10. The Role of Ontogeny in Understanding Human Demographic Behaviour
© 2024 Paula Sheppard and David A. Coall, CC BY 4.0 https://doi.org/10.11647/OBP.0251.10
Ontogeny, the development of an organism from conception to maturity, is one of Tinbergen’s two proximate explanations for understanding why we do the things we do. As well as genetic inheritance, the developmental environment, to which parents make a large contribution, is crucial for shaping a child’s life. It not only shapes their physical and psychological development, but also influences the adult child’s reproductive strategy and ultimately their life expectancy. Demographers care about fertility and mortality, which, when understood within an evolutionary framework, are two entwined processes that influence, and are in turn influenced by, the individual’s developmental trajectory. Here, we provide a summary of how development operates throughout life: from the womb, through childhood, adolescence and puberty, the reproductive years through to menopause and death. We take a life history approach with a focus on how developmental influences during early life have long-reaching consequences for mortality and fertility. We illustrate each section with theoretical advances, empirical examples and evaluation of the current literature. We hope to demonstrate that thinking about human demographic behaviour can be revealing in light of ontogeny, and provide a useful theoretical basis for demographic research.
Introduction
Ethology is the study of animal behaviour, starting with the assumption that organisms behave in ways that are adaptive (i.e. that enhance reproductive success), given individual and environmental constraints. Human behaviour is complex and includes a much richer social environment than other animals, but the same ethological principals can be applied. Niko Tinbergen, a Nobel-prize-winning ethologist, conceptualized an insightful multifaceted approach to understanding animal behaviour (Tinbergen, 1963). He argued, following Ernst Mayr’s (1961) teleology, that any ethological question could be answered at two levels — the ultimate (why a trait exists) and the proximate (how a trait operates), and each can be split in two components: phylogeny and function are ultimate explanations, and ontogeny and mechanism are proximate explanations. These four levels of explanation provide a framework for understanding behavioural traits.
Broadly, an ultimate explanation describes a phenomenon at its evolutionary, or adaptive, level — i.e. what is its function, and how did it evolve (phylogenetic). Proximate explanations are the more immediate or direct reasons which can be understood in terms of the mechanism(s) or triggers of the behaviour, as well as by the organism’s development (ontogeny) as an individual. For example, if we were to wonder “why do bears hibernate?”, four explanations can be given, and all would be correct. At the proximate level, bears hibernate because it is winter, food is scarce, and they feel cold (these are mechanisms). The propensity to hibernate is partly an innate trait, and partly learned by bear cubs denning with their mothers for the first few winters of their lives (this is the ontogenetic explanation). The ultimate explanations are that bears hibernate because it is adaptive for them to conserve energy and drop body temperature to survive harsh winters when food is scarce, improving their survival and reproductive chances — ancestral bear species who did hibernate were selected for — i.e. hibernation improves a bear’s survival and reproductive chances. The phylogenetic explanation for bear hibernation is that bears are part of the Ursine family and hibernation is part of their evolved behavioural repertoire.1 Tinbergen’s framework remains a hugely useful paradigm for thinking about animal and human behaviour, and although a more modern usage might include supplementary questions to provide fuller explanations (Bateson and Laland, 2013), the proximate/ultimate distinction is the foundation of any evolutionary understanding of behavioural traits.
Here we focus on the role of ontogeny (or development2) in understanding human demographic behaviour. Development is partly about traits that develop in the womb through to puberty and reproductive maturation (at least in mammals), but also refers to learned behaviours, which are especially important during childhood. Demographers are interested in three main facets of human behaviour, namely fertility, migration, and mortality.
Following on from the previous example, we can apply Tinbergen’s four levels of explanation to a demographic question. For instance, “why do humans live so long?”. Phylogenetically, humans are primates, which all live relatively long for their body size, and, in fact, among primates humans have the longest lifespans and slowest life histories. Long lifespans are adaptive (functional) for cooperative breeding (sharing childrearing duties among kin and non-kin), which allowed for the human trait of raising numerous “stacked” dependent offspring at a time (Bogin, 1998). The mechanisms for longevity are complex but may include the neural and hormonal responses triggered by caregiving that reduce stress and accentuate physiological health, leading to longer, healthier lives (Hilbrand and others, 2017). The ontogenetic explanation is that we gestate our young for nine months but give birth to highly altricial neonates (underdeveloped newborns compared to other primates) requiring prolonged periods of infancy in which highly dependent suckling babies mature. Human childhoods are also long compared to other primates, who only have a short juvenile growth period between weaning and adulthood (Hawkes, 2003). Human children enjoy many childhood years in which they can indulge in important social learning and skill acquisition with very low costs as they are still fed and protected by their parents. These longer early life stages are part of a suite of life history characteristics particular to humans (Kuzawa and Bragg, 2012; Bogin, 1999).
Theoretical Concepts
Life History Theory
We adopt a life history approach, focusing both on the human life stages prior to adulthood, as well as briefly addressing the ontogenetic process during later life, including old age, menopause and death. Ontogeny does not only refer to early development; it is relevant to the entire life course. The two demographic topics most relevant to ontogeny are fertility and mortality, and they are integral to understanding life history theory (although there is some evidence that migration can also affect human developmental trajectories (Núñez-De La Mora and others, 2007)).
Life history theory (LHT) is an evolutionary biology framework which can be used to explain the timing of human life events pertaining to growth, maintenance and reproduction at both the species and the individual level. The basic premise of LHT is that organisms make decisions about how best to allocate limited resources towards survival, growth and development, and reproduction in a bid to enhance their reproductive success, and thus their evolutionary “fitness” (genetic legacy) (Stearns, 1992; Kuzawa and Bragg, 2012). The fact that resources are limited leads to life history trade-offs; for instance, nutrition apportioned to growth cannot also be used for reproduction, which explains why organisms stop growing when they reach reproductive maturity. At the species level, the life histories of long-lived animals (including humans) are characterized by large body size, long gestation periods, production of relatively few offspring who remain dependent on the parent(s) for a long time (i.e. have extended childhoods), and relatively low early-life mortality. This suite of traits is typical of a slow life history species, and humans are among the slowest. This being the case, there is also plenty of variation among humans. Individuals who live in harsh environments with lower resource availability tend to live shorter lives than those from more affluent settings. Infant mortality is higher in harsh conditions, leading to higher rates of reproduction to offset this risk to lineage extinction (Chisholm, 1993). The relationship between mortality and fertility provides an ultimate, adaptive explanation for much of human reproductive behaviour.
Plasticity and prediction
Phenotypic plasticity is also important for understanding the basics of how development operates. The phenotype is the observable expression of the individual’s genetic potential, as shaped by the environment; phenotypes are the various physical and behavioural features of the organism — each trait, such as height or hair colour, is a phenotype. There is a difference between what is genetically inherited, and what is produced by that heredity. On average an individual inherits half of his or her genes from each parent, and together these constitute the individual’s unique genotype; all of our cells have the same genotype (except sex cells), but not all the genes are expressed in each phenotype, although they have the potential to be, and they can be passed on to the next generation. Phenotypic plasticity refers to the adaptive process that allows an organism to make flexible “choices” about how to behave or react to certain environments3 (Pigliucci, 2005; Fusco and Minelli, 2010). In other words, any genotype can express different phenotypes depending on the environment. This flexibility means we have the potential to express an array of behaviours around a given trait (i.e. adopt a strategy) and the environment we find ourselves in triggers one or more responses. Because this flexibility has been shaped through evolutionary history, and those individuals who could be flexible were more likely to survive and reproduce, plasticity is expected to be adaptive.
Insofar as developmental plasticity promotes Darwinian fitness, it also comes at a cost that can have negative health consequences in humans (Wells, 2019). There are conflicting views as to how the relationship between the environment and genotype works exactly. The concept of a predictive adaptive response (PAR) is that, given the environment in the organism’s early development (usually in the womb for mammals), the organism programs its strategy and then sticks to it (developmental programming). The assumption is that early environments are highly indicative of future environments; therefore, a phenotype that aligns with an early environment that remains stable is assumed to be adaptive (Gluckman and others, 2005). For humans, however, this is an unlikely scenario as a great deal can change during a lifespan of fifty to eighty years.
Throughout hominid evolution, environmental instability has been predominant. Even ancestral humans would have encountered variability in environments due to climatic fluctuations and migration (Foley, 1995; Potts, 1998). A recent reconsideration of how plasticity operates has focused on internal prediction. This argument stresses that early environmental conditions can leave a mark on the physical or mental health of the individual (Nettle and others, 2013). If the environmental assault is serious enough, the mark it leaves can impact on the individual’s lifespan by increasing the likelihood of premature death through a higher susceptibility to disease, or high levels of psychological stress. The individual’s strategy is then calibrated accordingly. In this case, there is no need to predict the future environment; the impact of early environments is embodied, carried within the person (Rickard and others, 2014). In the context of life history theory, under harsh environments the priority is simply to survive, therefore, physiological changes during gestation in response to a challenging environment is “making the best of a bad start”, not adapting for the future (Berghänel and others, 2017; Jones, 2005; Vitzthum, 2001; Rickard and others, 2014).
We next describe how ontogeny influences demographic traits (here, fertility and mortality) with the underlying assumption that humans react and behave flexibly in response to a combination of their genetic endowment and the environmental conditions within which they develop and mature.
Prenatal Environment and Development
Prenatal development focuses on the early stages of the life cycle, our most precarious time of life; it is the time of most rapid development, and thus the time of highest risk. Prenatal development, the development of a new life, is also where the parents’ fecundity (the physiological ability to reproduce) is translated into fertility (the number of offspring) and ultimately reproductive success. The impact of this life stage, however, extends far beyond reproduction. The prenatal period is when many environmental and physiological factors, working through the mother, impact a foetus and have consequences for subsequent development. From the perspective of life history theory, foetal growth is a measure of the resource flow to the foetus at the expense of others (parental investment). Thus, foetal growth also represents the non-genetic intergenerational transmission of phenotypes. The prenatal environment has the potential to increase the fit between the offspring’s phenotype and its environment, which can provide an evolutionary advantage by increasing the probability of survival and reproduction. It is therefore likely that natural selection would favour foetal sensitivity to maternal behaviour and physiology. In the broader ethology and biology literature, these are referred to as maternal effects (Maestripieri and Mateo, 2009).
Myriad factors influence pregnancy and the vast majority of non-genetic elements work through the mother’s phenotype (Wells, 2010). These include but are not limited to maternal stress, prenatal nutrition, environmental toxins and teratogens, antenatal care, prenatal bonding and early psychosocial environments (Coall and others, 2019). Across mammalian species, due to internal fertilization, gestation and lactation, interactions between the mother and offspring are close and of extended duration. Thus, mothers have the most profound influence on their developing offspring’s phenotype throughout pregnancy. The mechanisms by which the maternal phenotype can affect the foetus’s phenotype include her behaviour and hormone levels, nutrition, mental and physical health and size. For example, across mammalian species, smaller mothers tend to produce smaller offspring, with the most influential processes beyond genetics being other constraints such as the first pregnancy, smaller pelvic outlet or reduced nutrient supply to the foetus (Gluckman and Hanson, 2004; Godfrey and others, 1999)
Life history theory examines the relationship between the environment and life cycles from an evolutionary perspective. Therefore, foetal growth is one phase of the life cycle that is heavily influenced by the mother’s own life cycle. An example of this is the role of maternal constraints — there are limits on how large a baby can be — influencing foetal growth and potentially that of future generations. Indeed, in a study of 513 low-risk pregnancies, maternal birth weight was the only factor that consistently predicted children’s foetal and placental growth, affecting outcomes including birth weight, placental weight, placental ratio, placental surface area and placental thickness (Coall and others, 2009). Within these constraints, the idea that maternal birth weight is among the strongest predictors of her offspring’s birth weight and provides a “better” reflection of the likely nutrition environment over generations, rather than the nine months of pregnancy, is referred to as “intergenerational phenotypic inertia”.
Kuzawa’s (2005) intergenerational phenotypic inertia model provided an adaptationist rationale for expecting the effects of prenatal malnutrition or stress to last more than one generation: when environments are stochastic over time scales greater than a generation, nine months of gestation cannot provide the foetus with enough information upon which to “predict” its own within-generation optimal growth and development. Ultimately, possibly through the epigenetic regulation of growth factors (IGF2), intergenerational phenotypic inertia provides the foetus with information, not only about the environment into which it will be born, but also about the environment into which its mother was born, and perhaps even its mother’s mother, and so on, back through an unknown number of generations. Such inertia reduces the impact of short-term variations in nutrition, allowing the broader nutritional environment to influence foetal growth. Thus, associations between foetal development and adult health risks seen in the “Developmental Origins of Health and Disease” literature may reflect longer time frames than the nine months of gestation.
Within life history theory, because the amount of resources available is always limited (e.g. time, energy) there are trade-offs between the components of fitness, and the most all-encompassing trade-off is that between current and future reproduction (Stearns, 1992). At issue is whether it would be better for an organism’s lifetime reproductive success to reproduce at a given time or to wait for another opportunity in the future. The major determinants of the optimal current-future trade-off are (1) the probability of death at a given age, and (2) the availability of energy and other resources that determine parents’ capacity to invest in offspring (e.g. foetal growth). When environmental conditions are risky or uncertain, with high or unpredictable mortality rates and few or uncertain resources, organisms in general, including humans, tend to reproduce early and often, maximizing the probability of reproducing, but reducing the investment in each offspring (Coall and others, 2016). Conversely, when environmental conditions are safe and predictable with low and stable mortality rates and plentiful resources, organisms tend to reproduce later and less often, investing more resources in fewer offspring. Through their phenotypic plasticity, our ancestors were able to take advantage of good times by maximizing future reproduction (investing more in fewer offspring), and to cope with bad times by maximizing current reproduction (investing less in more offspring). None of this, of course, requires conscious awareness, and the world’s most disadvantaged peoples still tend to reproduce early and often (Low and others, 2009). What becomes apparent though is that the trade-off between current and future reproduction means that foetal adaptations to the effects of environmental stress on the mother can have evolutionarily adaptive consequences for her (future reproduction), but developmentally disadvantageous effects on foetal growth and development and thus postnatal health (maternal effects).
Childhood Influences on Fertility
Human childhoods are unusually long compared to other primates (Bogin, 1998), allowing for an extended period of growth and learning, while still largely dependent on care-givers for nutrition, safety, and shelter. The developmental environment during childhood can have far-reaching consequences; family settings that are nurturing and facilitate child growth and development are associated with slower reproductive strategies, while difficult childhood settings might instead increase the pace of life and initiate earlier reproduction and possibly higher fertility. Empirical studies support this view, although only in so-called WEIRD4 (Henrich and others, 2010) contexts where childhood psychosocial stressors often have a greater impact than nutrition (Sear and others, 2019). In more resource-stressed conditions, children whose early lives are nutritionally deprived will more likely delay reproduction in aid of growth.
It is arguable that early childhood (up to age 5–7 years) is a particularly critical period that sets the child’s reproductive trajectory (Belsky and others, 1991; Ellis, 2004), at least in WEIRD contexts. The empirical evidence tends to focus on girls and on age at puberty (often only menarche) as an outcome.5 Of these studies, those that separate early childhood from older childhood show a mixture of patterns. For instance, “harsh” parenting was associated with earlier age at puberty in a contemporary US sample, but this relationship was seen in both early and later childhood, and only for girls; boys appeared to be unaffected by harsh parenting, at least with regard to puberty (Belsky and others, 2007). Indeed, we might expect different effects for boys and girls; we discuss this in more detail in the next section. Also in the US, Quinlan (2003) found that parental separation during childhood was associated with earlier puberty, first voluntary sexual intercourse and first births for women. He tested for differences depending on which childhood stage the separation occurred, and although there were always earlier timed events compared to women who had had intact families since birth, the later the separation happened the weaker the association was.
In Malaysia, a country with a transitioning rate of fertility, and a lower economic setting than the US, Sheppard and others (2014), using father absence as a proxy for adverse childhood environments, report no association with timing of menarche in either early (before age 7) or later childhood. They did, however, find a statistically significant association between father absence and younger age at first birth, but only if the father became absent during later childhood (age 8–15). This might be explained by the different meanings “father absence” has in different cultural and economic contexts. These studies suggest that, while it is likely that childhood developmental ecologies have an impact on fertility-related outcomes, there might not be a critical early period in this respect. Generalizations like this should be made with caution as we cannot make true comparisons when childhood adversity is operationalized differently in each study, and they all use different methods. It might be more fruitful to think of developmental environments as sums of their parts where different types of adversity affect children differently and, depending on the cultural context, may not be indicative of adversity at all. For instance, the concept of “father absence” varies depending on the local norms regarding marriage and families (Sear and others, 2019). The absence of a father may be less of an adverse condition where extended families are more common and other alloparents can compensate, rather than the typical nuclear family found in WEIRD social settings.
Adolescence and Puberty
Adolescence bridges childhood and adulthood, and is mainly characterized by puberty. As with our relatively long childhoods, adolescence is purported to be the time when we hone our social skills further, and mentally and physiologically prepare for independence in adulthood (Sapolsky, 2017). Puberty is a key milestone in adolescence and is the physiological gateway to reproduction. Genetic, physiological (e.g. nutrition) and social (e.g. psychosocial stress) factors are known to influence pubertal timing. All children need to attain a certain body size (height and weight) and reach critical hormonal thresholds in order to successfully undergo this life transition (Ellison and others, 2012). There is usually a growth spurt that occurs during early adolescence which slows down as the pubertal stage commences. There is another growth spurt toward the end of adolescence and then continued growth to final adult height. All of these processes are fuelled by nutrition and exercise. However, they are also susceptible to psychosocial stressors, largely because stress hormones impact on growth and muscle mass, and negatively affect mental and emotional states (Ellis, 2004). Indeed, chronic psychosocial stress and high levels of cortisol resulting from disrupted environments may help to entrain adaptive life history strategies (Finch and Rose, 1995).
The timing of puberty is partially associated with the timing of first sex, the birth of the first child and reproductive lifespan, at least in women. Early maturers are more likely to have sex at a younger age, have earlier pregnancies and experience later cessation of reproduction (i.e. the start of menopause) compared with late bloomers (Coall and others, 2016). This suggests that the timing of menarche is a fair indicator of the pace of the reproductive strategy being pursued. Faster life history strategies, of which early reproduction is a component, are also associated with higher mortality (Nettle, 2010). For men, however, the story is not quite as clear, and the evidence much scanter than for women (Sheppard and Sear, 2012; Bogaert, 2005). Figure 1 illustrates how sex differences in human life histories might operate. Using data from the United States, Sheppard and others (2015) show two different life history trajectories between early childhood disruption, age at puberty and final adult height (an indicator of growth during childhood and puberty), for boys and girls. For girls, weight gain happens faster and puberty starts at a younger age, while for boys growth is slower and puberty is delayed — nonetheless, in both cases, childhood disruption ultimately leads to reduced growth and shorter adult stature.
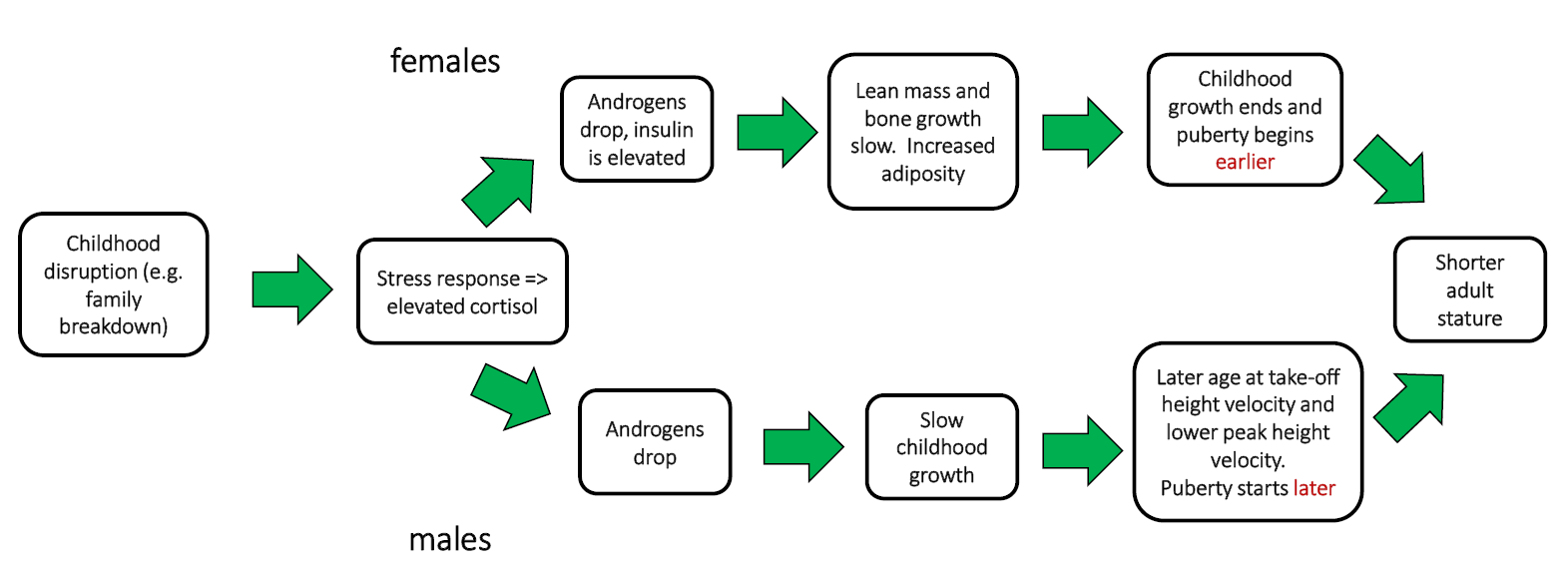
Fig. 1 Sex differences in stress responses to childhood disruption in high-income settings; different pubertal and growth pathways. All rights reserved.6
Demographers tend to focus on female fertility and largely ignore male fertility behaviour, but evolution predicts that men and women will not behave in the same way, and so a fuller understanding of demographic processes, especially around fertility, can only be attained by considering both sexes.
One associated adolescent trait that deserves a brief mention here is risk-taking. Risk-taking is most commonly found among adolescent boys until young adulthood (or even later). Although it is not only a male trait, it is much less frequently observed in girls (however, girls certainly can and do take risks too (Cross, 2010)). From an evolutionary standpoint, risk-taking is often thought to arise as an outcome of male-male competition (Chisholm, 1999). These are high-risk (e.g. failure, injury, death) high-payoff (reproductive and/or parenting opportunities) reproductive strategies. This behaviour is especially prominent during adolescence when young men are preparing for adulthood.
After men become fathers their testosterone levels recede as they turn to parenting rather than mating behaviour (Gettler and others, 2011). Young men want to have sex and will compete to attract young nubile women. It is a dual-purpose strategy: there is competition with other males (such as outwitting each other or simply fighting), and also showing off prowess and skill to the sought-after females. There are, by definition, costs (sometimes very high) to risk-taking. The whole point of the risk is that if it goes wrong, it can cost the young man’s life, result in injuries, or at the very least, cause embarrassment and the loss of status. Risk-taking requires the careful balance of risk; it needs to be risky enough to be impressive (ultimately increasing reproductive success), but not so risky that it results in no fitness legacy at all. Accidents happen when the risk is miscalculated, or unknowns are not factored in. Demographers who are interested in male-biased sex ratios and male mortality more generally would do well to understand male risk-taking strategies (Schacht and Borgerhoff Mulder, 2015). Seldom is the relationship between mortality and fertility more conspicuous than when witnessing male-male competition for females. Note that risk-taking is not the only male competition strategy available; not all young men fight and show off, at least not in life-threatening ways. Some men are more suited to other tactics such as displaying good parenting skills, being a good provider, exhibiting creative talents, etc. The strategy adopted will depend on the young man’s resources, both physical and material (i.e. his environment).
Demographers are often interested in the differences in fertility and mortality rates between countries within the context of the demographic transition. In high-income, low-fertility countries, the constraints are different to those settings where nutrition and other basic needs for survival are prioritized. Where access to sufficient good-quality nutrition is scarce, as is often the case in low-income countries, the basic weight requirements to enable pubertal processes to begin are limited by food stress. As such, girls from these areas tend to have later pubertal development than girls from high-income settings where the opposite is true. In high-income environments, socioeconomically disadvantaged girls have more access to highly calorific nutrition (junk food) which might not be healthy, but leads to rapid weight gain and early onset of puberty (Coall and others, 2016).
The association between psychosocial stress and pubertal timing has been shown empirically from many studies in so-called Western cultures. Due to meagre evidence in other settings, it is much less clear how this association works in lower-income settings where nutrition is a more salient problem. Puberty data are difficult to collect and have not been the focus of many longitudinal studies in less affluent countries, which limits research in this area. Nevertheless, there are a few studies which provide some evidence for a different trend in low- and middle-income contexts (Sear and others, 2019). In Malaysia, young women from father-absent homes were found to commence puberty (measured as first menstruation) no earlier than those from dual parent families (although they did marry younger) (Sheppard and others, 2014). It is likely that the mechanism driving the association between family background and age at puberty in lower income contexts has more to do with paternal provisioning (household resources) than in higher-income settings, where psychosocial stress is more of a problem than resource stress. Along similar lines, no evidence to support the hypothesis was found for young women in South Africa who were raised in father-absent families, compared with those where the father was present until age six and age at menarche (Anderson, 2015). There was, however, a significant association between father absence and age at first sex and pregnancy. These findings highlight the importance of understanding how contextual factors inform hypotheses derived from theories of human behaviour, such as life history theory.
Reproduction and Fertility
In demography, fertility is defined as the number of offspring an individual produces in a lifetime, and is usually measured for women. The total fertility rate (TFR) is a population-level measure of the average number of babies born to women of reproductive age (usually 15 to 49 years) in a given population. In addition to earlier declines in mortality and fertility, fertility in Europe has fallen dramatically over the last fifty years, along with decreasing mortality rates. This process is known as the “demographic transition to low fertility”, and is thought to be driven by economic development, as there is a strong correlation between country-level economic development and reduced fertility worldwide. Worldwide fertility has dropped from around five babies per woman in 1960 to half of that in 2015 (The World Bank 2018). In OECD member states (mostly high- and middle-income countries), the TFR has shifted from 3.2 to 1.7 in the same period, i.e. below replacement level (for the population to remain stable the TFR would need to be 2.1).
Demographers spend much research effort trying to understand this transition. It is a very well-described phenomenon, but not fully understood in terms of why it occurs. Evolutionary theorists also try to make sense of the counterintuitive pattern that apparently higher wealth is associated with lower fertility, when Darwinian reasoning would predict more resources equated to more offspring, and thus increased genetic fitness. The story is complicated and somewhat beyond the scope of this chapter, but part of the problem is due to the conflation of population measures with individual level outcomes (Borgerhoff Mulder, 1998; Mattison and Shenk, 2019). In other words, not all people from rich countries are in fact rich, and when considering individual-level wealth, the relationship is actually non-linear, with the very wealthy and the very low-socioeconomic groups both exhibiting relatively high fertility. This is explained by the observation that mortality also varies by socioeconomic status. Poorer people, even in relatively wealthy settings, tend to have lower life expectancy than more advantaged groups — even in London there is a more than twenty-year gap in life expectancy across neighbourhoods (Cheshire and O’Brien, 2015). In such environments it pays, in fitness terms, to produce more offspring earlier to offset the higher risk of death.
How can applying Tinbergen’s ontogenetic reasoning help us to understand fertility? A life history approach can help elucidate how early life conditions impact on the timing of first births, the timing of higher parity births, the decision to reproduce at all, and how many children to have in total. Despite the rather large body of evidence available on the developmental antecedents of puberty, there is relatively little on how development affects fertility (Coall and others, 2016). Furthermore, the evidence that we do have is not consistent across studies. Using data from the UK 1958 birth cohort, (Nettle and others, 2011) showed that women whose childhoods included: low paternal involvement; being breastfed for only a short while; frequent household moves; and being separated from their mothers had their first pregnancies at younger ages than women from non-disrupted backgrounds. Also, the more adverse conditions a woman had experienced, the younger the age at pregnancy (i.e. the effects are accumulative). Another study in the UK also found that poor childhood health was associated with earlier first births, even after accounting for the socioeconomic position of the family during childhood, and the women’s education (Waynforth, 2012). These studies looked at the timing of reproduction rather than total reproductive output or fecundity; other studies that do investigate markers of fertility have not been able to replicate these associations. Two studies from the US found that women who experienced adverse childhoods had irregular menstrual cycles, menstrual amenorrhea and difficulty conceiving (Jacobs and others, 2015; Allsworth and others, 2007).
While pubertal timing is certainly linked to reproductive timing, it is not the whole story. People’s life history trajectories are not determined from early life, and our evolved ability to adjust our responses to the environment in flexible ways means that these relationships are only partly co-dependent, and partly independent processes (Bornstein, 1989). In other words, childhood environments may mediate the relationship between people’s adult environments and their reproductive decisions. Indeed, the early environment does not “program” development, and only some who endure stressful childhoods go on to adopt an accelerated strategy of reproductive timing. Research using the 1958 UK birth cohort showed differences in reproductive timing between never- and ever-married women: early life adversity was associated with earlier pregnancy in never-married women, but delayed pregnancy in married women (Harville and Boynton-Jarret, 2013). Evolutionary demographers interested in fertility should consider the complex relationships throughout the life course, and be aware of likely mediating and moderating factors between early life, adult environments and childbearing (Coall and others, 2016).
What are the mechanisms? Early life and eventual reproduction are far apart in an individual’s lifespan. If childhood experiences influence fertility decision-making, then how does this operate? One idea is that having more children is a “predictive adaptive response” (PAR) to early childhood stress. The main problem with this argument is that it assumes that childhood conditions are good predictors of future ones. In other words, that we live (and have always lived) in environments that change little over the life course. This is implausible. Another hypothesis is that early life adversity has a tangible impact on that person’s health and that this is carried throughout life (e.g. stress reactivity, weight gain, growth), and is associated with higher mortality. Empirical evidence has so far revealed frustratingly little. In a longitudinal study of women born in Newcastle-upon-Tyne in 1947, low socioeconomic status (SES) and poor housing conditions at birth were both associated with younger age at first birth, and poor housing (though not low SES) was associated with higher completed fertility. Given the long duration between birth and the woman’s reproduction, a number of potential mediating factors were tested, such as birth weight, childhood illness, age at puberty, etc., but these had little influence on either reproductive outcome. Further, consistent with the idea of a life history strategy, when both outcomes were included in the model, age at first birth completely mediated the relationship between poor housing and total fertility (Sheppard and others, 2016). This is a useful investigation; however, it was a small study from a non-representative population — larger studies of these processes will be more revealing. Similarly, early research looking at telomere length as a marker of health in humans also does not reveal much — a review of the literature finds only a weak correlation between early life adversity and telomere length (shortened telomeres are a sign of ageing) (Pepper and others, 2018).
Few empirical studies have examined the relationship between childhood conditions and fertility outcomes in pre-demographic transition societies, and those that do report mixed results. A commonly-used indicator of childhood insecurity is father absence, and is often the focus of demographic studies among foraging peoples. For instance, the death of a father had no impact on fertility outcomes for sons or daughters among either the Venezuelan Ache or the Bolivian Tsimane foragers (Hill and Hurtado, 1996; Winking and others, 2011). Among the Belizean Maya and Paraguayan Ache males, however, father absence due to divorce was associated with delayed age at reproduction, possibly because father-absent sons were deprived of paternal investment leading to difficulty acquiring mates (Waynforth and others, 1998).
Menopause and Mortality
Menopause marks the end of a woman’s reproductive years, and is of substantial interest to demographers because of the associated reduction in female mortality advantage after menopause, changes in disease risk profiles (Hill, 1996) and its inverse association with all-cause mortality (Jacobsen and others, 2003). At the same time, menopause is grounded in ontogeny. Menopause is defined as one year after a woman’s final menstrual period and results from the gradual depletion of ovarian follicles that begins during foetal life and is complete at menopause. Therefore, events during development, such as age at sexual maturity, pregnancy, childbirth, contraceptive use and number of offspring influence the number of ovulatory menstrual cycles, the rate of ovarian follicle loss, and the length of reproductive lifespan (Bjelland and others, 2018; Gold and others, 2001). Several of these life history events have been associated with age at menopause.
Human life histories are characterized by a long lifespan and, particularly in women, a long post-reproductive lifespan after menopause. Originally, evolutionary biologists saw menopause and the long post-reproductive lifespan as unique human characteristics that may be usefully understood from an evolutionary perspective (Williams, 1957), although it is now known that menopause does exist in some long-lived species such as killer whales (Brent and others, 2015). Williams (1957) proposed that it may be beneficial for women to stop reproducing earlier, reducing their exposure to pregnancies of high risk to maternal and child survival. This would ensure mothers were more likely to survive to raise their existing family, in turn increasing survival. Data from natural fertility populations has not supported the proposed benefits to fitness of stopping reproduction early (Hill and Hurtado, 1991, 1996; Rogers, 1993). However, Williams’ article inspired a new field of investigation examining the impact grandparents have on survival and reproduction in families that continues to grow today (Coall and Hertwig, 2010) and has been discussed in several of the preceding chapters in this volume. Indeed, in our ageing populations, understanding the roles that longevity, ageing and the post-reproductive lifespan play in the human life history may be particularly valuable to active ageing and positive engagement with family, community, leisure activities and personal well-being.
At an ultimate level, ageing, senescence and menopause have long posed a challenge for the evolutionary perspective. Why, if the unit of selection is the individual, should ageing and menopause exist? Clearly they would appear to be bad for genetic fitness (Medawar, 1952). Why haven’t they been selected against? Moreover, as they occur after the reproductive lifespan is complete, there is little or no opportunity for natural selection to act upon them. These are valid points; however, they neglect the life-cycle focus of life history theory. As we have discussed, life history strategies are sensitive to resource availability and stress, creating trade-offs between the components of fitness (e.g. reproduction and maintenance). If post-reproductive adults, many of whom are likely to be grandparents, can invest in subsequent generations, thus influencing reproduction, growth, development and survival, the post-reproductive lifespan can be seen and moulded by natural selection.
Theoretical perspectives including the Grandmother Hypothesis, Cooperative Breeding Hypothesis, and Embodied Capital Hypothesis and their variants, propose that older post-reproductive individuals contribute resources to children and grandchildren that change their life cycles (Voland and others, 2005). That is to say, help from alloparents, such as grandparents, provides the resources that influence development in subsequent generations. Empirical studies have investigated the impact grandparent presence has on fitness measures, such as the fertility of their children and grandchild survival. The vast majority of this research has been conducted in natural fertility and historical human populations, and shows that the presence of grandmothers and the help they provide is often associated with increased grandchild survival (Sear and Mace, 2008).
Perhaps surprisingly, much less research has focused on the association between interactions with grandparents and grandchild outcomes in contemporary post-demographic transition populations. In these populations with low mortality and fertility, the outcomes are likely to reflect the emotional, social and material resources necessary to compete in those environments. The correlational research does support an association between grandparental involvement and improved psychological adjustment, mental and physical development and educational outcomes (Sear and Coall, 2011). Thus, consistent with theoretical perspectives mentioned above, downward resource transfers, which may be particularly evident through grandparenting, may confer a selective advantage to subsequent generations that drive human longevity and ultimately extend the human life cycle for both males and females.
Changes in the life cycle of human ancestors also suggest that post-reproductive lifespans and childhood are linked. In examinations of the fossil evidence, the extended post-reproductive lifespan in women and the long, slow growth period of childhood development appeared at roughly the same time in human history (Bogin, 1997). Both of these unique features of the human life history — most likely linked by a general increase in longevity — are likely to have evolved together as a self-reinforcing unit (Carey and Judge, 2001). As long ago as Homo erectus (1.9 million to 143,000 years ago), longevity estimates suggest they were living beyond 60 years of age, some fifteen years after menopause. This suggests that human longevity has a much more distant foundation, well beyond the increases in lifespan evident from recent advances in medicine and technology.
From an evolutionary perspective it has been hypothesized that helping behaviour, which brings additional resources to an individual within and beyond the family, ultimately developed from ancestral parenting and grandparenting. In turn, this helping behaviour may have contributed to extending the human lifespan. Recent evidence suggests that among elderly people, helpful grandparents, parents, and unrelated community members experience increased survivorship compared to individuals who do not help. Using data from the 516 participants in the Berlin Ageing Study, it was found that helpful grandparents who looked after their grandchildren survived five years longer than non-caregiving grandparents or non-grandparents (Hilbrand and others, 2017). Likely mechanisms that may link grandparental investments and reduced mortality include various measures of improved grandparental health and well-being. To date, longitudinal studies with more power to conduct within-individual analyses that more closely approximate causal relationships have found mixed results. Generally, however, health is unable to completely account for the relationship. With improved measures and statistical analyses, potential mediating pathways and causal relationships can be explored further in the association between grandparental involvement and longevity (Coall and others, 2018).
Conclusion
We hope we have demonstrated that thinking about human demographic behaviour in light of Tinbergen’s four explanations, and, in particular, considering ontogeny, is useful to demographers. Evolutionary theory adds value to demographic studies by underpinning ideas, hypotheses and empirical studies with a prediction-generating theory. Ontogeny is one of Tinbergen’s two proximate explanations for understanding how and why human demographic behaviour is adaptive. The genetic background and developmental environment are both crucial for shaping the individual’s reproductive trajectories and mortality expectations. It is crucial to consider different aspects of the childhood environment (e.g. psychosocial stress and nutrition) and the potential interactions between them. We hope this chapter has provided some answers, but more importantly, new questions and research opportunities for demographers. Demographers take a keen interest in both fertility and mortality, and when these topics are understood within an evolutionary life-history framework, a deeper understanding of the biological processes around death and reproduction can provide a fuller explanation of human demographic behaviour.
References7
Allsworth, Jenifer E., Jennifer Clarke, Jeffrey F. Peipert, Megan R. Hebert, Amy Cooper CRNP, and others. 2007. ‘The Influence of Stress on the Menstrual Cycle among Newly Incarcerated Women’, Women’s Health Issues : Official Publication of the Jacobs Institute of Women’s Health, 17.4: pp. 202–9, https://doi.org/10.1016/j.whi.2007.02.002
Anderson, Kermyt G. 2015. ‘Father Absence, Childhood Stress, and Reproductive Maturation in South Africa’, Human Nature, 26.4: pp. 401–25, https://doi.org/10.1007/s12110-015-9243-6
Bateson, Patrick, and Kevin N. Laland. 2013. ‘Tinbergen’s Four Questions: An Appreciation and an Update’, Trends in Ecology and Evolution, 28.12: pp. 712–18, https://doi.org/10.1016/j.tree.2013.09.013
Belsky, Jay, Laurence Steinberg, and Patricia Draper. 1991. ‘Childhood Experience, Interpersonal Development, and Reproductive Strategy: An Evolutionary Theory of Socialization’, Child Development, 62.4: pp. 647–70, https://doi.org/10.2307/1131166
Belsky, Jay, Laurence Steinberg, Renate M Houts, Sarah L Friedman, Ganie DeHart, and others. 2007. ‘Family Rearing Antecedents of Pubertal Timing’, Child Development, 78.4: pp. 1302–21, https://doi.org/10.1111/j.1467-8624.2007.01067.x
Berghänel, Andreas, Michael Heistermann, Oliver Schülke, and Julia Ostner. 2017. ‘Prenatal Stress Accelerates Offspring Growth to Compensate for Reduced Maternal Investment across Mammals’, Proceedings of the National Academy of Sciences, 114.50: pp. E10658–66, https://doi.org/10.1073/pnas.1707152114
Bjelland, E. K., S. Hofvind, L. Byberg, and A. Eskild. 2018. ‘The Relation of Age at Menarche with Age at Natural Menopause: A Population Study of 336 788 Women in Norway’, Human Reproduction, 33.6: pp. 1149–57, https://doi.org/10.1093/humrep/dey078
Bogaert, Anthony. 2005. ‘Age at Puberty and Father Absence in a National Probability Sample’, Journal of Adolescence, 28.4: pp. 541–46, https://doi.org/DOI: 10.1016/j.adolescence.2004.10.008
Bogin, Barry. 1997. ‘Evolutionary Hypotheses for Human Childhood’, Yearbook of Physical Anthropology, 104.S25: pp. 63–89. https://doi.org/10.1002/(SICI)1096-8644(1997)25+%3C63::AID-AJPA3%3E3.0.CO;2-8
—. 1998. ‘Evolutionary and Biological Aspects of Childhood’, in Biosocial Perspectives on Children, ed. by C Panter-Brick (Cambridge: Cambridge University Press).
—. 1999. Patterns of Human Growth, 2nd edn (Cambridge: Cambridge University Press).
Borgerhoff Mulder, Monique. 1998. ‘The Demographic Transition: Are We Any Closer to an Evolutionary Explanation?’, Trends in Ecology & Evolution, 13.7: pp. 266–70, https://doi.org/10.1016/S0169-5347(98)01357-3
Bornstein, M. H. 1989. ‘Sensitive Periods in Development: Structural Characteristices and Causal Interpretations’, Psychological Bulletin, 105.2: pp. 179–97, https://doi.org/10.1037/0033-2909.105.2.179
Brent, Lauren, Daniel Franks, Emma Foster, Kenneth Balcomb, Michael Cant, and others. 2015. ‘Ecological Knowledge, Leadership, and the Evolution of Menopause in Killer Whales’, Current Biology, 25.6: pp. 746–50, https://doi.org/10.1016/j.cub.2015.01.037
Carey, J. R., and D. S. Judge. 2001. ‘Life Span Extension in Humans Is Self-Reinforcing: A General Theory of Longevity’, Population and Development Review, 27: pp. 411–36, https://doi.org/10.1111/j.1728-4457.2001.00411.x
Cheshire, James, and Oliver O’Brien. 2015. ‘Lives on the Line’, http://tubecreature.com/#/livesontheline/current/same/U/*/TFTF/13/-0.1000/51.5200/
Chisholm, James. 1993. ‘Death, Hope, and Sex: Life-History Theory and the Development of Reproductive Strategies’, Current Anthropology, 34.1: pp. 1–24, https://doi.org/10.1086/204131
—. 1999. ‘The Young Male Syndrome: Coercion’, in Death, Hope and Sex: Steps to an Evolutionary Ecology of Mind and Morality (Cambridge: Cambridge University Press), p. 296.
Coall, D. A., A. C. Callan, J. Sartori, and J. S. Chisholm. 2019. ‘Prenatal Parenting’, in Handbook of Parenting, Biology and Ecology of Parenting, ed. by M. H. Bornstein (Routledge), https://doi.org/10.4324/9780429401459-5
Coall, D., A. K. Charles, and C. M. Salafia. 2009. ‘Gross Placental Structure in a Low-Risk Population of Singleton, Term, First-Born Infants’, Pediatric and Developmental Pathology, 12.3: pp. 200–10, https://doi.org/10.2350/08-02-0413.
Coall, David A., Sonja Hilbrand, Rebecca Sear, and Ralph Hertwig. 2018. ‘Interdisciplinary Perspectives on Grandparental Investment: A Journey towards Causality’, Contemporary Social Science, 13.2: pp. 159–74, https://doi.org/10.1080/21582041.2018.1433317
Coall, David A., and Ralph Hertwig. 2010. ‘Grandparental Investment: Past, Present, and Future’, Behavioral and Brain Sciences, 33.1: pp. 1–19; discussion pp. 19–40, https://doi.org/10.1017/s0140525x09991105
Coall, David A., M. Tickner, L. S. McAllister, and P. Sheppard. 2016a. ‘Developmental Influences on Fertility Decisions by Women: An Evolutionary Perspective’, Philosophical Transactions of the Royal Society B: Biological Sciences, 371.1692, https://doi.org/10.1098/rstb.2015.0146
Coall, David A., Michelle Tickner, Lisa McAllister, and Paula Sheppard. 2016b. ‘Developmental Influences on Human Fertility Decisions: An Evolutionary Perspective’, Philosophical Transactions of the Royal Society of London. Series B, Biological Sciences 371.1692: pp. 13–18. https://doi.org/10.1098/rstb.2015.0146
Cross, Catharine P. 2010. ‘Sex Differences in Same-Sex Direct Aggression and Sociosexuality: The Role of Risky Impulsivity’, Evolutionary Psychology, 8.4: pp. 779–92. https://doi.org/10.1177/147470491000800418
Ellis, B. 2004. ‘Timing of Pubertal Maturation in Girls: An Integrated Life History Approach’, Psychological Bulletin, 130.6: pp. 920–58, https://doi.org/10.1037/0033-2909.130.6.920
Ellison, Peter T., Meredith W. Reiches, Heather Shattuck-Faegre, Alicia Breakey, Martina Konecna, and others. 2012. ‘Puberty as a Life History Transition’, Annals of Human Biology, 39.5: pp. 352–60, https://doi.org/10.3109/03014460.2012.693199
Finch, Caleb E., and Michael R. Rose. 1995. ‘Hormones and the Physiological Architecture of Life History Evolution’, The Quarterly Review of Biology, 70.1: pp. 1–52, https://doi.org/10.1086/418864
Foley, Robert. 1995. ‘The Adaptive Legacy of Human Evolution: A Search for the Environment of Evolutionary Adaptedness’, Evolutionary Anthropology: Issues, News, and Reviews, 4.6: pp. 194–203, https://doi.org/10.1002/evan.1360040603
Fusco, Giuseppe, and Alessandro Minelli. 2010. ‘Phenotypic Plasticity in Development and Evolution: Facts and Concepts’, Philosophical Transactions of the Royal Society B: Biological Sciences, 365.1540: pp. 547–56, https://doi.org/10.1098/rstb.2009.0267
Gettler, Lee T., Thomas McDade, Alan B. Feranil, and Christopher W. Kuzawa. 2011. ‘Longitudinal Evidence That Fatherhood Decreases Testosterone in Human Males’, Proceedings of the National Academy of Sciences, 108.39: pp. 16194–99, https://doi.org/10.1073/pnas.1105403108
Gluckman, Peter D., and Mark A. Hanson. 2004. ‘Maternal Constraint of Fetal Growth and Its Consequences’, Seminars in Fetal and Neonatal Medicine, 9.5: pp. 419–25, https://doi.org/10.1016/j.siny.2004.03.001
Gluckman, Peter, Mark Hanson, and Hamish G. Spencer. 2005. ‘Predictive Adaptive Responses and Human Evolution’, Trends in Ecology & Evolution, 20.10: pp. 527–33, https://doi.org/10.1016/j.tree.2005.08.001
Godfrey, K., B. Breier, and C. Cooper. 1999. ‘Constraint of the Materno-Placental Supply of Nutrients: Causes and Consequences’, in Fetal Programming: Influences on Development and Disease in Later Life., ed. by P. O’Brien and D. Barker (London: RCOG Press), pp. 283–98.
Gold, Ellen B., Joyce Bromberger, Sybil Crawford, Steve Samuels, Gail A. Greendale, and others. 2001. ‘Factors Associated with Age at Natural Menopause in a Multiethnic Sample of Midlife Women’, American Journal of Epidemiology, 153.9: pp. 865–74, https://doi.org/10.1093/aje/153.9.865
Harville, E. W., and R. Boynton-Jarrett. 2013. ‘Childhood Social Hardships and Fertility: A Prospective Cohort Study’, Annals of Epidemiology, 23.12: pp. 784–90.el, https://doi.org/10.1016/j.annepidem.2013.10.001
Hawkes, Kristen. 2003. ‘Grandmothers and the Evolution of Human Longevity’, American Journal of Human Biology, 15.3: pp. 380–400, https://doi.org/10.1002/ajhb.10156
Henrich, Joseph, Steven J. Heine, and Ara Norenzayan. 2010. ‘The Weirdest People in the World?’, The Behavioral and Brain Sciences, 33.2–3: pp. 61–83; discussion pp. 83–135, https://doi.org/10.1017/S0140525X0999152X
Hilbrand, Sonja, David A. Coall, Denis Gerstorf, and Ralph Hertwig. 2017. ‘Caregiving within and beyond the Family Is Associated with Lower Mortality for the Caregiver: A Prospective Study’, Evolution and Human Behavior, 38.3: pp. 397–403, https://doi.org/10.1016/j.evolhumbehav.2016.11.010
Hill, Kenneth. 1996. ‘The Demography of Menopause’, Maturitas, 23.2: pp. 113–27, https://doi.org/10.1016/0378-5122(95)00968-X
Hill, Kim, and A. Magdalena Hurtado. 1991. ‘The Evolution of Premature Reproductive Senescence and Menopause in Human Females’, Human Nature, 2.4: pp. 313–50, https://doi.org/10.1007/BF02692196
Hill, Kim, and A. Magdalena Hurtado. 1996. Aché Life History: The Ecology and Demography of a Foraging People (Hawthorne, New York: Aldine de Gruyter).
Jacobs, Marni B., Renee D. Boynton-Jarrett, and Emily W. Harville. 2015. ‘Adverse Childhood Event Experiences, Fertility Difficulties, and Menstrual Cycle Characteristics’, Journal of Psychosomatic Obstetrics and Gynaecology, 36.2: pp. 46–57, https://doi.org/10.3109/0167482X.2015.1026892
Jacobsen, Bjarne K., Ivar Heuch, and Gunnar Kvåle. 2003. ‘Age at Natural Menopause and All-Cause Mortality: A 37-Year Follow-up of 19,731 Norwegian Women’, American Journal of Epidemiology, 157.10: pp. 923–29, https://doi.org/10.1093/aje/kwg066
Jones, James Holland. 2005. ‘Fetal Programming: Adaptive Life-History Tactics or Making the Best of a Bad Start?’, American Journal of Human Biology, 17.1: pp. 22–33, https://doi.org/10.1002/ajhb.20099
Kuzawa, Christopher W. 2005. ‘Fetal Origins of Developmental Plasticity: Are Fetal Cues Reliable Predictors of Future Nutritional Environments?’, American Journal of Human Biology, 17.1: pp. 5–21, https://doi.org/10.1002/ajhb.20091
Kuzawa, Christopher W., and Jared M. Bragg. 2012. ‘Plasticity in Human Life History Strategy: Implications for Contemporary Human Variation and the Evolution of Genus Homo’, Current Anthropology, 53.S6: pp. S369–82, https://doi.org/10.1086/667410
Lahdenperä, Mirkka, Khyne U. Mar, and Virpi Lummaa. 2014. ‘Reproductive Cessation and Post-Reproductive Lifespan in Asian Elephants and Pre-Industrial Humans’, Frontiers in Zoology, 11.1: p. 54, https://doi.org/10.1186/s12983-014-0054-0
Low, B., A. Hazel, and N. Parker. 2009. ‘Influences on Women’s Reproductive Lives Unexpected Ecological Underpinnings’, Cross-Cultural Research, 42.3, https://doi.org/10.1177/1069397108317669
Maestripieri, Dario, and J. Mateo. 2009. Maternal Effects in Mammals (Chicago: University of Chicago Press).
Mattison, Siobhán M., and Mary K. Shenk. 2019. ‘Ecological Evolutionary Demography’. https://www.researchgate.net/publication/339314425_Ecological_Evolutionary_Demography
Mayr, E. 1961. ‘Cause and Effect in Biology’, Science, 134.3489: pp. 1501–6, https://doi.org/10.1126/science.134.3489.1501
Medawar, P. 1952. An Unsolved Problem of Biology (London: H.K. Lewis).
Nettle, Daniel. 2010. ‘Dying Young and Living Fast: Variation in Life History across English Neighborhoods’, Behavioural ecology, 21.2: pp. 387–95, https://doi.org/10.1093/beheco/arp202
Nettle, Daniel, David Coall, and Thomas E. Dickins. 2011. ‘Early-Life Conditions and Age at First Pregnancy in British Women’, Proceedings of the Royal Society B: Biological Sciences, 278.1712: pp. 1721–27, https://doi.org/10.1098/rspb.2010.1726
Nettle, Daniel, Willem E. Frankenhuis, and Ian Rickard. 2013. ‘The Evolution of Predictive Adaptive Responses in Human Life History’, Proceedings of the Royal Society B: Biological Sciences, 280.1766: pp. 1–9, https://doi.org/10.1098/rspb.2013.1343
Núñez-De La Mora, Alejandra, Robert T. Chatterton, Osul A. Choudhury, Dora A. Napolitano, and Gillian R. Bentley. 2007. ‘Childhood Conditions Influence Adult Progesterone Levels’, PLoS Medicine, 4.5: pp. 0813–21, https://doi.org/10.1371/journal.pmed.0040167
Pepper, G. V., M. Bateson, and D. Nettle. 2018. ‘Telomeres as Integrative Markers of Exposure to Stress and Adversity: A Systematic Review and Meta-Analysis’, BioRxiv: pp. 1–19, https://doi.org/10.1101/320150
Pigliucci, Massimo. 2005. ‘Evolution of Phenotypic Plasticity: Where Are We Going Now?’, Trends in Ecology & Evolution, 20.9: pp. 481–86, https://doi.org/10.1016/j.tree.2005.06.001
Potts, Richard. 1998. ‘Variability Selection in Hominid Evolution’, Evolutionary Anthropology: Issues, News, and Reviews, 7.3: pp. 81–96, https://doi.org/10.1002/(SICI)1520-6505(1998)7:3<81::AID-EVAN3>3.0.CO;2-A
Quinlan, Robert J. 2003. ‘Father Absence, Parental Care, and Female Reproductive Development’, Evolution and Human Behavior, 24.6: pp. 376–90, https://doi.org/Doi: 10.1016/s1090-5138(03)00039-4
Rickard, Ian J., Willem E. Frankenhuis, and Daniel Nettle. 2014. ‘Why Are Childhood Family Factors Associated with Timing of Maturation? A Role for Internal Prediction’, Perspectives on Psychological Science, 9.1: pp. 3–15, https://doi.org/10.1177/1745691613513467
Rogers, Alan R. 1993. ‘Why Menopause?’, Evolutionary Ecology, 7.4: pp. 406–20, https://doi.org/10.1007/BF01237872
Sapolsky, Robert M. 2017. Behave: The Biology of Humans at Our Best and Worst (New York: Penguin Random House USA).
Schacht, Ryan, and Monique Borgerhoff Mulder. 2015. ‘Sex Ratio Effects on Reproductive Strategies in Humans’, Royal Society Open Science, 2.1: p. 140402, https://doi.org/10.1098/rsos.140402
Sear, Rebecca, and David Coall. 2011. ‘How Much Does Family Matter? Cooperative Breeding and the Demographic Transition’, Population and Development Review, 37.s1: pp. 81–112, https://doi.org/10.1111/j.1728-4457.2011.00379.x
Sear, Rebecca, and Ruth Mace. 2008. ‘Who Keeps Children Alive? A Review of the Effects of Kin on Child Survival’, Evolution and Human Behavior, 29.1: pp. 1–18, https://doi.org/10.1016/j.evolhumbehav.2007.10.001
Sear, Rebecca, Paula Sheppard, and David A. Coall. 2019. ‘Cross-Cultural Evidence Does Not Support Universal Acceleration of Puberty in Father-Absent Households’, Philosophical Transactions of the Royal Society B: Biological Sciences, 374.1770: pp. 20180124. https://doi.org/10.1098/rstb.2018.0124
Sheppard, P., K. Snopkowski, and R. Sear. 2014. ‘Father Absence and Reproduction-Related Outcomes in Malaysia, a Transitional Fertility Population’, Human Nature, 25.2, https://doi.org/10.1007/s12110-014-9195-2
Sheppard, Paula, Justin R. Garcia, and Rebecca Sear. 2015. ‘Childhood Family Disruption and Adult Height: Is There a Mediating Role of Puberty?’, Evolution, Medicine, and Public Health, 2015.1: pp. 332–42, https://doi.org/10.1093/emph/eov028
Sheppard, Paula, Mark Pearce, and Rebecca Sear. 2016. ‘How Does Childhood Socioeconomic Hardship Affect Reproductive Strategy? Pathways of Development’, American Journal of Human Biology, 28.3: pp. 356–63, https://doi.org/10.1002/ajhb.22793
Sheppard, Paula, and Rebecca Sear. 2012. ‘Father Absence Predicts Age at Sexual Maturity and Reproductive Timing in British Men’, Biology Letters, 8.2: pp. 237–40, https://doi.org/10.1098/rsbl.2011.0747
Stearns, Stephen. 1992. The Evolution of Life Histories (Oxford: Oxford University Press).
The World Bank. 2018. ‘World Development Indicators’, https://databank.worldbank.org/source/world-development-indicators
Tinbergen, N. 1963. ‘On Aims and Methods of Ethology’, Zeitschrift Für Tierpsychologie, 20.4: pp. 410–33, https://doi.org/10.1111/j.1439-0310.1963.tb01161.x
Vitzthum, Virginia J. 2001. ‘Why Not so Great Is Still Good Enough’, in Reproductive Ecology and Human Evolution, ed. by Peter T Ellison (New York: Aldine de Gruyter), pp. 179–202.
Voland, E., A. Chasiotis, and W. Schiefenhovel. 2005. ‘Grandmotherhood: A Short Overview of Three Fields of Research on the Evolutionary Significance of Postgenerative Female Life’, in Grandmotherhood. The Evolutionary Significance of the Second Half of Female Life, ed. by E. Voland, A. Chasiotis, and W Schiefenhovel (New Brunswick: Rutgers University Press), pp. 1–17.
Waynforth, David. 2012. ‘Life-History Theory, Chronic Childhood Illness and the Timing of First Reproduction in a British Birth Cohort’, Proceedings of the Royal Society B: Biological Sciences, 279.1740: pp. 2998–3002, https://doi.org/10.1098/rspb.2012.0220
Waynforth, David, A. Magdalena Hurtado, and Kim Hill. 1998. ‘Environmentally Contingent Reproductive Strategies in Mayan and Ache Males’, Evolution and Human Behavior, 19.6: pp. 369–85, https://doi.org/10.1016/S1090-5138(98)00031-2
Wells, Johnathan. 2019. ‘Developmental Plasticity as Adaptation: Adjusting to the External Environment under the Imprint of Maternal Capital’, Philosophical Transactions of the Royal Society B: Biological Sciences, 374.1770: p. 20180122, https://doi.org/10.1098/rstb.2018.0122
Wells, Jonathan. 2010. ‘Maternal Capital and the Metabolic Ghetto: An Evolutionary Perspective on the Transgenerational Basis of Health Inequalities’, American Journal of Human Biology, 22.1: pp. 1–17, https://doi.org/10.1002/ajhb.20994
Williams, G. C. 1957. ‘Pleiotropy, Natural Selection, and the Evolution of Senescence’, Evolution, 11.4: pp. 398–411, https://doi.org/10.1111/j.1558-5646.1957.tb02911.x
Winking, Jeffrey, Michael Gurven, and Hillard Kaplan. 2011. ‘Father Death and Adult Success among the Tsimane: Implications for Marriage and Divorce’, Evolution and Human Behavior, 32.2: pp. 79–89, https://doi.org/10.1016/j.evolhumbehav.2010.08.002
1 Bears do not actually hibernate; they go into “torpor” which is a very deep sleep state, but not strictly hibernation. The four explanations as to why they do so still apply.
2 We use these two terms interchangeably in this chapter.
3 In biology, “choices”, “decisions”, and “strategies” are generally not conscious (although in some cases they can be); they are responses to the interactions between environmental and genetic influences.
4 Western, Educated, Industrial, Rich, and Democratic.
5 Age at puberty is often taken to indicate if an individual is on a fast or slow life history track. This assumes that all life history traits (or at least puberty, reproduction and death) are correlated, which has not yet been empirically established.
6 Reproduced from Sheppard et al. 2015. ‘Childhood family disruption and adult height: is there a mediating role of puberty?’, Evolution, Medicine, and Public Health, 1: pp. 332–42. By permission of Oxford University Press on behalf of the International Society for Evolution, Medicine & Public Health. For commercial reuse queries, please contact journals.permissions@oup.com.
7 Note this chapter has been posted on the Open Science Framework website since 10/04/2020, after it was accepted for publication, so the references will reflect when the chapter was written and not the OBP publication date.