading to overgrazing and soil erosion of the remaining grazing lands (deGeorges and Reilly, 2006), in addition to a 90% loss of fisheries downstream (Acreman, 1996).
5.3.3 Wetlands
Throughout Africa, wetlands are being mined for valuable peat, or drained and/or filled in for development and agriculture. Through these activities, the region has already lost approximately 43% of its wetlands, with current rates of loss among the highest in the world (Davidson, 2014). This is a major concern because wetlands serve as spawning grounds and nurseries for aquatic and amphibious wildlife and stop-over sites for migratory birds (Box 5.3). Wetlands also provide multiple important ecosystem services. For example, they prevent erosion and runoff by capturing large volumes of floodwater, which is then released slowly over time. This process also allows sediments and nutrients kicked up during flood events to settle out, creating fertile habitats for a wide diversity of animals and plants, as well as for agriculture. Water that leaves after this settling period is cleaner than when it entered, having been filtered by the soil, plants, and microbes of wetlands. This water purification and filtration service is generally cheaper and much more efficient than man-made filtrations systems. The loss of any wetlands, but especially at such large scales, is thus a grave concern not only because of the countless animals and plants threatened with extinction, but also the people that depend on all the valuable ecosystem services they offer.
Box 5.3 Migratory Birds of Africa: The Largest of the Last Great Migrations?
1Acadia National Park, US National Park Service,
Bar Harbor, ME, USA.
How are Africa’s bird migrations, the biggest in the world, faring in a rapidly changing world? Each year, about 2.1–5 billion birds (mostly songbirds, but also raptors, waterbirds, and many others) travel back and forth between their wintering grounds in Africa and breeding grounds in Europe and Asia (Figure 5.C). Of the 126 species involved in this migration, over 40% have continuously decreased in abundance since 1970 (Vickery et al., 2014). At first, populations that overwintered in open dry savannahs declined: examples include the Ortolan bunting (Emberiza hortulana, LC) and European turtle dove (Streptopelia turtur, VU) which decreased by 84% and 69% between 1980 and 2009, respectively. More recently, species overwintering in the humid Afrotropics also started declining: this includes songbirds, such as the common nightingale (Luscinia megarhynchos, LC) and river warbler (Locustella fluviatilis, LC)—populations of both declined by 63%—and waterbirds such as the black-tailed godwit (Limosa limosa, NT), which declined by 45%.
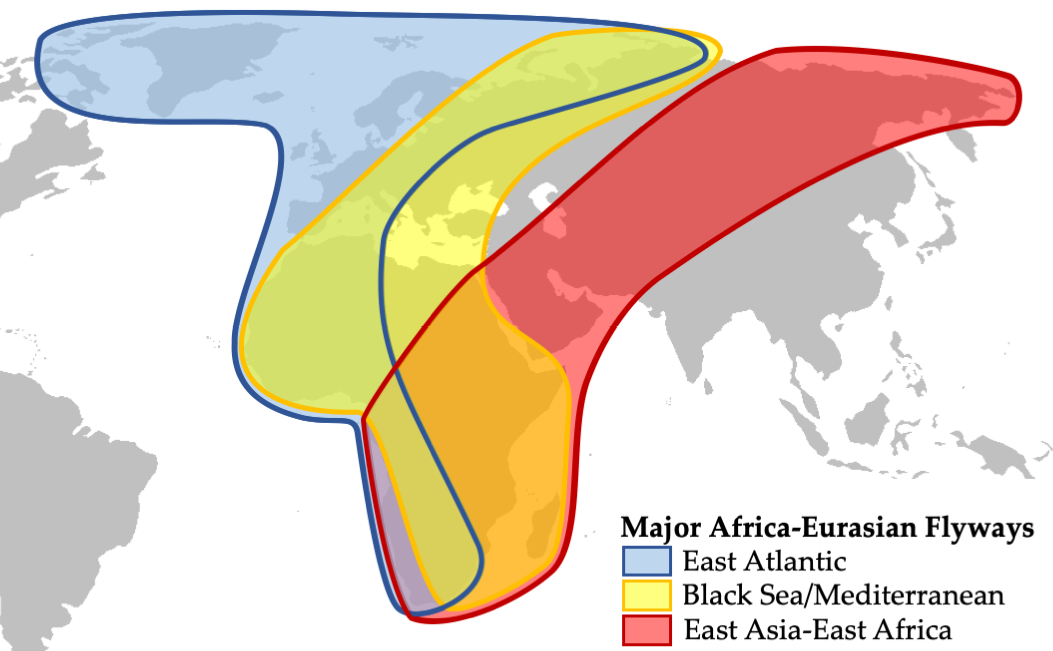
Figure 5.C The three major migratory flyways that African birds use to travel back and forth between their wintering grounds in Africa and breeding grounds in Europe and Asia each year. After BirdLife International, 2019, CC BY 4.0.
To survive their long journeys, migratory birds need favourable weather conditions, adequate food sources, and intact habitat not only at the end points where they breed or overwinter, but also along their routes where the migratory animals can rest and refuel (Runge et al., 2015). Disturbances in any of these places can lead to sharp population declines. For example, recent research showed that the habitat quality of a single stop-over site can determine whether a migration is successful or not (Gómez et al., 2017). Illustrating this point, a drought in the Sahel, an important migratory stop-over site, led to food shortages that killed 77% of the world’s common whitethroats (Sylvia communis, LC); even today, this population has not yet fully recovered (Vickery et al., 2014).
Human activities have greatly contributed to the declines of Africa’s migratory birds (Kirby et al., 2008; Vickery et al., 2014). For example, each year thousands of hectares of wetlands, forests, grasslands, and savannahs are being converted into farmlands and urban areas or polluted by rampant use of pesticides and herbicides. Migratory birds also need to deal with hunters and trappers, and an increasing number of human-made structures, such as high-rise buildings, wind turbines, and power lines that represent collision and electrocution hazards (e.g. Rushworth et al., 2014). Then there is the threat of inconsistent rainfall, which causes food shortages and direct mortality, and climate change, which causes temporal mismatches between migratory movements and abundance of key food resources (Both et al., 2006; Vickery et al., 2014).
Addressing these declines, governments, conservation organisations, and local communities all over Africa have started initiatives to protect migratory birds and their habitats. One such initiative is happening in Kenya’s Tana River Delta, one of the most important stop-over sites along the Asian-East African Flyway. Every year, Basra reed warblers (Acrocephalus griseldis, EN) return from their Middle Eastern breeding grounds to overwinter in the Delta, which covers 1,300 km2 and supports dozens of threatened species. The area, however, has been under serious threat from development for sugarcane and biofuel crops since 2008. These activities could reduce dry season water flow by up to one third. Local people and conservationists strongly oppose these developments because of its threat to local communities’ ways of life and to wildlife populations. Their efforts gained international attention, and in 2012, Kenyan courts halted development until comprehensive management plans were developed that included environmental impact assessments and local stakeholder engagement (Neville, 2015). Today, local people gain benefit from more sustainable industries, including eco-charcoal audited by the Forest Stewardship Council (FSC), and solar-powered energy to reduce the need for wood.
Also, in West Africa, collaborative conservation initiatives are taking steps to protect the critical East Atlantic Flyway. For example, under the guidance of BirdLife International, Guinea-Bissau residents are now monitoring several wetlands in the Bijagós Archipelago to track how well migratory waterbirds are doing at this critically important stop-over site. Also, in Senegal, where two important stop-over sites (Saloum Delta and Djoudj wetlands) are located, the local non-profit NGO Nature Communautés Développement initiated an extensive conservation education programme aimed at safeguarding the region’s birds.
Conserving migratory species that cover huge distances and rely on habitats in many areas is not easy. However, efforts like these in West Africa and Kenya (which combine the interests of local people and wildlife) provide excellent models for others to build from.
Mangrove swamps (sometimes called mangrove forests, though technically a wetland because their function and structure are primarily determined by hydrology, Lewis, 2005; Gopal, 2013) are one of Africa’s most threatened wetland ecosystems. Characterised by woody plants that can tolerate saltwater, mangrove swamps occupy brackish waters in tropical coastal areas, typically where there are muddy bottoms. These areas are sparsely distributed; globally, mangrove swamps cover only 53,000 km2 of land scattered across 118 countries (Dybas, 2015). Protecting Africa’s mangrove swamps, comprising 21% of Earth’s total, is important both biologically and economically. In addition to holding many unique species, mangrove swamps also protect coastal cities and villages from cyclone/hurricane and tsunami damage and provide important breeding and feeding grounds for marine shellfish and fish. One study estimated that mangrove swamps provide an estimated US $57,000 worth of ecosystem services per hectare (van Bochove et al., 2014). Yet, only 7% of Africa’s mangrove swamps are protected. With so little protection, it comes as no surprise that a large percentage of Africa’s mangrove swamps have been destroyed or damaged by agriculture, urban expansion, pollution, and commercial shellfish farming (Giri et al., 2011). In West Africa, the situation is particularly dire. Wood extraction for commercial fish smoking is one of the biggest drivers of mangrove losses, even within protected areas (Feka et al., 2009). With so much destruction, it should come as little surprise that about 40% of vertebrate species endemic to mangrove swamps are currently threatened with extinction (Luther and Greenberg, 2009).
Mangrove losses around Africa have been extensive despite them providing an estimated US $57,000 worth of ecosystem services per hectare.
5.3.4 Seasonal drylands
Africa is also rapidly losing its semi-arid savannahs, scrublands, and grasslands through conversion to agriculture (Box 5.4) and desertification—the systematic degradation of formerly complex and adaptive seasonal drylands into barren wastelands (Figure 5.7). When human populations were low, nomadic pastoralism and shifting cultivation enabled people to utilise seasonal drylands in a sustainable way. Today however, population growth, combined with restrictions placed on free movement by administrative borders and competition for land, forces people and animals living on drylands to be more sedentary. While these areas may initially support some agriculture and livestock, unsustainable techniques, such as overgrazing and excessive tilling, lead to soil erosion and the depletion of soil nutrients and natural seed banks. With the cover vegetation gone, the unprotected topsoil is easily lost to wind and flooding, leaving behind the deeper, infertile, and compact subsoil layers with little capacity tfo hold water. The result is something that closely resembles a man-made desert. However, rather than a functional ecosystem characterised by species adapted to life in the desert, these wastelands have lost their original productivity and biological communities, only to be revived through expensive and/or time-consuming land reclamation methods.
Africa is rapidly losing semi-arid ecosystems due to desertification, the conversion of productive ecosystems into barren wastelands.
Box 5.4 Saving Critically Endangered Ground Nesting Birds from Habitat Loss
1Biology Department, Kotebe Metropolitan University,
Addis Ababa, Ethiopia.
2Edge of Existence Fellow, Zoological Society of London,
London, UK.
brukabdu.m@gmail.com
We are currently witnessing the start of the sixth mass extinction of species on our planet. From here onwards, biodiversity losses are expected to increase rapidly: a recent UN report estimated that about one million species are already threatened with extinction (IBPES, 2019). While the reasons behind these losses vary by region, in Africa, a major driver is habitat loss. With the current push for development, the impacts of habitat loss are increasing dramatically, affecting species both inside and outside of protected areas. Two Ethiopian birds (Figure 5.D), the Liben lark (Heteromirafra archeri, CR) and white-winged flufftail (Sarothrura ayresi, CR), exemplify many of the dilemmas associated with protecting biodiversity on unprotected lands where habitat loss is severe.
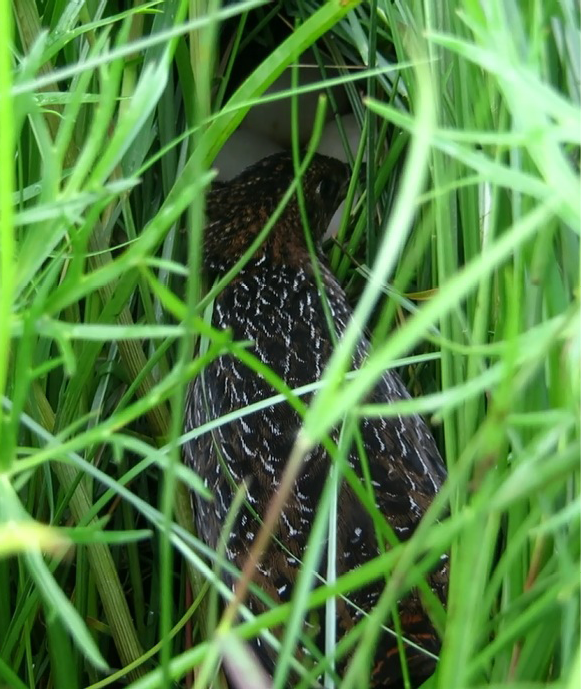
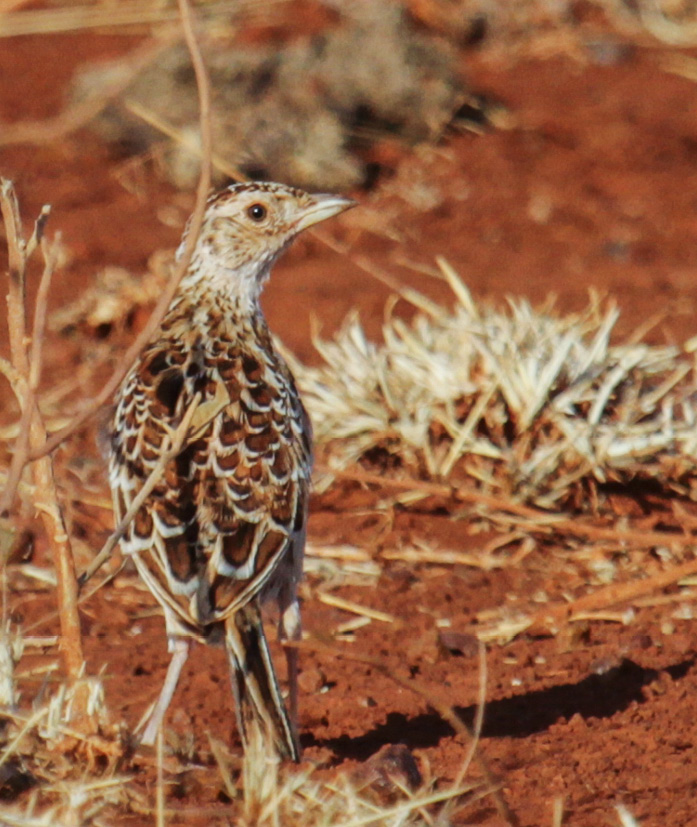
Figure 5.D (Top) A white-winged flufftail, one of Africa’s most enigmatic birds, standing defensively in front of its nest (eggs can be seen in the background) in the flooded grassland on the Berga floodplain, Ethiopia. Photograph by Bruktawit Abdu Mahamued, CC BY 4.0. (Bottom) A Liben lark on its last remaining stronghold in the world, Ethiopia’s Liben Plain. Photograph by Tommy P. Pedersen, CC BY 4.0.
The Liben Plain is part of the Borana rangelands, managed by Borana pastoralists under their traditional rangeland management system which is generally compatible with conservation ideals. The Borana’s way of life was disrupted about 40 years ago due to pressure from a former Ethiopian government who wanted the Boranas to adopt a more sedentary lifestyle. For example, drilling of water wells in dry season grazing areas disrupted seasonal grazing systems, while fires that the Boranas used to maintain productive grazing lands and prevent shrub encroachment were prohibited. The Boranas also face pressure from changing land tenure systems. The Liben Plain grasslands are located on communal lands upon which nobody can claim ownership. However, if someone wants to farm here, they just pay a tax that in effect assures ownership of the land. The Boranas were initially slow to adopt this farming lifestyle, but when outside settlers started taking advantage of the government’s farming incentives, the Boranas were pushed to do the same to prevent all their ancestral land from being turned over (Mahamued, 2016). The subsequent loss of fire management (and associated shrub encroachment) and cropland expansion, together with increased human and livestock populations, have led to a major loss of the Liben Plains’ natural ecosystem.
The Liben lark is a ground-nesting bird that is near-endemic to Ethiopia (a second population in Somalia may already be extinct; Spottiswoode et al., 2013). Here, its main population is restricted to the open grasslands of the Liben Plain. Although it was previously common in this ecosystem, habitat loss and degradation have reduced the availability of suitable feeding and nesting sites. Further, the reduced population is also increasingly vulnerable to direct threats such as nest predation and trampling of nests by cattle (Spottiswoode et al., 2009). Due to these threats, the lark’s numbers have decreased so dramatically in recent years that it was classified as Critically Endangered in 2009.
To prevent the extinction of the lark, the Ethiopian Wildlife and Natural History Society (EWNHS), BirdLife International, and other organizations collaborated with local authorities and community leaders in 2016 to establish enclosures for grassland regeneration. These enclosures are in effect communally-managed grassland reserves regulated under a subset of customary laws. These areas not only secure suitable habitats for the Liben lark, they also provide benefits to the Borana community like securing grazing lands for the dry season when the lark is not breeding. This initiative shows early promise—over 350 ha of grassland reserves have already been established, and over 1,000 ha of shrub have been cleared (Kariuki and Ndang’ang’a, 2018). But to truly secure the future of the Liben lark, more support is needed from the Ethiopian government, particularly in preventing further land conversion, supporting ecosystem restoration, and encouraging the Borana pastoralists’ traditional way of life.
Another species facing imminent extinction due to habitat loss is the white-winged flufftail. One of Africa’s most enigmatic birds, the flufftail is an intra-African migrant restricted to a few seasonal high-altitude wetlands in South Africa and Ethiopia. Like the lark, the flufftail is a ground-nester that struggles to find suitable nesting sites relatively free from disturbance. The Berga floodplain, the flufftail’s Ethiopian stronghold, used to be covered by productive grasslands. This unspoiled landscape is now being replaced by settlements, crop farms, and eucalyptus plantations that generate quick profits. This, together with overgrazing, has led to extensive soil erosion, which in turn has altered the structure and grass composition of the floodplain. Today, the floodplain is encroached by invasive weeds and other less desirable vegetation (seen during EDGE project surveys in 2018) which, together with others forms of disturbance, have reduced the amount of suitable habitat available for the flufftail to such an extent that it is now considered Critically Endangered.
To prevent the extinction of the flufftail, the EWNHS along with the Middlepunt Trust and BirdLife South Africa have taken several steps to improve the outlook for the flufftail. Much of this work involved working with the people at Berga to improve their livelihoods, and to instil a sense of ownership of their local biodiversity. A prominent outcome of this collaboration was a primary school named after the flufftail; results from the project also contributed to a species action plan (Sande et al., 2008). But without continued maintenance, the progress made by this short-term initiative will have limited long-term value. The flufftail’s future thus continues to be dire, as unsustainable land use practices continue to destroy the Berga floodplain. There is an urgent need for joint long-term efforts to reverse the fate of the species, including taking steps to establish protected areas, to initiate carefully-planned ecosystem restoration efforts, and to develop a new species management plan that will provide lasting benefits.
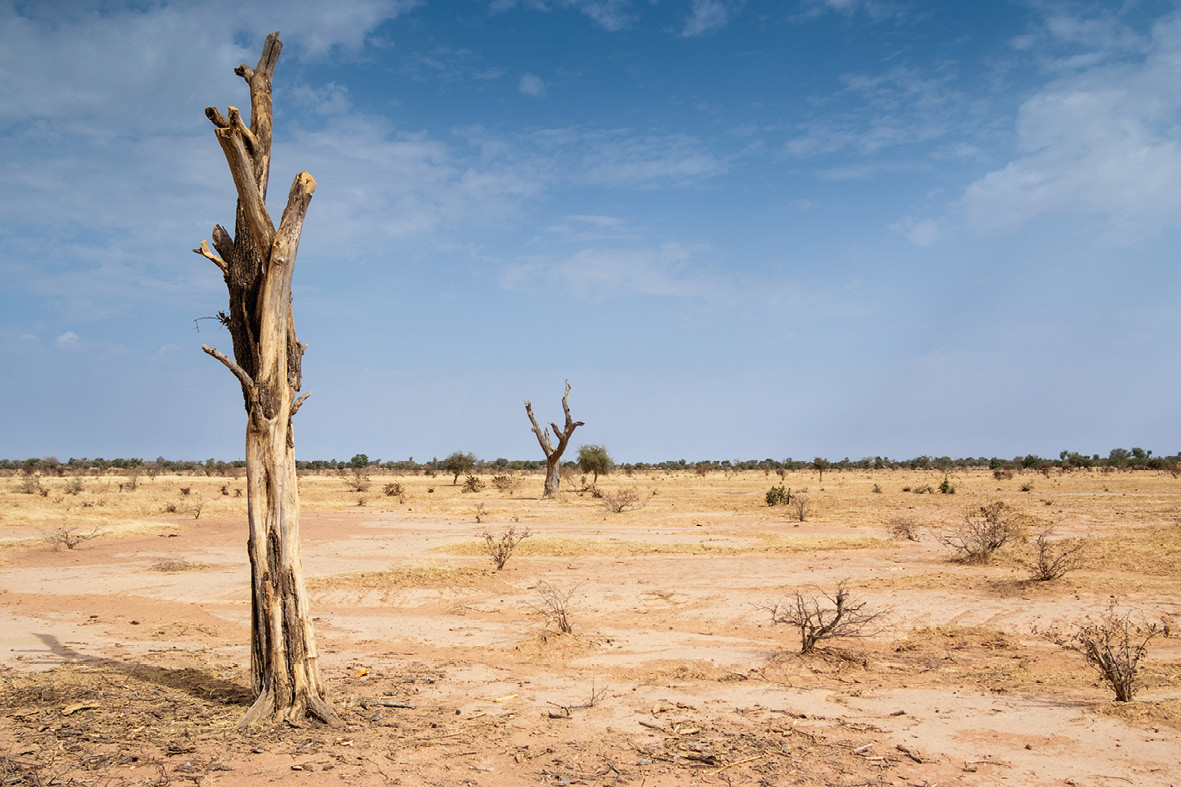
Figure 5.7 Desertification, the degradation of formerly complex and adaptive seasonal drylands into barren wastelands, is a growing threat to Africa’s natural environment, its wildlife, and its people. It is a prominent problem in the Sahel region, such as the area pictured, in Burkina Faso. Photograph by Jose Navarro, https://www.flickr.com/photos/53871588@N05/5630241115, CC BY 4.0.
5.4 Population Growth and Consumption?
Until about 150 years ago, the rate of human population growth in Africa had been relatively slow, with the birth rate only slightly exceeding the death rate. Modern medical achievements and more reliable food supplies have changed this balance; they have reduced mortality rates while birth rates remain high. Consequently, Sub-Saharan Africa’s human population has exploded to 1 billion people over the past decade (World Bank, 2019). Today, Sub-Saharan Africa is leading the world in human population growth, projected to increase by four-fold over the next century. Population growth rates for individual countries are similar, if not higher. For example, Ethiopia’s human population has grown from 48 million in 1990—when the region experienced a famine crisis—to nearly 100 million in 2015; current projections forecast a population of 172 million by 2050. The human population of Tanzania’s Dar es Salaam, a coastal city particularly vulnerable to sea level rise (Section 6.3.2), is expected to increase from 4 million to 21 million between 2015 and 2050, while Lagos in Nigeria is expected to grow from 21 million to 39 million people over the same time.
Simple math suggests that more people leads to less space for biodiversity (Figure 5.8), because humans and wildlife compete for the same resources, broadly speaking. With many countries in Africa already facing social, economic, and developmental challenges such as malnutrition, crime, and unemployment, one can almost understand why politicians prioritise socio-economic upliftment over biodiversity conservation. This is a grave mistake; as discussed in Chapter 4, biodiversity and human well-being are intricately linked. It is one of conservation biologists’ most important tasks: to make the link between conservation and human welfare clear to policy scholars and politicians.
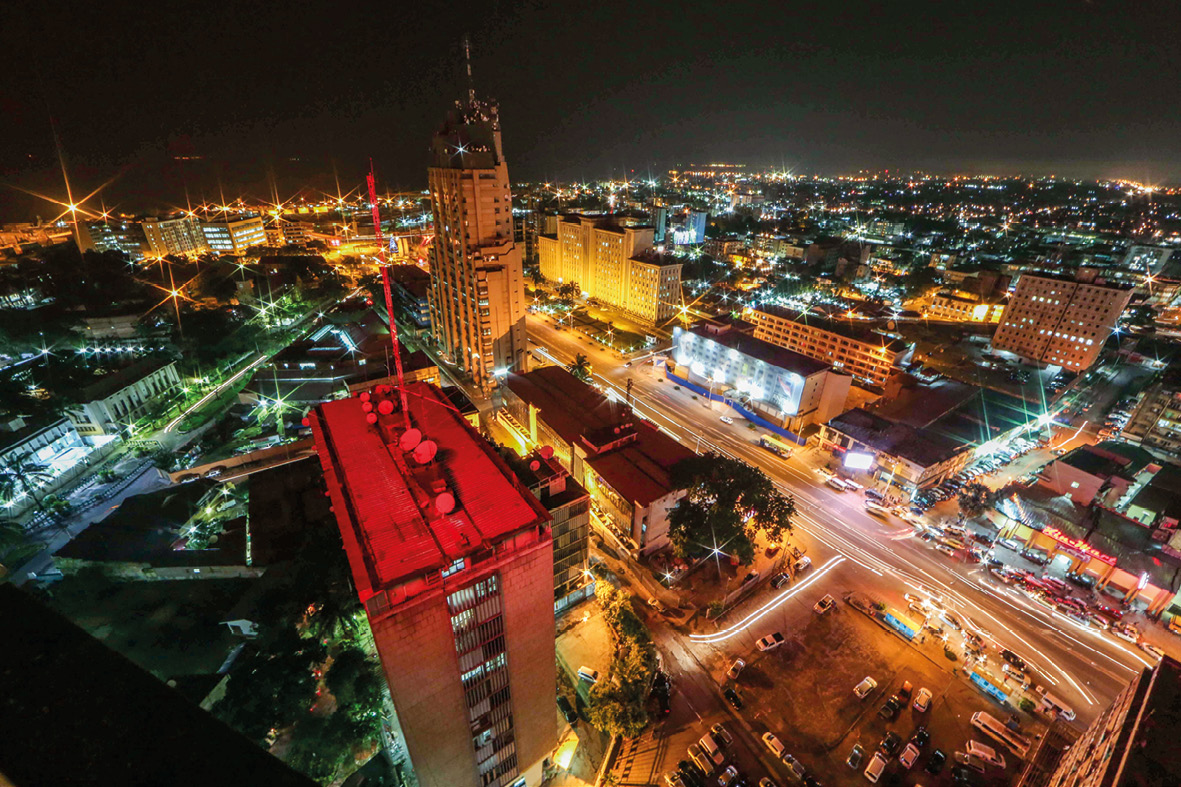
Figure 5.8 Night lights of Kinshasa, capital of the DRC and Africa’s second largest city. To have more people leads to more competition for space, leaving less space to maintain biodiversity and ecosystem services. It also means more natural resources extracted, more pollution, and more greenhouse gas emissions. Photograph by MONUSCO/Abel Kavanagh, https://www.flickr.com/photos/monusco/23769991270, CC BY-SA 2.0.
In recent years, there has been an increasing tendency of economists, scientists, and politicians to shift the focus from population growth to consumption as the more important underlying driver of biodiversity loss. For many, the emphasis on consumption avoids politically charged topics, such as population control, which most people oppose on ethical or moral grounds, and because it is associated with divisive topics such as xenophobia, racism, and eugenics (Kolbert and Roberts, 2017). Others highlight that it is not the number of people per se, but how natural resources are consumed that is the main cause of environmental decline. Indeed, affluent people and affluent countries have a disproportionate impact on the natural environment because they consume a disproportionately large share of the world’s natural resources. To use one example, the USA accommodates only 5% of the world’s human population but uses 25% of the world’s harvested natural resources each year (WRI, 2019). In fact, decorative Christmas lights in the USA alone use more energy than the annual energy usage of the entirety of Ethiopia or Tanzania (Moss and Agyapong, 2015). And yet, the average USA citizen uses less than half of the energy (measured as carbon emissions) that an average citizen of Qatar uses (World Bank, 2019; see also Figure 5.9), Qatar being a small but wealthy Middle Eastern country.
The major threats to biodiversity are all rooted in expanding human populations and unsustainable consumption patterns.
The global demand for natural resources such as coffee, cacao, palm oil, and timber is helping fuel habitat loss in Africa.
Another important aspect to consider in the consumption argument is that, through increased globalisation, the impacts of consumption in industrialised countries are felt over much greater distances than before (Moran and Kanemoto, 2017). For instance, chocolate consumed in Europe was most likely made with cacao produced in West Africa (Gockowski and Sonwa, 2011); other crops, such as coffee and tea, produced in Africa are similarly enjoyed all over the world. In the best-case scenario, African farmers are satisfying a demand in a global market; at worst, foreign companies are establishing croplands with little benefit trickling down to local people. Supporters of the consumption argument rightfully point out that it would be very unjust to blame the local farmers for cleared forests when they simply produce commodity crops that the international market demands.
As with many other complex challenges, both sides of the population-consumption debate are correct. One method to link the impact (I) of a human population on the environment is through the formula I = P × A × T (IPAT in short), where P is population size, A is Affluence (e.g. per capita GPD), and T is technology (e.g. per capita energy use) (Ehrlich and Goulder, 2007). The IPAT equation is similar in concept to the ecological footprint (Figure 5.9): both illustrate that human populations and consumption patterns interact to exacerbate human impacts on the environment. In other words, many poor Africans can have the same impact on the environment as just a few wealthy Americans, and vice versa.
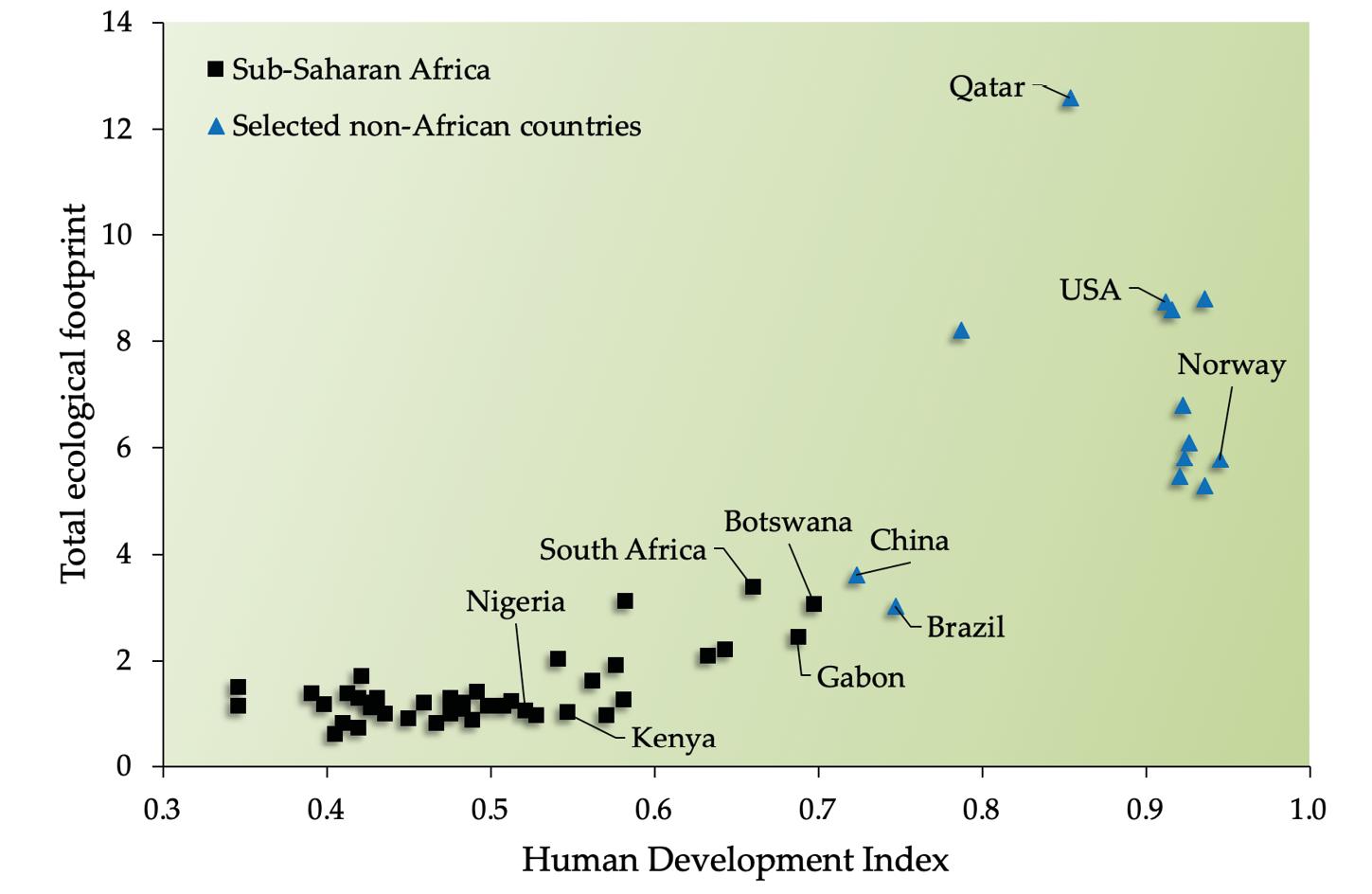
Figure 5.9 A nation’s ecological footprint is calculated by estimating the amount of land needed to support the average resident of that nation. Although there is some disagreement as to the exact methods for these calculations, the overall message is clear: people in more developed nations use a disproportionately large amount of natural resources. However, the overall impacts of countries with huge populations, such as China, are also huge because of the cumulative impact from so many people. Source: GFN, 2017, CC BY 4.0.
Both the IPAT equation and ecological footprint concept are insightful as to the challenges facing Africa’s ecosystems and people. Today, Africans are increasingly aspiring to attain the same levels of high consumption as industrial countries. These patterns generally lead to an inefficient, wasteful, and unsustainable use of natural resources (i.e. overconsumption). Population growth rates in many industrial countries are currently slowing; some countries are even experiencing long-term population declines, which allow conservation-minded individuals in those countries to focus their efforts on addressing consumption patterns. The situation is quite different in Africa, where we are faced with increasing per capita consumption and the fastest growing human population on Earth. In the face of the resulting increased competition for space, African conservation biologists must adopt a holistic approach to ensure that welfare standards are upheld or improved while our natural heritage is protected. One of the most important strategies involves championing sustainable economic development over unsustainable economic growth (Section 15.1). While conservation biologists differ in terms of how strongly they argue for addressing the population size issue, most also agree that conservation goals benefit from education, the empowerment of women, and wider access to family planning and reproductive health services.
5.5 Concluding Remarks
There is no doubt that agriculture, forestry, and infrastructure developments—the main drivers of habitat loss and fragmentation—play an important role in socio-economic development across Africa. Nevertheless, many (perhaps most) of these developments are set up to benefit a select few individuals and corporations primarily interested in short-term gains rather than a wide range of stakeholders over the long-term. To maintain biodiversity and improve our quality of life, governments across the region must ensure that the benefits of development are shared fairly across society and that industries are accountable for their fair share of the natural resources they use (Section 4.5.3). Also, the region’s growing number of wealthy people who benefit most from development must re-evaluate their lifestyles (whether willingly or through government interventions, such as taxation) to avoid excessive consumption patterns. Some of the first steps may be relatively easy. For example, the water used to produce Sub-Saharan Africa’s wasted food—a full third of all produced food (FAO, 2013)—equals the annual discharge of the mighty Zambezi River where it enters the Indian Ocean in Mozambique (Beilfuss and dos Santos, 2001). At the same time, we must all play our part in achieving sustainable development, by encouraging family planning activities and assisting industries to grow in a responsible way (Section 15.1). Neglecting that, we compromise our own futures, and that of our children.
5.6 Summary
- One of the primary threats to biodiversity today are habitat loss and habitat fragmentation. Many species living in tropical forests, freshwater ecosystems, the marine environment, and seasonal drylands are at risk of extinction due to habitat loss.
- The theory of island biogeography and the species-area relationship can be used to predict the numbers of species that will go extinct due to habitat loss. Both theories predict that large habitat patches are better able to maintain wildlife populations because they accommodate populations better buffered against extinction.
- Habitat fragmentation describes the process when once large and widespread habitats (and hence wildlife populations) are divided into several increasingly smaller and isolated units. This process leads to extinctions because it impedes dispersal, colonisation, foraging, and reproduction.
- Edge effects reduce the functional size of habitats because they alter microclimates and expose habitat specialists to displacement by invasive species, predators, and other disturbances.
- Habitat loss and fragmentation are rooted in expanding human populations and excessive consumption of natural resources. The IPAT equation illustrates how population size, wealth, and technology together determine our impact on the environment.
5.7 Topics for Discussion
- Why does oil palm (Elaeis guineensis, LC) cultivation represent a significant threat to biodiversity in Africa? (In addition to your own research, it might also be useful to read Box 6.1.)
- Read Harris et al. (2009) on the decline of the world’s mass wildlife migrations. Which lost African migration appeals to you most and why? What species were involved? What numbers of animals were involved? How do you think this migration can be revived?
- Which ecosystem in your region would you consider the most damaged, and which would you consider the most pristine? Can you explain why these two ecosystems have such different fates?
- Do you agree with the idea that human population growth is the primary driver of extinctions today? Why? How do we balance protecting biodiversity with providing for a growing human population, and the right of people to have children?
5.8 Suggested Readings
Arcilla, N., L.H. Holbech, and S. O’Donnell. 2015. Severe declines of understory birds follow illegal logging in Upper Guinea forests of Ghana, West Africa. Biological Conservation 188: 41–49. https://doi.org/10.1016/j.biocon.2015.02.010 Illegal logging is on the increase, and wildlife communities struggle to recover.
Caro, T., J. Darwin, T. Forrester, et al. 2012. Conservation in the Anthropocene. Conservation Biology 26: 185–88. https://doi.org/10.1111/j.1523-1739.2011.01752.x Even though human activities dominate large areas of the earth, it is important to remember and plan for the many places and ecosystems where human influence is still minimal.
Haddad, N.M., L.A. Brudvig, J. Clobert, et al. 2015. Habitat fragmentation and its lasting impact on Earth’s ecosystems. Science Advances 1: e1500052. https://doi.org/10.1126/sciadv.1500052 There are many ways that fragmentation hurts biodiversity.
Harris, G., S. Thirgood, J.G.C. Hopcraft, et al. 2009. Global decline in aggregated migrations of large terrestrial mammals. Endangered Species Research 7: 55–76. https://doi.org/10.3354/esr00173 Habitat loss continues to threaten the world’s remaining mass migrations.
Ibisch, P.L., M.T. Hoffmann, S. Kreft, et al. 2016. A global map of roadless areas and their conservation status. Science 354: 1423–27. https://doi.org/10.1126/science.aaf7166 Africa still holds large roadless areas; we need to keep it that way.
Laurance, W.F., J. Sayer, and K.G. Cassman. 2014. Agricultural expansion and its impacts on tropical nature. Trends in Ecology and Evolution 29: 107–16. https://doi.org/10.1016/j.tree.2013.12.001 Agriculture and roads will have severe impacts on Africa’s ecosystems in the coming century.
Rudel, T.K. 2013. The national determinants of deforestation in sub-Saharan Africa. Philosophical Transactions of the Royal Society B 368: 20120405. https://doi.org/10.1098/rstb.2012.0405 One manuscript in a special issue on deforestation in Africa; other manuscripts in this issue are also worth scanning.
van der Hoeven, C.A., W.F. de Boer, and H.H. Prins. 2010. Roadside conditions as predictor for wildlife crossing probability in a Central African rainforest. African Journal of Ecology 48: 368–77. https://doi.org/10.1111/j.1365-2028.2009.01122.x Some species are highly reluctant to cross roads, even inside protected areas.
Woodborne, S., K.D.A. Huchzermeyer, D. Govender, et al. 2012. Ecosystem change and the Olifants River crocodile mass mortality events. Ecosphere 3: 1–17. https://doi.org/10.1890/ES12-00170.1 Damming rivers can lead to ecological disasters
Ykhanbai, H., R. Garg, A. Singh, et al. 2014. Conservation and “Land Grabbing” in Rangelands: Part of the Problem or Part of the Solution? (Rome: International Land Coalition). http://pubs.iied.org/pdfs/G03853.pdf Conservation biologists should work with local communities to prevent land grabs.
Bibliography
Abbink, J. 2011. ‘Land to the foreigners’: Economic, legal, and socio-cultural aspects of new land acquisition schemes in Ethiopia. Journal of Contemporary African Studies 29: 513–35. https://doi.org/10.1080/02589001.2011.603213
Achard, F., R. Beuchle, P. Mayaux, Het al. 2014. Determination of tropical deforestation rates and related carbon losses from 1990 to 2010. Global Change Biology 20: 2540–54. https://doi.org/10.1111/gcb.12605
Acreman, M.C. 1996. Environmental effects of hydro‐electric power generation in Africa and the potential for artificial floods. Water and Environment Journal 10: 429–35. https://doi.org/10.1111/j.1747-6593.1996.tb00076.x
Arcilla, N., L.H. Holbech, and S. O’Donnell. 2015. Severe declines of understory birds follow illegal logging in Upper Guinea forests of Ghana, West Africa. Biological Conservation 188: 41–49. https://doi.org/10.1016/j.biocon.2015.02.010
Austin, K.G., M. González-Roglich, D. Schaffer-Smith, et al. 2017. Trends in size of tropical deforestation events signal increasing dominance of industrial-scale drivers. Environmental Research Letters 12: 054009. https://doi.org/10.1088/1748-9326/aa6a88
Beier, P., M. van Drielen, and B.O. Kankam. 2002. Avifaunal collapse in West African forest fragments. Conservation Biology 16: 1097–111. https://doi.org/10.1046/j.1523-1739.2002.01003.x
Beilfuss, R., and D. dos Santos. 2001. Patterns of hydrological change in the Zambezi Delta, Mozambique. Working Paper 2 (Baraboo: International Crane Foundation). https://doi.org/10.13140/RG.2.2.14255.12961
Benítez-López, A., R. Alkemade, A.M. Schipper, et al. 2017. The impact of hunting on tropical mammal and bird populations. Science 356: 180–83. https://doi.org/10.1126/science.aaj1891
BirdLife International. 2019. Migratory birds and flyways. https://www.birdlife.org/worldwide/programmes/migratory-birds
Blake, S., S.L. Deem, S. Strindberg, et al. 2008 Roadless wilderness area determines forest elephant movements in the Congo Basin. PloS ONE 3: e3546. https://doi.org/10.1371/journal.pone.0003546
Both, C., S. Bouwhuis, C.M. Lessells, et al. 2006. Climate change and population declines in a long-distance migratory bird. Nature 441: 81–83. https://doi.org/10.1038/nature04539
Bredenhand, E., and M.J. Samways. 2009. Impact of a dam on benthic macroinvertebrates in a small river in a biodiversity hotspot: Cape Floristic Region, South Africa. Journal of Insect Conservation 13: 297–307. http://doi.org/10.1007/s10841-008-9173-2
Cordeiro, N.J., H.J. Ndangalasi, J.P. McEntee, et al. 2009. Disperser limitation and recruitment of an endemic African tree in a fragmented landscape. Ecology 90: 1030–41. http://doi.org/10.1890/07-1208.1
Corlett, R., and R.B. Primack. 2010. Tropical Rainforests: An Ecological and Biogeographical Comparison (Malden: Wiley-Blackwell). https://doi.org/10.1002/9781444392296
Dale, S., K. Mork, R. Solvang, et al. 2000. Edge effects on the understorey bird community in a logged forest in Uganda. Conservation Biology 14: 265–76. https://doi.org/10.1046/j.1523-1739.2000.98340.x
Davidson, N.C. 2014. How much wetland has the world lost? Long-term and recent trends in global wetland area. Marine and Freshwater Research 65: 934–41. https://doi.org/10.1071/MF14173
deGeorges, A., and B.K. Reilly. 2006. Dams and large-scale irrigation on the Senegal River: impacts on man and the environment. International Journal of Environmental Studies 63: 633–44. https://doi.org/10.1080/00207230600963296
Drechsel, P., L. Gyiele, D. Kunze, et al. 2001. Population density, soil nutrient depletion, and economic growth in sub-Saharan Africa. Ecological Economics 38: 251–58. https://doi.org/10.1016/S0921-8009(01)00167-7
Drijver, C.A., and M. Marchand, 1985. Taming the floods. Environmental aspects of the floodplain developments of Africa (Leiden: Centre of Environmental Studies, University of Leiden).
Durant, S.M., M.S. Becker, S. Creel, et al. 2015. Developing fencing policies for dryland ecosystems. Journal of Applied Ecology 52: 544–51. https://doi.org/10.1111/1365-2664.12415
Dybas, C.L. 2015. Forests between the tides: Conserving Earth’s vanishing mangrove ecosystems. BioScience 65: 1039–45. https://doi.org/10.1093/biosci/biv132
Ehrlich, P.R., and L.H. Goulder. 2007. Is current consumption excessive? A general framework and some indications for the United States. Conservation Biology 21: 1145–54. https://doi.org/10.1111/j.1523-1739.2007.00779.x
Estrada, A., P.A. Garber, A.B. Rylands, et al. 2017. Impending extinction crisis of the world’s primates: Why primates matter. Science Advances 3: e1600946. https://doi.org/10.1126/sciadv.1600946
FAO (Food and Agriculture Organisation). 2013. Food wastage footprint: Impacts on natural resources. Summary Report (Rome: FAO). http://www.fao.org/docrep/018/i3347e/i3347e.pdf
Feka, N.Z., G.B. Chuyong, and G.N. Ajonina. 2009. Sustainable utilization of mangroves using improved fish-smoking systems: A management perspective from the Douala-Edea wildlife reserve, Cameroon. Tropical Conservation Science 2: 450–68. https://doi.org/10.1177/194008290900200406
FFI and FDA (Forestry Development Authority). 2013. National action plan for the conservation of the pygmy hippopotamus in Liberia (Cambridge: FFI; Monrovia: FDA).
Freedman, A.H., W. Buermann, M. Lebreton, et al. 2009. Modeling the effects of anthropogenic habitat change on savanna snake invasions into African rainforest. Conservation Biology 23: 81–92. https://doi.org/10.1111/j.1523-1739.2008.01039.x
GFN (Global Footprint Network). 2017. National Footprint Accounts. http://www.footprintnetwork.org
Giri, C., E. Ochieng, L.L. Tieszen, et al. 2011. Status and distribution of mangrove forests of the world using Earth observation satellite data. Global Ecology and Biogeography 20: 154–59. https://doi.org/10.1111/j.1466-8238.2010.00584.x
Gockowski, J., and D. Sonwa. 2011. Cocoa intensification scenarios and their predicted impact on CO2 emissions, biodiversity conservation, and rural livelihoods in the Guinea Rain Forest of West Africa. Environmental Management 48: 307–21. https://doi.org/10.1007/s00267-010-9602-3
Gómez, C., N.J. Bayly, D.R. Norris, et al. 2017. Fuel loads acquired at a stopover site influence the pace of intercontinental migration in a boreal songbird. Scientific Reports 7: 3405. https://doi.org/10.1038/s41598-017-03503-4
Gopal, B. 2013. Mangroves are wetlands, not forests: Some implications for their management. In: Mangrove Ecosystems of Asia, ed. by I. Faridah-Hanum et al. (New York: Springer). https://doi.org/10.1007/978-1-4614-8582-7
Greger, M.M. 2012. Traditional Healers as a Foundation Pillar of Medicinal Plant Conservation in Uganda. M.Sc. Thesis (Ås: Norwegian University of Life Sciences).
Haddad, N.M., L.A. Brudvig, J. Clobert, et al. 2015. Habitat fragmentation and its lasting impact on Earth’s ecosystems. Science Advances 1: e1500052. https://doi.org/10.1126/sciadv.1500052
Hansen, M.C., P.V. Potapov, R. Moore, et al. 2013. High-resolution global maps of 21st-century forest cover change. Science 342: 850–53. https://doi.org/10.1126/science.1244693 Data available on-line from: http://earthenginepartners.appspot.com/science-2013-global-forest
Harris, G., S. Thirgood, J.G.C. Hopcraft, et al. 2009. Global decline in aggregated migrations of large terrestrial mammals. Endangered Species Research 7: 55–76. https://doi.org/10.3354/esr00173
Hillers A., G.M. Buchanan, J.C. Garteh, et al. 2017. A mix of community-based conservation and protected forests is needed for the survival of the Endangered pygmy hippopotamus Choeropsis liberiensis. Oryx 51: 230–39. https://doi.org/10.1017/S003060531600020X
Hoffmann, M., J.W. Duckworth, K. Holmes, et al. 2015. The difference conservation makes to extinction risk of the world’s ungulates. Conservation Biology 29: 1303–13. https://doi.org/10.1111/cobi.12519
Hopcraft, J.G.C., S.A.R. Mduma, M. Borner, et al. 2015. Conservation and economic benefits of a road around the Serengeti. Conservation Biology 29: 932–36. https://doi.org/10.1111/cobi.12470
Ibisch, P.L., M.T. Hoffmann, S. Kreft, et al. 2016. A global map of roadless areas and their conservation status. Science 354: 1423–27. https://doi.org/10.1126/science.aaf7166
IBPES. 2019. Nature’s dangerous decline ‘unprecedented’: Species extinction rates accelerating. IBPES media release. https://www.ipbes.net/news/Media-Release-Global-Assessment
IUCN. 2019. The IUCN Red List of Threatened Species. http://www.iucnredlist.org
Kalwij, J.M., M.P. Robertson, and B.J. Rensburg. 2008. Human activity facilitates altitudinal expansion of exotic plants along a road in montane grassland, South Africa. Applied Vegetation Science 11: 491–98. https://doi.org/10.3170/2008-7-18555
Kariuki, M. and K. Ndang’ang’a, 2018. Can the Critically Endangered Liben lark be saved? Our latest update. BirdLife News. https://www.birdlife.org/worldwide/news/can-critically-endangered-liben-lark-be-saved-our-latest-update
Kirby, J.S., A.J. Stattersfield, S.H.M. Butchart, et al. 2008. Key conservation issues for migratory land-and waterbird species on the world’s major flyways. Bird Conservation International 18: S49–S73. https://doi.org/10.1017/S0959270908000439
Kolbert, E., and D. Roberts. 2017. I’m an environmental journalist, but I never write about overpopulation. Here’s why. Vox. https://www.vox.com/energy-and-environment/2017/9/26/16356524/the-population-question
Koohafkan, P., M. Salman, and C. Casarotto. 2011. Investments in land and water. In: The State of the World’s Land and Water Resources for Food and Agriculture (SOLAW)—Managing Systems at Risk (Rome: FAO; London: Earthscan). http://www.fao.org/docrep/017/i1688e/i1688e.pdf
Laurance, W.F., J. Sayer, and K.G. Cassman. 2014. Agricultural expansion and its impacts on tropical nature. Trends in Ecology and Evolution 29: 107–16. https://doi.org/10.1016/j.tree.2013.12.001
Lehouck, V., T. Spanhove, L. Colson, et al. 2009. Habitat disturbance reduces seed dispersal of a forest interior tree in a fragmented African cloud forest. Oikos 118: 1023–34. https://doi.org/10.1111/j.1600-0706.2009.17300.x
Lewis, R.R. 2005. Ecological engineering for successful management and restoration of mangrove forests. Ecological Engineering 24: 403–18. https://doi.org/10.1016/j.ecoleng.2004.10.003
Luther, D.A., and R. Greenberg. 2009. Mangroves: A global perspective on the evolution and conservation of their terrestrial vertebrates. BioScience 59: 602–12. https://doi.org/10.1525/bio.2009.59.7.11
MacArthur, R.H., and E.O. Wilson. 2015. Theory of Island Biogeography (Princeton: Princeton University Press).
Mahamued, B.A. 2016. Designing a Rangeland to Preserve Africa’s Most Endangered Mainland Bird and a People’s Way of Life. Doctoral dissertation (Manchester: Manchester Metropolitan University).
Mertens, B., and E.F. Lambin. 1997. Spatial modelling of deforestation in southern Cameroon: Spatial disaggregation of diverse deforestation processes. Applied Geography 17: 143–62. https://doi.org/10.1016/S0143-6228(97)00032-5
Moran, D., and K Kanemoto. 2017. Identifying species threat hotspots from global supply chains. Nature Ecology and Evolution 1: 0023. https://doi.org/10.1038/s41559-016-0023
Morgan, B.J., E.E. Abwe, A.F. Dixson, et al. 2013. The distribution, status, and conservation outlook of the drill (Mandrillus leucophaeus) in Cameroon. International Journal of Primatology 34: 281–302. http://doi.org/10.1007/s10764-013-9661-4
Moss, T., and P. Agyapong. 2015. US holiday lights use more electricity than El Salvador does in a year (London: Center for Global Development). http://www.cgdev.org/blog/us-holiday-lights-use-more-electricity-el-salvador-does-year
Neville, K. 2015. The contentious political economy of biofuels. Global Environmental Politics 15: 21–40. https://doi.org/10.1162/GLEP_a_00270
Olson, D.M., and E. Dinerstein. 2002. The Global 200: Priority ecoregions for global conservation. Annals of the Missouri Botanical Garden 89: 199–224. https://doi.org/10.2307/3298564
Potapov, P., M.C. Hansen, L. Laestadius, et al. 2017. The last frontiers of wilderness: Tracking loss of intact forest landscapes from 2000 to 2013. Science Advances 3: e1600821. https://doi.org/10.1126/sciadv.1600821
Primack, R.B. 2012. A Primer for Conservation Biology (Sunderland: Sinauer).
Ransom, C, P.T. Robinson, and B. Collen. 2015. Choeropsis liberiensis. The IUCN Red List of Threatened Species 2015: e.T10032A18567171. http://doi.org/10.2305/IUCN.UK.2015-2.RLTS.T10032A18567171.en
Rudel, T.K. 2013. The national determinants of deforestation in sub-Saharan Africa. Philosophical Transactions of the Royal Society B 368: 20120405. https://doi.org/10.1098/rstb.2012.0405
Runge, C.A., J.E.M. Watson, S.H.M. Butchart, et al. 2015. Protected areas and global conservation of migratory birds. Science 350: 1255–58. https://doi.org/10.1126/science.aac9180
Rushworth, I., and S. Krüger. 2014. Wind farms threaten southern Africa’s cliff-nesting vultures. Ostrich 8: 13–23. http://doi.org/10.2989/00306525.2014.913211
Sande, E., P.K. Ndang’ang’a, J. Wakelin, J., et al. 2008. International single species action plan for the conservation of the White-winged Flufftail Sarothrura ayresi. CMS Technical Series 19 (Bonn: CMS and AWEA). http://www.cms.int/sites/default/files/publication/Whitewinged_flufftail_3_0_0.pdf
Sayer, J. 1992. A future for Africa’s tropical forests. In: The Conservation Atlas of Tropical Forests: Africa, ed. by J.A. Sayer et al. (London: Palgrave Macmillan). https://portals.iucn.org/library/sites/library/files/documents/1992-063.pdf
Sedláček, O., M. Mikeš, T. Albrecht, et al. 2014. Evidence for an edge effect on avian nest predation in fragmented afromontane forests in the Bamenda-Banso Highlands, NW Cameroon. Tropical Conservation Science 7: 720–32. https://doi.org/10.1177/194008291400700410
Smaller, C., Q. Wei, and L. Yalan. 2012. Farmland and water: China invests abroad (Winnipeg: IISD). https://www.iisd.org/pdf/2012/farmland_water_china_invests.pdf
Sokolow, S.H., E. Huttinger, N. Jouanard, et al. 2015. Reduced transmission of human schistosomiasis after restoration of a native river prawn that preys on the snail intermediate host. Proceedings of the National Academy of Sciences 112: 9650–55. https://doi.org/10.1073/pnas.1502651112
Spottiswoode, C.N., M. Wondafrash, M. Gabremicheal, et al. 2009. Rangeland degradation is poised to cause Africa’s first recorded avian extinction. Animal Conservation 12: 249–57. https://doi.org/10.1111/j.1469-1795.2009.00246.x
Spottiswoode, C.N., U. Olsson, M.S. Mills, et al. 2013. Rediscovery of a long-lost lark reveals the conspecificity of endangered Heteromirafra populations in the Horn of Africa. Journal of Ornithology 154: 813–25. https://doi.org/10.1007/s10336-013-0948-1
Stabach, J.A., G. Wittemyer, R.B. Boone, et al. 2016. Variation in habitat selection by white‐bearded wildebeest across different degrees of human disturbance. Ecosphere 7: e01428. https://doi.org/10.1002/ecs2.1428
Tabuti, J.R.S. 2006. Traditional knowledge in Bulamogi County - Uganda: Importance to sustainable livelihoods. In: African Knowledge and Sciences: Understanding and Supporting the Ways of Knowing in Sub-Saharan Africa, ed. by D. Millar et al. (Leusden: Compas).
Tucker, M.A., K. Böhning-Gaese, W.F. Fagan, et al. 2018. Moving in the Anthropocene: Global reductions in terrestrial mammalian movements. Science 359: 466–69. https://doi.org/10.1126/science.aam9712
Tyukavina, A., M.C. Hansen, P. Potapov, et al. 2018. Congo Basin forest loss dominated by increasing smallholder clearing. Science Advances 4: eaat2993. https://doi.org/10.1126/sciadv.aat2993
van Bochove, J., E. Sullivan, and T. Nakamura. 2014. The Importance of Mangroves to People: A Call to Action (Cambridge: UNEP). http://wedocs.unep.org/handle/20.500.11822/9300
van der Hoeven, C.A., W.F. de Boer, and H.H. Prins. 2010. Roadside conditions as predictor for wildlife crossing probability in a Central African rainforest. African Journal of Ecology 48: 368–77. https://doi.org/10.1111/j.1365-2028.2009.01122.
van Wilgen, B.W., J.L. Nel, and M. Rouget. 2007. Invasive alien plants and South African rivers: A proposed approach to the prioritization of control operations. Freshwater Biology 52: 711–23. https://doi.org/10.1111/j.1365-2427.2006.01711.x
Vickery, J.A., S.R. Ewing, K.W. Smith, et al. 2014. The decline of Afro‐Palaearctic migrants and an assessment of potential causes. Ibis 156: 1–22. https://doi.org/10.1111/ibi.12118
Vogt, T. 2011. Results of Sapo National Park bio-monitoring programme 2007–2009. FFI-FDA Biomonitoring and Research Report (Cambridge: FFI; Monrovia: FDA).
von Braun, J., and R.S. Meinzen-Dick. 2009. “Land grabbing” by foreign investors in developing countries: Risks and opportunities (Washington: IFPRI). http://ebrary.ifpri.org/cdm/ref/collection/p15738coll2/id/14853
Wallenfang, J., M. Finckh, J. Oldeland, et al, 2015. Impact of shifting cultivation on dense tropical woodlands in southeast Angola. Tropical Conservation Science 8: 863–92. https://doi.org/10.1177/194008291500800402
Weisse, M., and E.D. Goldman. 2019. The world lost a Belgium-sized area of primary rainforest last year. World Resources Institute Blog. https://www.wri.org/blog/2019/04/world-lost-belgium-sized-area-primary-rainforests-last-year
Woodborne, S., K.D.A. Huchzermeyer, D. Govender, et al. 2012. Ecosystem change and the Olifants River crocodile mass mortality events. Ecosphere 3: 1–17. https://doi.org/10.1890/ES12-00170.1
World Bank. 2019. World Bank Open Data. http://data.worldbank.org/region/sub-saharan-africa
WRI (World Resources Institute). 2019. Climate Analysis Indicators Tool: WRI’s Climate Data Explorer. http://cait2.wri.org
Ykhanbai, H., R. Garg, A. Singh, et al. 2014. Conservation and “land grabbing” in rangelands: Part of the problem or part of the solution? (Rome: International Land Coalition). http://www.landcoalition.org/sites/default/files/documents/resources/conservation%20and%20land%20grabbing%20in%20rangelands_web_en_0.pdf