7. Extreme Weather Events and Local Impacts of Climate Change: The Scientific Perspective
© Friederike E. L. Otto, CC BY 4.0 https://doi.org/10.11647/OBP.0212.07
While global and regional temperature increases are the most certain indicators of anthropogenic climate change, due to the emissions from burning fossil fuels, the damage caused by climate change is most clearly manifest in changes in seasons and extreme weather events. Recent advances in the attribution of extreme weather events, combined with newly available observations of past weather and climate, have made it possible to causally link high-impact extreme events to human-induced climate change. The level of confidence in these findings, however, varies according to the type of event and region of the world. While the increase in heatwaves can be quantified with confidence in most parts of the world, attribution assessments for droughts and hurricanes are much more uncertain.
From Global Climate Change to Local Impacts
Scientific reports, political debates, and to a large extent the media, use global mean temperature rise as the metric to determine how humans are changing the climate by burning fossil fuels. Most prominently, the United Nations Framework Convention on Climate Change negotiations used this metric to measure and discuss the changing climate. More recently, the December 2015 Paris Agreement defines the central goal of international climate policy and politics as limiting global mean temperature increases to well below 2°C.
It is, however, not the abstract measure of global mean temperature that will cause loss and damage from climate change. Instead, the impacts of climate change primarily manifest themselves through rising sea levels and the changing risks of extreme weather events. This chapter provides a scientific account of the kinds of changes that are already being experienced locally, and the changes that we might see in the future. It then assesses what climate scientists know about the attribution of weather events (such as those discussed elsewhere in this volume) to global warming.
From Greenhouse Gas Emissions to Weather
The levels of scientific evidence and our ability to quantify each link in the chain of causality from greenhouse gas emissions via global mean temperature increases to local effects on weather and hydrology, to impacts affecting society, and loss and damage vary considerably (see Fig. 7.1). The evidence of the first element, from individual countries’ (Skeie et al. 2017) and companies’ (Heede 2014) greenhouse gas emissions to concentrations in the atmosphere, is unambiguous. The next link in the chain—from atmospheric CO2 concentrations to global mean temperature—is also certain and has been known for a long time (IPCC 2014). Recent quantitative assessments estimate the global mean temperature increase resulting from anthropogenic greenhouse gas emissions from the beginning of the industrial revolution to the present at about 1°C (Haustein et al. 2017). The evidence is also strong for the increase in large-scale average temperatures; the absolute changes are very different, but temperatures are increasing in all inhabited regions of the world. For instance in Europe, annual mean temperatures have changed twice as fast as the global average (Hegerl et al. 2018; IPCC 2014). In recent years an increase in global precipitation that is expected in a warming climate has also been observed and attributed to human-induced greenhouse gas emissions (Marvel et al. 2017; IPCC 2014; Allen and Ingram 2002).
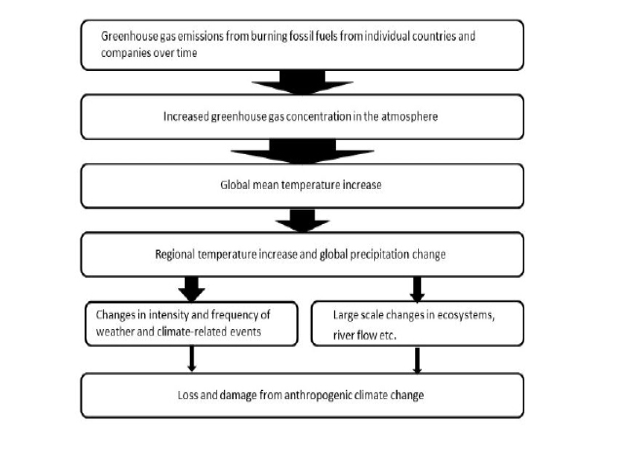
Fig. 7.1 Chain of causality from greenhouse gas emissions to loss and damage. The width of the arrow signifies the relative level of confidence in the quantitative assessments for different parts of the chain. For the last four boxes, confidence levels are very different depending on the region and type of event (e.g., heatwaves have high confidence, windstorms have low confidence) or impact (source: author, 2020).
The next—and arguably most crucial for the day-to-day lives of the majority of the world’s population—link from anthropogenic climate change and global warming to local or regional individual weather and climate-related events has long been impossible to make with confidence. Yet, this has changed in recent years. One of the most noticeable local effects is the change in seasons: the mid-latitudes are experiencing an earlier spring and shorter winters, which is clearly caused by anthropogenic climate change (Santer et al. 2018; Christidis, Jones, and Stott 2014). Quantifying and establishing the link between individual weather events, which often cause significant damage, has been the focus of the emerging science of extreme event attribution (Otto 2017; Stott et al. 2016). This strand of research is ambitious because whether and to what extent an extreme weather event leads to impacts on natural and human systems depends not only on the meteorological hazard (i.e., the weather event) but also on the vulnerability and exposure to that hazard (i.e., who or what is in harm’s way). Attributing damage and losses from extreme weather to climate change is thus possible with quantitative information on vulnerability and exposure and an understanding of local decision-making and governance structures, but very few studies have assessed this final link in the chain of causality (Mitchell et al. 2016; Schaller et al. 2016). However, enormous progress has been made in being able to robustly and routinely determine whether and to what extent anthropogenic climate change has altered the likelihood of extreme weather events to occur. Compared to only five years ago, we are now able to much more fully understand what anthropogenic global greenhouse gas emissions translate to for a particular location, because we can quantify the link between global warming and extreme weather. This is a crucial step that facilitates a true assessment of the changing risks on the level at which both individual and political decisions are made.
In 2003, a landmark publication (Allen 2003) suggested approaching the attribution question regarding the role of anthropogenic climate change in extreme weather events (Otto et al. 2016) in a probabilistic way. This approach entails assessing the likelihood of the extreme event in question to occur in the current climate, all man-made drivers included, and comparing it with the probability of its occurrence in a world without human-induced climate change in order to isolate and quantify the effect of climate change. The first paper to apply this approach was published in 2004; it found an increase of at least 100% in the likelihood that the 2003 European heatwave was attributable to human-induced climate change (Stott, Stone, and Allen 2004).
For today’s climate, it is possible to use observations of weather and climate to estimate the likelihood that an event will occur. Yet we lack observations of a hypothetical, counterfactual world without anthropogenic climate change. Furthermore, we can only observe weather that has occurred; it is not possible to observe all weather events that are possible in a given climate. The method of event attribution thus relies on climate models to simulate possible weather, including the extreme event in question, in a given region and season accurately enough to draw robust conclusions on the role of climate change. Early studies applying the probabilistic event attribution approach employed a single climate model; thus, the results are heavily dependent on that model’s reliability. A methodology has recently been developed that includes multiple models and several lines of evidence; a whole new field of climate science has emerged, and the methods are constantly improving. Two aspects of the methodology are important to stress here. First, the definition of an extreme event is a crucial part of the analysis and determines the outcome. In the most widely applied approach discussed here, the event is always defined as a type of weather that leads to an impact (e.g., extreme rainfall above a certain threshold in a particular area or season that causes flooding). Other approaches favor much more narrow definitions of an event by, e.g., conditioning on the exact atmospheric circulation state without assessing the likelihood of such a situation (Otto et al. 2016). The latter is useful for understanding the impact of climate change on weather but less useful for local decision-making. Second, attribution of extreme events is only reliable for the types of events for which climate models are available that realistically simulate the type of event; thus, the evidence of a causal link between anthropogenic climate change and localized weather events is not comprehensive.
Because the role of climate change is always mentioned by the media, by members of the public, and by people affected by the impacts of an extreme event, a significant amount of public attention has been focused on the development of the science. This exposure has also generated close scrutiny of scientific studies by peers; critical publications from both sides claim that scientists are too confident in their attribution statements (Bellprat and Doblas-Reyes 2016) or that they are too cautious (Lloyd and Oreskes 2018). While this might have produced temporary confusion in public understanding, overall, the public scientific discussions led to the comparably fast development of better and more robust methods of estimating changing hazards and reducing the uncertainty in the results.
In 2016, the US National Academy of Sciences asked climate scientists who were not involved in the science itself to write a report on the state of event attribution science and the robustness of the results of such studies. The resulting report (National Academies of Sciences, Engineering, and Medicine 2016) concluded that the results were robust overall, but not for all types of extreme events. For example, current climate models cannot reliably simulate very small-scale events, like flash floods, hail, or tornados, in order to assess the role of climate change in their occurrence. Nor do we have comprehensive observations on hailstorms with which to evaluate climate models. By contrast, since heatwaves and large-scale rainfall events are well observed and simulated, confidence in the attribution results is high. For droughts and other more complex events resulting from a combination of meteorological drivers (such as a lack of rainfall and high evaporation), confidence very much depends on which aspects of the droughts are studied. Despite these limitations, this independent report written by first-class climate scientists noted the thoroughness of the new approach to answering one of the most pressing questions when natural disasters strike. The report concluded that it is now possible to attribute individual weather events to anthropogenic climate change. It is thus only in the last few years that the role of climate change in extreme weather events around the world has been assessed; before, only a few studies examined specific events.
The advancement of event attribution science, and the availability of much improved simulations of future changes in extreme events, allow us to look at specific events and regions and thus enable a growing understanding of how climate change is impacting natural and human systems at the local level. This is necessary, as a warming climate has a twofold impact on local weather. First, given the increase in greenhouse gases in the atmosphere and rising global mean temperatures, we expect the number of heatwaves to increase and the coldwaves to decrease. Similarly, because a warmer atmosphere can hold more water vapor, extreme precipitation will increase globally, on average. Indeed, both of these impacts have already been observed (IPCC 2012).
Second, locally, however, this can look very different. The extent of extreme precipitation and temperature increases varies, because the thermodynamic (warming) effect is not the only way in which climate change affects weather and extreme events. Higher levels of greenhouse gases in the atmosphere, combined with more water vapor and a changing land surface, alter the atmospheric circulation, which affects where and when weather systems develop and how they progress. This so-called dynamic effect can be the same size as the thermodynamic effect or larger, and it can act in the same direction, thus increasing the risk of extreme precipitation, for example, more than would be expected from high temperatures alone (Risser and Wehner 2017; van Oldenborgh et al. 2017). The effects could also counteract each other, and thus prevent changes in extremes (Schaller et al. 2014). Thus, there are a priori three possible ways in which climate change affects weather and extreme weather; it can: (1) increase the likelihood of an event occurring, (2) decrease the likelihood of an event occurring, or (3) not affect the likelihood of events and extremes (Otto et al. 2016). Event attribution studies thus allow us to assess which of these three cases applies to a given type of weather event in a particular region and season and quantify the change.
While all three of these impacts can be the result of anthropogenic climate change alone, anthropogenic greenhouse gases in the atmosphere are not the only external driver affecting the likelihood of extreme events. For example, an increase in heat events can be offset by a cooling trend induced by implementing large-scale irrigation systems that keep the soil wet and thus reduce the land-atmosphere feedback (van Oldenborgh et al. 2018).
Aerosols of anthropogenic and natural origin also have a large influence on extreme weather events. Depending on the type of aerosol, they either reflect sunlight (and thus lead to a cooling) or absorb long-wave radiation (and thus exacerbate the effect of greenhouse gases). However, these are only the direct effects. Aerosols also interact with clouds in various ways, making it extremely hard to estimate their exact influence on the climate system. On a global scale they do lead to a cooling, however (IPCC 2014). In contrast to greenhouse gases, aerosols have a very short lifetime, on the order of days or weeks depending on how high in the atmosphere they are emitted. If the effect of greenhouse gases in an extreme event is masked by local aerosol pollution, irrigation or other land use changes, the observed data will reveal no trend in extremes.
Thus, if observations are the basis of decision-making, these can be fatally wrong. Increasing global attempts to combat air pollution will soon cancel the masking effect. This of course also means that attribution studies that focus only on the attribution of current extreme events without putting them in the context of future climate change miss an important part of the story. Very few studies have examined changing hazards across timescales from the past to the present and future. For instance, in the IPCC’s fifth assessment report, detection and attribution (IPCC 2014) were discussed in a different chapter than projections of future climate change (IPCC 2014), and they used very different scales and methods of assessment. Future studies will address changing hazards, due to requests from city planners, disaster risk reduction experts, and other people involved in climate-change impacts and adaptation planning (van Aalst et al. 2018).
Thus, extreme event attribution allows us to assess the current impacts of climate change, in terms of weather as well as costs and ecological and societal implications; a comprehensive picture is slowly emerging, but there are huge gaps in the developing world. We also have the methodologies to combine this understanding with assessment of future climate change on geographical scales that matter for decision-making (e.g., Otto et al. 2018). However, while in theory it is possible to conduct an inventory of a large proportion of the changing hazards and extreme events taking place in a warming world, few studies have examined specific events in individual countries.
Regional Understanding
Bangladesh is in many ways the poster child of climate-change impacts (see Chapter 6, Bangladesh). On the one hand, its population is extremely vulnerable to flooding and saltwater intrusion (e.g. Warner and van der Geest 2013). Therefore, even small changes in the likelihood of extreme precipitation have a huge impact on the country’s exposure to flooding. On the other hand, Bangladesh’s scientists and government are acutely aware of this situation, and are exploring ways to deal with the impacts of climate change, including understanding and quantifying its impacts. For example, it was the first country to establish a national mechanism to address loss and damage from climate change—the costs (economic and otherwise) that mitigation and adaptation efforts have not avoided. While no articles have been published thus far that attribute individual extreme events in Bangladesh to anthropogenic climate change, a growing body of literature has confirmed that climate change increases the risk of damage from extreme events in a changing climate (Caesar et al. 2015; Ahmed, Diffenbaugh, and Hertel 2009; Karim and Mimura 2008).
Extreme weather events also occur elsewhere on the Indian subcontinent; each year it experiences droughts, floods, and heatwaves (Dash et al. 2007). In recent years many events have received high levels of international media attention because of heat records being broken (e.g., in Rajasthan in 2016, a large-scale heatwave in Andhra Pradesh in 2015) and large losses of assets (e.g., after massive flooding in Chennai in 2015), and of life (e.g., after exceptionally high monsoon rains in 2018). While there is little doubt that climate change plays a role in extreme weather events in India (Goswami et al. 2006), attribution studies of these recent events have shown that factors other than increased greenhouse gases continue to play a crucial role in driving extreme weather events over India (van Oldenborgh et al. 2018, 2016; Wehner at al. 2016). Natural variability as well as other man-made drivers like aerosol pollution play a particularly large role on small scales. At the same time, drivers outside the climate system, like river management, sewage water systems, and the sheer number of people in harm’s way determine to a large degree the impacts of changing weather and climate. Understanding how these risks are changing throughout the world requires disentangling these factors and assessing their future trajectories.
In India and elsewhere, information on these changing risks is lacking at a scale where it is needed most—in the most vulnerable regions, where events cause the greatest impacts, where climate change is increasing risk, and where the media and the general public are asking questions about the root causes of disasters and their own vulnerability. India has a strong climate science community and widespread awareness of the fact that changes in the frequency and intensity of extreme weather events and rising sea levels caused by human-induced climate change threaten decades of development gains, and pose a clear and present danger to the social and economic welfare of communities. The city of Ahmedabad in Gujarat is a good example of how awareness of the changing risk of extreme heat has led to the development of a heatwave plan. The plan, consisting mostly of awareness rising and simple behavioral advice when heat wave warnings are being issued, dramatically reduced hospital admissions for heat-related conditions during the most recent heat wave compared with the 2005 heatwave (Knowlton et al. 2014). With heat-related diseases on the rise in a warming climate (Glaser et al. 2016), this example indicates that access to information on the extent to which extreme weather events are influenced by climate change and other factors can save lives.
The science base in Northern Europe for localized extreme events is broader: a large number of studies have assessed the changing risk of extreme events and heatwaves in particular. But the awareness and preparedness of individual cities is similar to those of India, although vulnerability and exposure are of course very different (cf. Chapter 4, Germany). The region’s 2003 heatwave, which caused a reported 70,000 deaths (Donner, Muller, and Koppel 2015), demonstrated that preparedness is not a given in this part of the world (Aguiar et al. 2018). The event led the United Kingdom to develop a heatwave plan the following year, before the first attribution study (Stott, Stone, and Allen 2004) on this event was published. Subsequent heatwaves had less severe impacts, but all showed a clear attributable signal in likelihood and intensity to anthropogenic climate change (Christidis, Jones, and Stott 2014; Otto et al. 2012). Apart from heatwaves, an increase in extreme precipitation events is the most likely impact of anthropogenic climate change in Northern Germany. While there are no attribution studies on this specific area, given the similarity of the UK’s climate, the attributable increase in the intensity and frequency of winter rainstorms there gives a good indication of what can be expected in Hamburg (Otto et al. 2018b; Schaller et al. 2016). In the summer, the risk of extreme precipitation is not currently changing (Otto et al. 2015a; Schaller et al. 2014). These results are in line with projections of future precipitation extremes, which show a significant increase in the winter but no clear sign of an attributable change in likelihood or intensity in the summer (IPCC 2012). Much less studied are the impacts of anthropogenic climate change on windstorms, which often cause casualties. The latest generation of regional climate models is good enough to enable realistic simulations, but the lack of comprehensive and reliable observational data on these events prevents assessments of this potential impact of climate change.
Our knowledge and understanding of extreme weather and their changes in a changing climate is again different in East Africa. Droughts and floods are the extreme events that have the largest impacts in the region; droughts have been studied intensely in recent years. Over the last few decades, an observed drying trend in much of the region has provided a puzzle for the climate science community, as the observations are at odds with model simulations that generally predict a wetting trend. Rowell, Booth, Nicholson, and Good (2015) have recently made progress in understanding this apparent paradox from a modelling perspective. In addition, a large number of event attribution studies (Liebmann et al. 2017; Philip et al. 2017; Uhe et al. 2017; Funk et al. 2016; Marthews et al. 2015; Otto et al. 2015a; Otto et al. 2015b) have helped to disentangle some of the drivers behind the observed trend and to identify the relative importance of different drivers in individual droughts in recent years.
The main finding of these event attribution studies is that anthropogenic climate change is not a key driver of the lack of rain in East Africa in recent years. Drought is a complex phenomenon; the higher global temperatures have exacerbated the drought by increasing the risk of dry soil moisture (Philip et al. 2017) through increased evaporation (Marthews et al. 2015), or via teleconnection through higher ocean temperatures (Funk et al. 2016). Teleconnections are patterns of variability in ocean temperatures; where they are analyzed for East Africa, the predominant mode of variability is the El Niño Southern Oscillation (Indeje, Semazzi, and Ogallo 2000). Variability in Indian Ocean temperatures is also important. Different modes in these patterns have always been crucial to determining seasonal rainfall and the likelihood of drought or flood in East Africa. A key question is whether (and to what extent) climate change will affect these patterns. There is currently insufficient evidence to draw definite conclusions, but some studies show that a warming trend, in combination with strong El Niño events, may increase the number of droughts (Funk et al. 2018) and flood disasters (Li et al. 2016) in the future.
In East Africa, huge advances in our understanding of rainfall variability and climate model reliability have been made possible by the better access to observed data (Funk et al. 2015), and close collaboration with scientists from East African Met Services and universities and international scientists. Yet many questions remain, particularly regarding the role of temperature.
An increase in temperature can contribute to drier soils and thus exacerbate the risk of drought, an effect often referred to when aiming to establish a climate change link (Funk et al. 2016). However, particularly in already arid regions, temperature often only exacerbates evaporation when moisture is present. Compounding the meteorological effects, it has been shown that hotter than normal temperatures have accelerated forage and water depletion across most of the region’s pastoral and marginal agricultural areas (FEWS NET 2017), and thus identifying increasing temperatures as an additional stressor, which will have implications during future extreme heat and drought events, as well as for livelihood activities such as crop production (Republic of Kenya 2013).
East Africa has always experienced extreme weather events (see Chapter 5, Tanzania). But the fact that not all of the recent droughts were very extreme from a meteorological perspective highlights that it is high vulnerability that led to the severe societal impacts (Magnan et al. 2016).
In sum, the results generated by the nascent field of event attribution indicate that attribution studies around the world largely confirm our expectations—that a warming climate increases heatwaves and extreme rainfall events. The magnitude of this increase, however, varies widely by region and season, and local factors unrelated to climate change can prevent or decrease the risk of these extremes.
The examples above thus highlight that a heightened understanding of regional changes in individual types of extreme weather events facilitates preparation for all types of extreme weather. But if these advancements in natural science are not made relevant to those experiencing such weather and deciding how best to adapt, even perfect models and complete inventories of climate-change impacts will not prevent future loss and damage from extreme weather caused by climate change.
References
Aguiar, Francisca C., Julia Bentz, João M. N. Silva, Ana L. Fonseca, and Rob Swart. 2018. “Adaptation to Climate Change at Local Level in Europe: An Overview”, Environmental Science and Policy, 86: 38–63, https://doi.org/10.1016/j.envsci.2018.04.010
Ahmed, Syud S., Noah S. Diffenbaugh, and Thomas W. Hertel 2009. “Climate Volatility Deepens Poverty Vulnerability in Developing Countries”, Environmental Research Letters, 4.3: 34004, https://doi.org/10.1088/1748-9326/4/3/034004
Allen, Myles. 2003. “Liability for Climate Change”, Nature, 421.6926: 891–92, https://doi.org/10.1038/421891a
Allen, Myles, and William J. Ingram. 2002. “Constraints on Future Changes in Climate and the Hydrologic Cycle”, Nature, 419.6903: 224–32, https://doi.org/10.1038/nature01092
Bellprat, Omar, and Francisco Doblas-Reyes. 2016. ‘Attribution of Extreme Weather and Climate Events Overestimated by Unreliable Climate Simulations”, Geophysical Research Letters, 43.5: 2158–64, https://doi.org/10.1002/2015gl067189
Caesar, John, Tamara Janes, Amanda Lindsay, and Bhaski Bhaskaran. 2015. “Temperature and Precipitation Projections over Bangladesh and the Upstream Ganges, Brahmaputra and Meghna Systems”, Environmental Science: Processes and Impacts, 17.6: 1047–56, https://doi.org/10.1039/c4em00650j
Christidis, Nikolaos, Gareth S. Jones, and Peter A. Stott. 2014. “Dramatically Increasing Chance of Extremely Hot Summers Since the 2003 European Heatwave”, Nature Climate Change, 5: 46–50, https://doi.org/10.1038/nclimate2468
Dash, Sushil Kumar, Rajendra Kumar Jenamani, Shri S.R. Kalsi, and Subrat Kumar Panda. 2007. “Some Evidence of Climate Change in Twentieth-century India”, Climatic Change, 85.3: 299–321, https://doi.org/10.1007/s10584-007-9305-9
Donner, Julie, Juliana M. Muller, and Johann Koppel. 2015. “Urban Heat: Towards Adapted German Cities?”, Journal of Environmental Assessment Policy and Management, 17.2: 1550020, https://doi.org/10.1142/s1464333215500209
FEWS NET. 2017. “Kenya Food Security Outlook”, Early Warning of Food Insecurity, https://reliefweb.int/sites/reliefweb.int/files/resources/KENYA_Food_Security_Outlook_February%202020_final_0.pdf
Field, Christopher B., Vicente Barros, Thomas F. Stocker, and Qin Dahe. 2012. Managing the Risks of Extreme Events and Disasters to Advance Climate Change Adaptation (Cambridge, UK: Cambridge University Press), https://doi.org/10.1017/CBO9781139177245
Funk, Chris, Sharon E. Nicholson, Martin Landsfeld, Douglas Klotter, Pete Peterson, et al. 2015. “The Centennial Trends Greater Horn of Africa Precipitation Dataset”, Scientific Data, 2: 150050, https://doi.org/10.1038/sdata.2015.50
Funk, Chris, Laura Harrison, Shraddhanand Shukla, Diriba Korecha, Tamuke Magadzire, et al. 2016. “Assessing the Contributions of Local and East Pacific Warming to the 2015 Droughts in Ethiopia and Southern Africa”, Bulletin of the American Meteorological Society, 97.12: S75–S80, https://doi.org/10.1175/BAMS-D-16-0167.1
Glaser, Jason, Jay Lemery, Balaji Rajagopalan, Henry F. Diaz, Ramón Garcia-Trabanino, et al. 2016. “Climate Change and the Emergent Epidemic of CKD from Heat Stress in Rural Communities: The Case for Heat Stress Nephropathy”, Clinical Journal of the American Society of Nephrology, 11.8: 1472–83, https://doi.org/10.2215/cjn.13841215
Goswami, Bhupendra Nath, V. Venugopal, D. Sangupta, M.S. Madhusoodanan, et al. 2006. “Increasing Trend of Extreme Rain Events Over India in a Warming Environment”, Science, 314.5804: 1442–45, https://doi.org/10.1126/science.1132027
Haustein, Karsten, Myles Allen, Piers Forster, Friederike Otto, Daniel M. Mitchell, et al. 2017. “A Real-Time Global Warming Index’, Scientific Reports, 7.1: 15417, https://doi.org/10.1038/s41598-017-14828-5
Heede, Richard. 2014. “Tracing Anthropogenic Carbon Dioxide and Methane Emissions to Fossil Fuel and Cement Producers, 1854–2010”, Climatic Change, 122.1: 229–41, https://doi.org/10.1007/s10584-013-0986-y
Hegerl, Gabriele C., Stefan Brönnimann, Andrew Schurer, and Tim Cowan. 2018. “The Early 20th Century Warming: Anomalies, Causes, and ConsequencesW, WIREs Clim Change, 9.4: e522, https://doi.org/10.1002/wcc.522
Indeje, Matayo, Fredrick H. M. Semazzi, and Laban J. Ogallo. 2000. “ENSO Signals in East African Rainfall Seasons”, International Journal of Climatology, 20.1: 19–46, https://doi.org/10.1002/(SICI)1097-0088(200001)20:1%3C19::AID-JOC449%3E3.0.CO;2-0
IPCC. 2012. Managing the Risks of Extreme Events and Disasters to Advance Climate Change Adaptation: Special Report of the IPCC (Cambridge, UK: Cambridge University Press) https://www.ipcc.ch/report/managing-the-risks-of-extreme-events-and-disasters-to-advance-climate-change-adaptation/
——. 2014. Climate Change 2014 Synthesis Report: Contribution of Working Groups I, II and III to the Fifth Assessment Report of the Intergovernmental Panel on Climate Change (Geneva: IPCC), https://www.ipcc.ch/report/ar5/syr/
Karim, Mohammed F., and Nobuo Mimura. 2008. “Impacts of Climate Change and Sea-Level Rise on Cyclonic Storm Surge Floods in Bangladesh”, Global Environmental Change, 18.3: 490–500, https://doi.org/10.1016/j.gloenvcha.2008.05.002
Knowlton, Kim, Suhas P. Kulkarni, Gulrez Shah Azhar, Dileep Mavalankar, Anjali Jaiswal, et al. 2014. “Development and Implementation of South Asia’s First Heat-Health Action Plan in Ahmedabad (Gujarat, India)”, International Journal of Environmental Research and Public Health, 11.4: 3473–92, https://doi.org/10.3390/ijerph110403473
Li, Chan-juan, Yuan-qing Chai, Lin-sheng Yang, and Hai-rong Li 2016. “Spatio-Temporal Distribution of Flood Disasters and Analysis of Influencing Factors in Africa”, Natural Hazards, 82.1: 721–31, https://doi.org/10.1007/s11069-016-2181-8
Liebmann, Brant, Ileana Bladé, Chris Funk, Dave Allured, Xiao-Wei Quan, et al. 2017. “Climatology and Interannual Variability of Boreal Spring Wet Season Precipitation in the Eastern Horn of Africa and Implications for Its Recent Decline”, Journal of Climate, 30.10: 3867–86, https://doi.org/10.1175/jcli-d-16-0452.1
Lloyd, Elisabeth A., and Naomi Oreskes. 2018. “Climate Change Attribution: When Is It Appropriate to Accept New Methods?”, Earth’s Future, 6.3: 311–25, https://doi.org/10.1002/2017ef000665
Magnan, K., Lisa Schipper, Maxine Burkett, Sukaina Bharwani, et al. 2016. “Addressing the Risk of Maladaptation to Climate Change”, WIREs Climate Change, 7.5: 646–65, https://doi.org/10.1002/wcc.409
Marthews, Toby, Friederike E. L. Otto, Daniel Mitchell, Simon James Dadson, and Richard G. Jones. 2015. “The 2014 Drought in the Horn of Africa: Attribution of Meteorological Drivers”, Bulletin of the American Meteorological Society, 96.12: 83–88, https://doi.org/10.1175/bams-d-15-00115.1
Marvel, Kate, Michela Biasutti, Céline Bonfils, Karl E. Taylor, Yochanan Kushnir, et al. 2017. “Observed and Projected Changes to the Precipitation Annual Cycle”, Journal of Climate, 30.13: 4983–95, https://doi.org/10.1175/jcli-d-16-0572.1
Mitchell, Daniel, Clare Heaviside, Sotiris Vardoulakis, Chris Huntingford, Giacomo Masato, et al. 2016. “Attributing Human Mortality during Extreme Heat Waves to Anthropogenic Climate Change”, Environmental Research Letters, 11.7: 74006, https://doi.org/10.1088/1748-9326/11/7/074006
National Academies of Sciences, Engineering, and Medicine. 2016. Attribution of Extreme Weather Events in the Context of Climate Change (Washington, DC: The National Academies Press), https://doi.org/10.17226/21852
Otto, Friederike E. L. 2017. ‘Attribution of Weather and Climate Events’, Annual Review of Environment and Resources, 42.1: 627–46, https://doi.org/10.1146/annurev-environ-102016-060847
Otto, Friederike E. L., Neil Massey, Geert Jan van Oldenborgh, Richard G. Jones, and Myles R Allen. 2012. “Reconciling Two Approaches to Attribution of the 2010 Russian Heat Wave”, Geophysical Research Letters, 39.4, https://doi.org/10.1029/2011gl050422
Otto, Friederike E. L., Suzanne M. Rosier, Myles R. Allen, Neil R. Massey, Cameron J. Rye, et al. 2015a. “Attribution Analysis of High Precipitation Events in Summer in England and Wales over the Last Decade”, Climatic Change, 132.1: 77–91, https://doi.org/10.1007/s10584-014-1095-2
Otto, Friederike E. L., Emily Boyd, Richard G. Jones, Rosalind J. Cornforth, Rachel James, et al. 2015b. “Attribution of Extreme Weather Events in Africa: A Preliminary Exploration of the Science and Policy Implications”, Climatic Change, 132.4: 531–43, https://doi.org/10.1007/s10584-015-1432-0
Otto, Friederike E. L., Geert Jan van Oldenborgh, Jonathan Eden, Peter A. Stott, David J. Karoly, et al. 2016. “The Attribution Question”, Nature Climate Change, 6.9: 813–16, https://doi.org/10.1038/nclimate3089
Otto, Friederike E. L., Karin van der Wiel, Geert Jan van Oldenborgh, Sjouke Philip, Sarah F. Kew, et al. 2018. “Climate Change Increases the Probability of Heavy Rains in Northern England/Southern Scotland Like Those of Storm Desmond—a Real-Time Event Attribution Revisited’, Environmental Research Letters, 13.2: 24006, https://doi.org/10.1088/1748-9326/aa9663
Philip, Sjoukje, Sarah F. Kew, Geert Jan van Oldenborgh, Friederike Otto, Sarah O’Keefe, et al. 2017. “Attribution Analysis of the Ethiopian Drought of 2015”, Journal of Climate, 31.6: 2465–86, https://doi.org/10.1175/jcli-d-17-0274.1
Republic of Kenya. 2013. National Climate Change Action Plan 2013–2017 (Nairobi: Ministry of Environment and Mineral Resources), https://cdkn.org/wp-content/uploads/2013/03/Kenya-National-Climate-Change-Action-Plan.pdf
Risser, Mark D., and Michael F. Wehner. 2017. “Attributable Human-Induced Changes in the Likelihood and Magnitude of the Observed Extreme Precipitation during Hurricane Harvey”, Geophysical Research Letters, 44.24: 12,457–64, https://doi.org/10.1002/2017gl075888
Rowell, David, Ben Booth, Sharon Nicholson, and Peter Good. 2015. “Reconciling Past and Future Rainfall Trends over East Africa”, Journal of Climate, 28.24: 9768–88, https://doi.org/10.1175/JCLI-D-15-0140.1
Santer, Benjamin D., Stephen Po-Chedley, Mark D. Zelinka, Ivana Cvijanovic, Céline Bonfils, et al. 2018. “Human Influence on the Seasonal Cycle of Tropospheric Temperature”, Science, 361.6399: 245-56, https://doi.org/10.1126/science.aas8806
Schaller, Nathalie, Friederike E. L. Otto, Geert Jan Van Oldenborgh, Neil R. Massey, Sarah Sparrow, et al. 2014. “The Heavy Precipitation Event of May–June 2013 in the Upper Danube and Elbe Basins”, American Meteorological Society, 95.9: S69–S72, https://pdfs.semanticscholar.org/b7f3/90dab80a8363a3ef415e601df5a63800b6ac.pdf
Schaller, Nathalie, Alison L. Kay, Rob Lamb, Neil R. Massey, Geert Jan van Oldenborgh, et al. 2016. “Human Influence on Climate in the 2014 Southern England Winter Floods and Their Impacts”, Nature Climate Change, 6.6: 627–34, https://doi.org/10.1038/nclimate2927
Skeie, Ragnhild B., Jan Fuglestvedt, Terje Bernsten, Glen P. Peters, Robbie Andrew, et al. 2017. “Perspective Has a Strong Effect on the Calculation of Historical Contributions to Global Warming”, Environmental Research Letters, 12.2: 24022, https://doi.org/10.1088/1748-9326/aa5b0a
Stott, Peter A., Nikolaos Christidis, Friederike E. L. Otto, Ying Sun, Jean-Paul Vanderlinden, et al. 2016. “Attribution of Extreme Weather and Climate-Related Events”, WIREs Climate Change, 7.1: 23–41, https://doi.org/10.1002/wcc.380
Stott, Peter A., Dáithí A. Stone, and Myles R. Allen. 2004. “Human Contribution to the European Heatwave of 2003”, Nature, 432.7017: 610–14, https://doi.org/10.1038/nature03089
Uhe, Peter, Sjoukje Philip, Sarah Kew, Kasturi Shah, Joyce Kimutai, et al. 2017. “Attributing Drivers of the 2016 Kenyan Drought”, International Journal of Climatology, 38: e554–68, https://doi.org/10.1002/joc.5389
van Aalst, Maarten, Richard Jones, Allan Lavell, Hans-Otto Pörtner, Debra Roberts, et al. 2018. Bridging Climate Science, Policy and Practice. Report of the International Conference on Climate Risk Management, Pre-Scoping Meeting for the IPCC Sixth Assessment Report (The Hague: IPCC), https://www.climatecentre.org/downloads/files/RCCC%20IPCC%20Nairobi%20Report%202018-4%20V5.pdf
van Oldenborgh, Geert J., Friederike E. L. Otto, Karten Haustein, and Krishna Achuta Rao. 2016. “17. The Heavy Precipitation Event of December 2015 in Chennai, India”, Bulletin of the American Meteorological Society, 97.12: 87–90, https://doi.org/10.1175/bams-d-16-0129.1
van Oldenborgh, Geert J., Karin van der Wiel, Antonia Sebastian, Roop Singh, Julie Arrighi, et al. 2017. “Attribution of Extreme Rainfall from Hurricane Harvey, August 2017”, Environmental Research Letters, 12.12: 124009, https://doi.org/10.1088/1748-9326/aa9ef2
van Oldenborgh, Geert J., Sjoujke Philip, Sarah Kew, Michiel van Weele, Peter Uhe, et al. 2018. “Extreme Heat in India and Anthropogenic Climate Change”, Natural Hazards and Earth System Sciences, 18.1: 365–81, https://doi.org/10.5194/nhess-18-365-2018
Warner, Koko, and Kees van der Geest. 2013. “Loss and Damage from Climate Change: Local-Level Evidence from Nine Vulnerable Countries”, International Journal of Global Warming, 5.4: 367–86, https://doi.org/10.1504/ijgw.2013.057289
Wehner, Michael, Dáithí Stone, Hari Krishnan, Krishna Achuta Rao, and Federico Castillo. 2016. “The Deadly Combination of Heat and Humidity in India and Pakistan in Summer 2015”, Bulletin of the American Meteorological Society, 97.12: S81–S86, https://doi.org/10.1175/bams-d-16-0145.1