13. Fly Colony Establishment, Quality Control and Improvement
© 2022 Chapter Authors, CC BY-NC 4.0 https://doi.org/10.11647/OBP.0300.13
This chapter provides guidance on the collection and selection of species suitable for maggot therapy. All life stages are suitable for collection, except pupae that are generally hidden from view. Correct identification of the species that are collected and the correct selection of breeding stock is critical. Domestication of the newly established fly colony proceeds via adaptation to the insectary environment and the producers’ operating procedures. Monitoring of fly colony life history and morphological traits enables producers to manage the adaptation of flies to the insectary environment and to improve performance through selective breeding, genetic replenishment, and genetic engineering.
Introduction
The first step in the establishment of a laboratory colony is the collection of flies and their subsequent maintenance in the laboratory or insectary [e.g. 1, 2, 3]. While in some instances blowfly strains have been reared continuously for decades [4], there is concern that relatively small population sizes, limited genetic diversity, rapid selection and adaptation to the laboratory [5] may require genetic replenishment and rejuvenation of lab colonies with newly caught flies on a regular basis to ensure productivity and health of the colonies [6].
Moreover, maggot therapy treatment conditions vary between geographic areas and socio-economic conditions. For example, maggot therapy in Sub-Saharan Africa might benefit from the domestication of African fly species or strains such as Lucilia sericata or perhaps even the tropical African latrine fly Chrysomya putoria, that are already adapted to African environmental conditions. It is known that fly development is temperature-dependent and speeds up as temperature increases, up to a threshold temperature beyond which development is negatively impacted and may even lead to death [7]. While the laboratory and insectary conditions can be regulated, the wound temperature during treatment may be difficult to control. Average chronic wound temperature has been reported to be between 32 and 33ºC under mild climatic conditions [8–10]. Ambient atmospheric conditions in Sub-Saharan Africa may lead to a significant increase in the temperature medicinal maggots experience on the wound. Therefore, rather than importing flies from Europe, it will be beneficial to develop locally collected fly strains that are better adapted to higher developmental temperatures. However, further research to determine the influence of temperature on maggot therapeutic performance in tropical regions is required.
Finally, local quarantine laws and regulations may not permit the introduction of foreign insect material, which means that local flies will need to be collected. If the therapeutic efficacy of the species has not already been shown, its suitability for maggot therapy will need to be established. However, at times of great need when disaster or war is raging, or where there is no access to medical treatment, these restrictions may not apply, particularly regarding the use of local fly species [11, 12].
Chapter 11 is chiefly concerned with the bioprospecting and testing of new fly species for maggot therapy [13], while this chapter provides guidance on the collection and selection of species deemed suitable for maggot therapy. Not all of the species suggested here have been subject to robust testing as proposed in Chapter 11 but have a recorded history of successful medicinal use and at least one of the discussed species can be found in every major zoogeographic region. Because domestication is critical to the reliable supply of high-quality medicinal maggots, some attention must be given to the monitoring of fly colony life history and morphological traits. This will enable producers to manage the adaptation of flies to the insectary environment and to improve performance through selective breeding, genetic replenishment, and potentially genetic engineering.
Of course, it is not strictly necessary for maggot therapists to use maggots that were reared in the laboratory. Under extremely austere conditions and with no access to medical aid and purpose-produced medicinal maggots, it is possible to collect eggs from the wild or permit free-living females to deposit eggs on a wound [11, 12]. Such controlled myiasis is not without its risks but anecdotal and published case histories of maggot colonisation (myiasis) of necrotic wounds from surgery, violence, neglect, or misfortune demonstrate its potential benefit [14–16].
Medicinal Fly Species
There are many fly species worldwide that could potentially be employed to treat wounds. These belong mostly to the family Calliphoridae of which over 1,500 species have been described to date [17]. There are some prerequisites for species to be suitable for maggot therapy:
- In the first instance, any species used for maggot therapy must not consume or damage healthy tissue, but only debride dead or devitalised tissue.
- Ideally, the species used should not only debride dead tissue but also control infection and stimulate wound healing.
- The species should be easy to maintain in captivity and colonies should retain their vigour over many generations.
- The species should lay eggs and not give birth to live maggots. This is necessary to efficiently harvest and disinfect eggs and rear medicinal maggots prior to treatment.
Although a good number of fly species have been used to treat wounds (Table 13.1) the fly species most widely cited and regularly used for maggot therapy are the greenbottle blowfly L. sericata and to a lesser extent the sheep blowfly Lucilia cuprina [18–20]. However, other species are also under active experimental and clinical investigation for use in maggot therapy, including: i) the secondary screwworm Cochliomyia macellaria which is found from southern Canada to the South American tropics [21, 22], ii) the tropical African latrine fly Ch. putoria that is now also found across the Americas [23], and iii) the South American Sarconesiopsis magellanica [24]. There are likely to be many more fly species globally that could be employed to treat wounds and all except the harshest of environments should be home to candidate fly species for maggot therapy.
Table 13.1 Species that have been used in maggot therapy to date and associated references.
Reference |
|
Calliphora vicina |
[25] |
Lucilia caesar |
[26] |
Lucilia illustris |
[27] |
L. sericata |
[24, 28–30] |
L. cuprina |
[20, 31–33] |
Phormia regina |
[26, 29, 34] |
Protophormia terraenovae |
[35] |
Chrysomya megacephala |
[36] |
Ch. putoria |
[23] |
Co. macellaria |
[21, 22, 37] |
S. magellanica |
[24] |
Wohlfahrtia nuba |
[38] |
Musca domestica |
[39] |
Collecting Wild Flies
Several options are available to the collector of live flies for the establishment of laboratory colonies. Female flies can be encouraged to lay eggs on decomposing meat or other suitable bait [40], maggots may be hand-collected from wild carcasses and cadavers [7, 41, 42], and adult flies can be trapped [6, 43–45]. Common choices of bait include minced ovine (sheep), porcine (pork), or bovine (cattle) meat, but a wide range of other mammal and bird meats will attract medicinal fly species. It is best to use meat which has not been treated with preservatives. If fresh store-bought meat is used, give the meat up to 3 days to become putrid (depending on the ambient temperature), at which stage it will begin to attract significant numbers of blowflies. If using meat other than mince, to aid the process of decomposition and the release of volatile organic compounds, it can be beneficial to cut the meat into smaller pieces. The attractiveness of the decomposing meat can be further enhanced when it is mixed with sodium sulphide (Na2S) [46]. Furthermore, the attractive potential of meat bait is also enhanced once blowfly adults and larvae begin to arrive, as they release attractive semiochemicals when feeding [47]. Occasionally stirring the bait can further aid in the release of volatile organic compounds.
Importantly, the occurrence and distribution of necrophagous calliphorids varies greatly depending on the season and habitat [48, 49]. While some species are cold-tolerant and will remain active in winter in low abundances (e.g. C. vicina) [48], the majority of necrophagous calliphorids are only active in the warmer months. As such, fly collection should be undertaken in the warmer months in most geographic regions.
Trapping of Adult Flies
The characteristic behaviour of flies can be used to efficiently trap adults without killing them [50]. Flies in search of food and cadavers on which to deposit eggs have no difficulty searching out small dark holes and crevices as would be found on a cadaver. However, when taking flight, blowflies invariably head straight up and towards light. Cone or funnel traps make use of this behaviour. There are various versions of this trap type, but all work the same. A bait is provided to attract the flies. Suspended above the bait station is a cone/funnel (wide at the bottom and narrowing to a small opening at the top) which ends in a holding compartment made of fabric, a plastic bag or such containment. Flies that visit the bait station fly off vertically into the cone and wander upward through the small opening into the holding compartment. The flies have difficulty finding the small opening again and remain trapped [50]. Although cone/funnel traps are easily constructed, there are now various types of these traps available online via entomological equipment suppliers and popular retail platforms such as eBay and Alibaba (e.g. Figure 13.1). Traps that have been specifically designed for entomological investigations will allow easy access and collection of trapped flies while the inexpensive traps sold for pest control may be permanently closed and make access to the fly compartment difficult. This requires modification of the fly trap prior to use, or particular retrieval strategies as described in Figure 13.2.
A variant of this trap mechanism utilises the same inability of flies to escape through an inverted, funnel-shaped opening. Inexpensive plastic storage containers may be baited with meat or other attractants and furnished with inward-pointing cones [44]. Commercial traps exploit this approach to capture sheep blowflies that can cause significant stock loss and animal suffering [51]. Figure 13.3 explains how an inexpensive trap based on the same principle can be constructed from a simple plastic bottle.
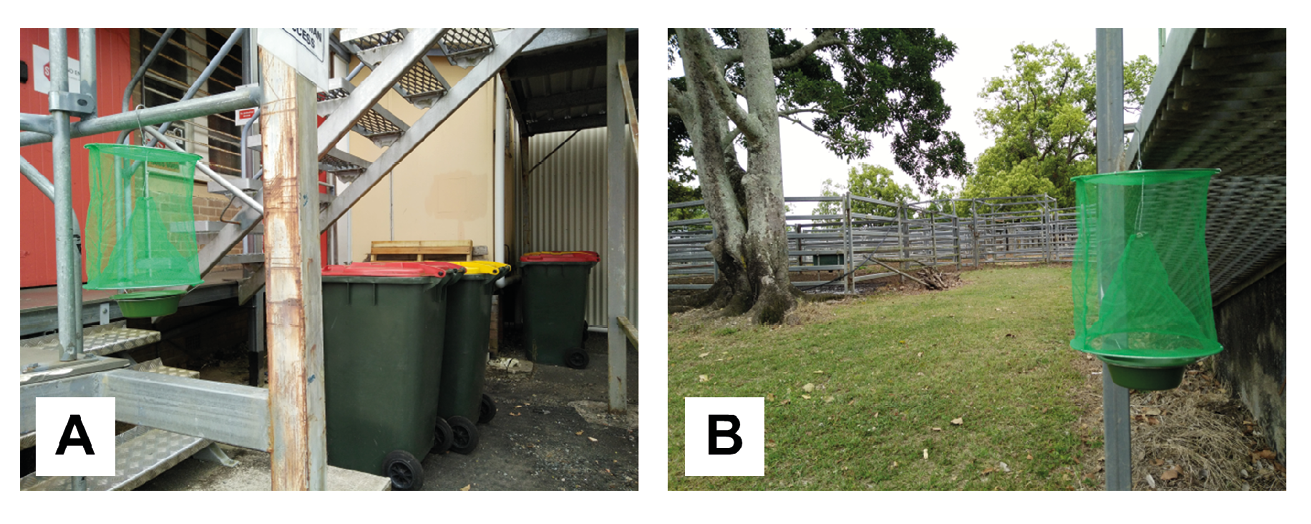
Figure 13.1 Inexpensive fly trap available from online retailers. The trap is best set up where flies naturally congregate. In an urban setting, this may be domestic or commercial garbage bins or near food businesses (A). In the rural environment, traps may be set up near livestock (B), but flies will detect attractive bait from afar and find the trap even if it is set up away from livestock or food waste. Photos by F. Stadler, CC BY-NC.
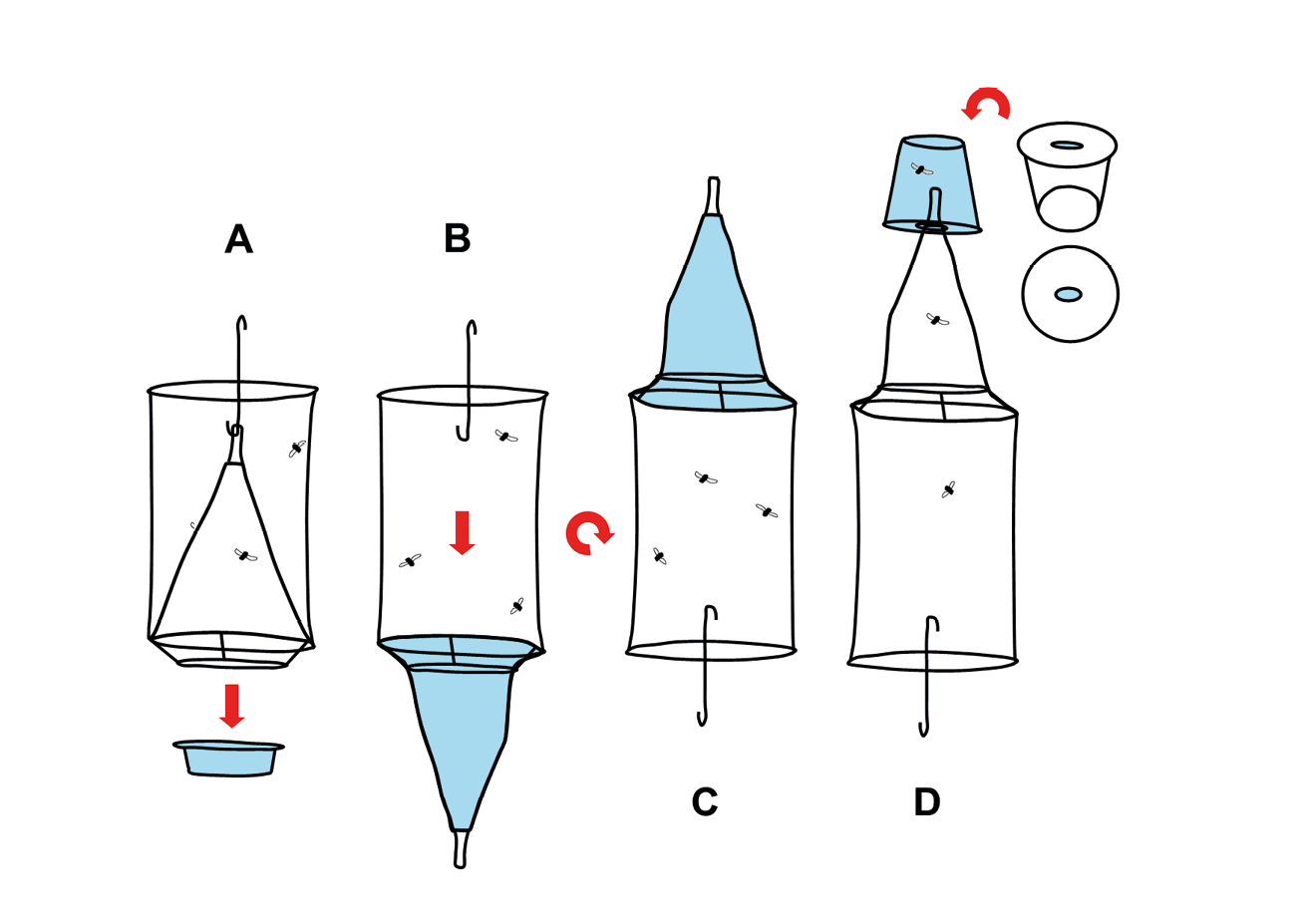
Figure 13.2 Retrieval of flies. Flies may be removed from the trap in Figure 13.1 by exploiting their natural inclination to move upward. Remove the bait tray from the trap (A). Unhook the net funnel and pull it out through the wire basket that held the bait tray (B). Turn the trap upside down (C). Use a transparent plastic container and cut an oval hole into the lid or bottom of it. It has to be wide enough to receive the narrow end of the trap’s net funnel but thinner than the diameter of the funnel opening (D). When inserted, the metal-enforced funnel opening will sit on the inside edge of the hole without slipping out. Let the trap hang from the plastic container and make sure the funnel is untwisted to avoid obstruction. Flies will climb upward and through the funnel into the collection container (D). Illustration by F. Stadler, CC BY-NC.
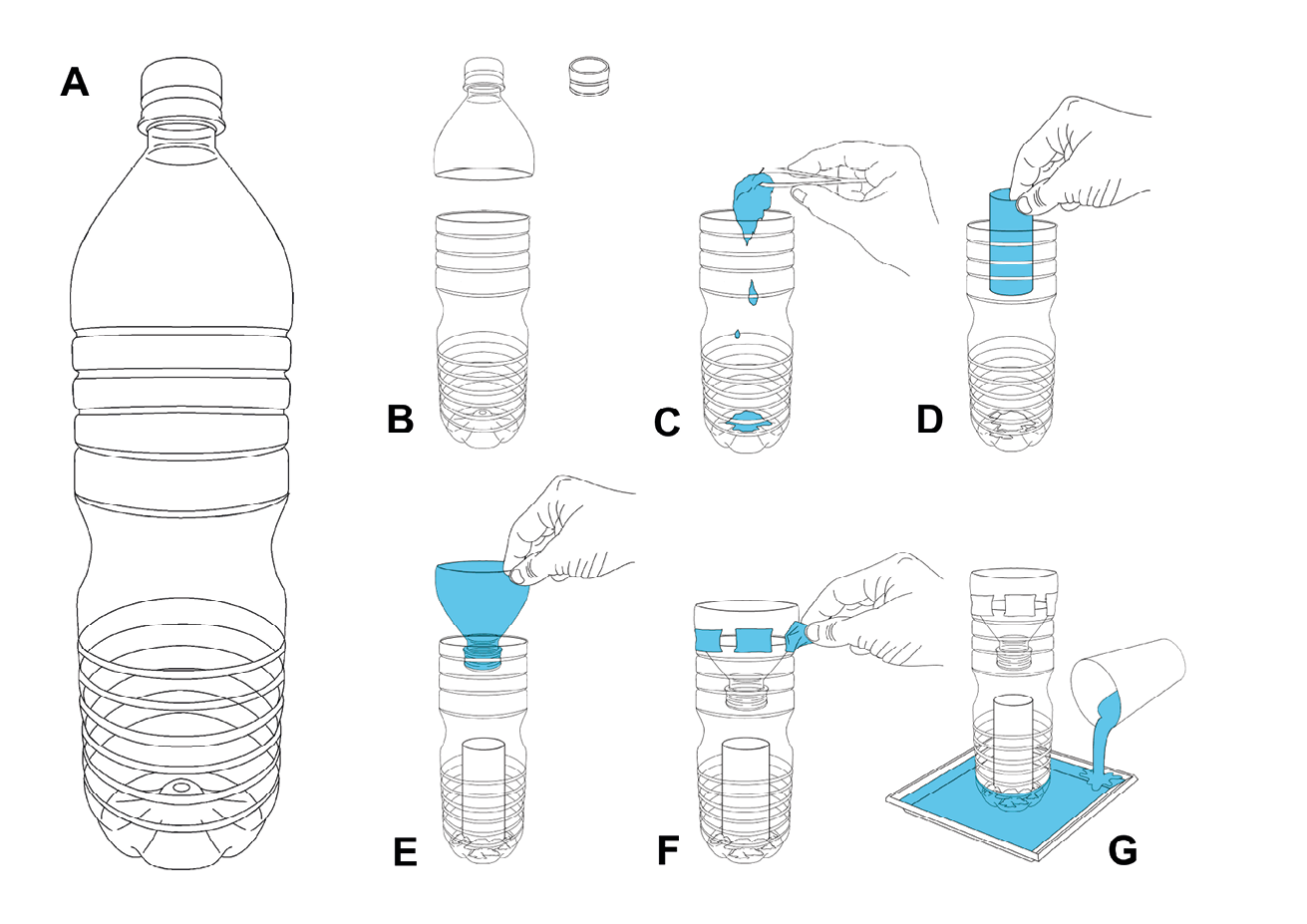
Figure 13.3 Instruction for a DIY plastic container cone trap. A standard soft drink plastic bottle (A). The tapered neck is cut off and the lid is removed (B). The bottle is baited with putrid meat to attract flies (C). A piece of cardboard such as a toilet paper roll is placed on top of the bait to provide a perch for flies as they may not be able to easily grip the plastic bottle walls after contact with the moist bait (D). The tapered neck is inverted and fitted back onto the bottle (without the lid) (E) and secured with a few strips of sticky tape (F). The trap is then placed in a dish of water to keep ants and other crawling insects out (G). The trap should be set up in a spot that is accessible to flies but protected from rain and direct sun. Illustration by P. Busana, MedMagLabs and Creating Hope in Conflict: A Humanitarian Grand Challenge, CC BY-ND.
Collecting Eggs
Female flies are attracted to cadavers to feed on protein-rich food and lay their eggs. It is therefore possible to collect eggs by placing meat bait in select locations during the day. Flies are not nocturnal and will not seek out the bait during the night. However, flies prefer to lay their eggs in dark recesses and orifices of cadavers such as the mouth or nostrils. Therefore, offering the bait in a dark container mimicking a cadaver and covering it while still allowing flies easy access via entry holes will make the bait more attractive. The bait must also be protected from larger animals and ants that will raid this free food. Birds and rats may be excluded with a wire mesh cage of sorts. Ants and other crawling invertebrates can be prevented from accessing the bait by placing the meat container into a larger tray/container partially filled with water. This creates a moat surrounding the meat that cannot be crossed by ants. Rain protection and a dark cover to mimic a cadaver can be achieved by placing a dark sheet of plastic material or similar cover onto the exclusion cage. The bait station may be set up in the morning and by late afternoon the eggs must be collected from the bait or else they are prone to dry out if it is hot, or hatch overnight. Unfortunately, it is not easy to discern what fly species has laid the eggs. One way to narrow down the species is by collecting egg masses from the bait used in a trap as described earlier and in Figure 13.1. It will be highly likely that the eggs will have been deposited by flies that were subsequently trapped. Irrespective of the method, it is best to place individual egg masses laid by a single female into separate rearing containers with larval diet. Once they have been raised to adult stage, they can be properly identified and placed into communal cages along with flies of the same species.
Hand Collecting of Adults, Eggs and Maggots
When hand collecting fly specimens, a bait such as an animal carcass, a quantity of putrid meat, or even faeces can be placed in a convenient spot to attract flies over a period of a few days. The collector returns regularly to the bait to collect adult flies with an entomological sweep net. The cadavers may also be examined periodically for egg masses and maggots. Various utensils such as spoons, forceps and applicator sticks may be used to pick eggs and maggots off the bait. Pathology specimen containers, various test tubes, or even empty food jars may be used to store and transport collected eggs and maggots to the insectary for identification and culture. Containers will not need to be ventilated if this transfer happens quickly. It is, however, best to use containers that are fitted with lids that permit air exchange. For this purpose, larger holes can be drilled into the lid and a sheet of fine-weave fabric can be sandwiched between lid and container-mouth prior to closing. This is especially necessary if very active, minute maggots are to be collected.
Taxonomic Identification and Confirmation of Species
Great care must be taken in accurate identification and selection of the desired fly species to ensure that the flies added to the existing laboratory stock are of the same species and that no harmful species is inadvertently selected. Morphological characteristics are used for the identification of adult flies [52–58], larvae [59–62], and pupae [63]. Even non-specialists can use reliable morphological character differences between L. sericata and L. cuprina [64]. In recent times a variety of molecular genetic techniques have been employed for taxonomic studies and identification of species, including L. sericata, and have been found to be as reliable as examination of morphological characters [55, 65–68]. Because many maggot therapy entrepreneurs will not have access to molecular laboratory facilities and may find it difficult to access the taxonomic literature, it follows an identification guide that highlights the most relevant medicinal fly species for each zoogeographic region (Figure 13.4).
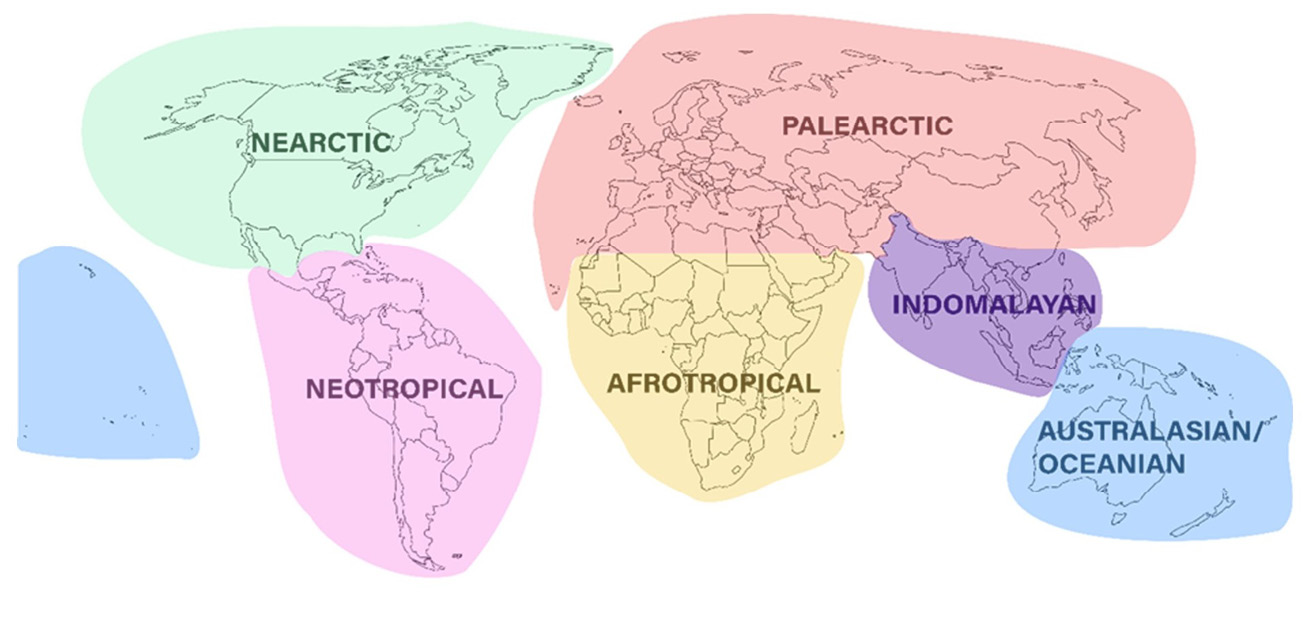
Figure 13.4 The six major zoogeographic regions of the world.
Although a range of species have been used in maggot debridement therapy (Table 13.1), we have chosen to provide identification guides to the following five species because they are well described biologically and broadly distributed throughout the world.
- C. vicina
- L. sericata
- L. cuprina
- Co. macellaria
- Ch. megacephala
At least one of these species can be found in every major zoogeographic region (Table 13.2). Importantly, the diagnoses provided here are only intended to be used as a general guide for identifying flies that are associated with and breed in carrion. There will be uncommon and non-carrion-breeding blowfly species that cannot be discriminated by the simplified diagnoses we provide here. It is therefore crucial that specimen identity be confirmed by reference to one of the many published resources such as those cited here (Table 13.2), or by sending your specimen to an expert entomologist at your closest research institution. For a list of recommended institutions refer to Table 13.2. For later reference throughout this section, the term ‘bristles’ refers to larger hairs (setae) located in a sunken pit in the exoskeleton. The term ‘hairs’ refers to smaller hairs (setulae) not located in such a pit.
Table 13.2 The six major zoogeographic regions of the world, and which of the five fly species (C. vicina, L. sericata, L. cuprina, Co. macellaria, Ch. megacephala) are found there. Also provided are suggested facilities that can provide specimen identification services. The last column lists the references to the relevant taxonomic keys for species identification.
Species |
ID Facility |
Key |
|
Afrotropical |
L. sericata L. cuprina Ch. megacephala |
KwaZulu-Natal Museum, South Africa |
[69] |
Indomalayan |
Ch. megacephala L. cuprina |
Department of Parasitology, Faculty of Medicine, Chiang Mai University, Thailand |
[70–72] |
Palearctic |
C. vicina L. sericata |
Natural History Museum, UK |
[58, 73] |
Nearctic |
L. sericata L. cuprina Co. macellaria |
Smithsonian National Museum of Natural History, USA |
[74, 75] |
Australasian-Oceanian |
L. sericata L. cuprina Ch. megacephala C. vicina |
Australian National Insect Collection, Australia |
[76, 77] |
Neotropical |
Co. macellaria L. cuprina Ch. megacephala |
Laboratory of Integrative Entomology, Department of Animal Biology, University of Campinas, Brazil |
[74, 78] |
Calliphorid Fly Morphology Relevant to the Identification of Species
In order to facilitate the identification of flies using the information and figures provided in this section, it will be helpful to first locate the relevant morphological characters on a fly (Table 13.3 and Figure 13.5). The blue highlighting is omitted in subsequent species plates in order not to obstruct the features.
Table 13.3 Key to morphological characters used for species identification.
Head |
||
Fo.P |
fronto-orbital plate |
Figure 13.5a, b |
G |
genae |
Figure 13.5a, e |
Pa.V |
paravertical |
Figure 13.5c |
Pf |
parafacials |
Figure 13.5e |
Vi.S |
vibrissal setulae |
Figure 13.5e |
Pl |
palpi |
Figure 13.5e |
Thorax |
||
H.C. |
humeral calli |
Figure 13.5a, b |
Lo.C |
lower calypter |
Figure 13.5a |
Wing |
||
St.V |
stem vein |
Figure 13.5d |
B |
basicosta |
Figure 13.5a, b |
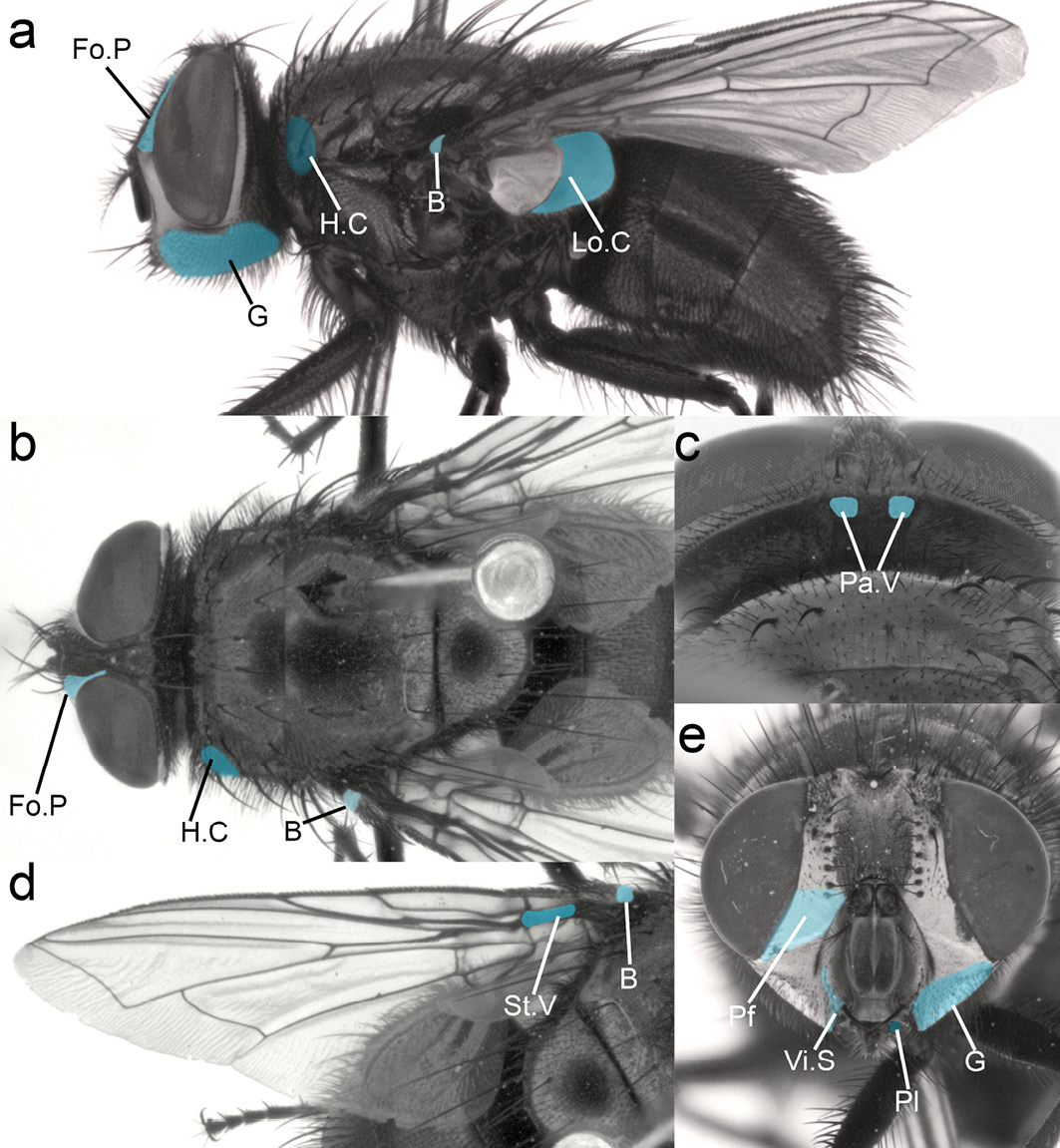
Figure 13.5 Morphological characters required for the identification of the five medicinal fly species. Head: Fo.P fronto-orbital plate, G genae, Pa.V paravertical, Pf parafacials, Vi.S vibrissal setulae, Pl palpi. Thorax: H.C. humeral calli, Lo.C lower calypter. Wing: St.V stem vein, B basicosta. CC BY-NC.
Genus Calliphora
Genus Diagnosis. Flies of the genus Calliphora (Figure 13.6) are generally black or brown in colour with a blue-metallic abdomen (rarely green), and a powdery coating on their thorax and abdomen. The genus Calliphora can be distinguished from Lucilia, Chysomya and Cochliomya by the combination of the following characters: lower parafacial area without strong bristles (Figure 13.6d), stem vein without bristles on dorsal surface (Figure 13.6e), dorsal surface of lower calypter hairy (Figure 13.6b). Note that some of these characters may be present in the other mentioned genera, but the combination of all three is unique to the genus Calliphora.
Calliphora vicina. C. vicina can be distinguished from other carrion-breeding members of the genus Calliphora through the combination of following characteristics: abdomen metallic blue with a silver powdery coating that changes with viewing angle (Figure 13.6c), eyes without any hairs (Figure 13.6a, c, d), parafacials and genae orange, with black hairs on genae (Figure 13.6a, d), basicosta yellow/orange-brown (can appear darker but not black) (Figure 13.6c, e).
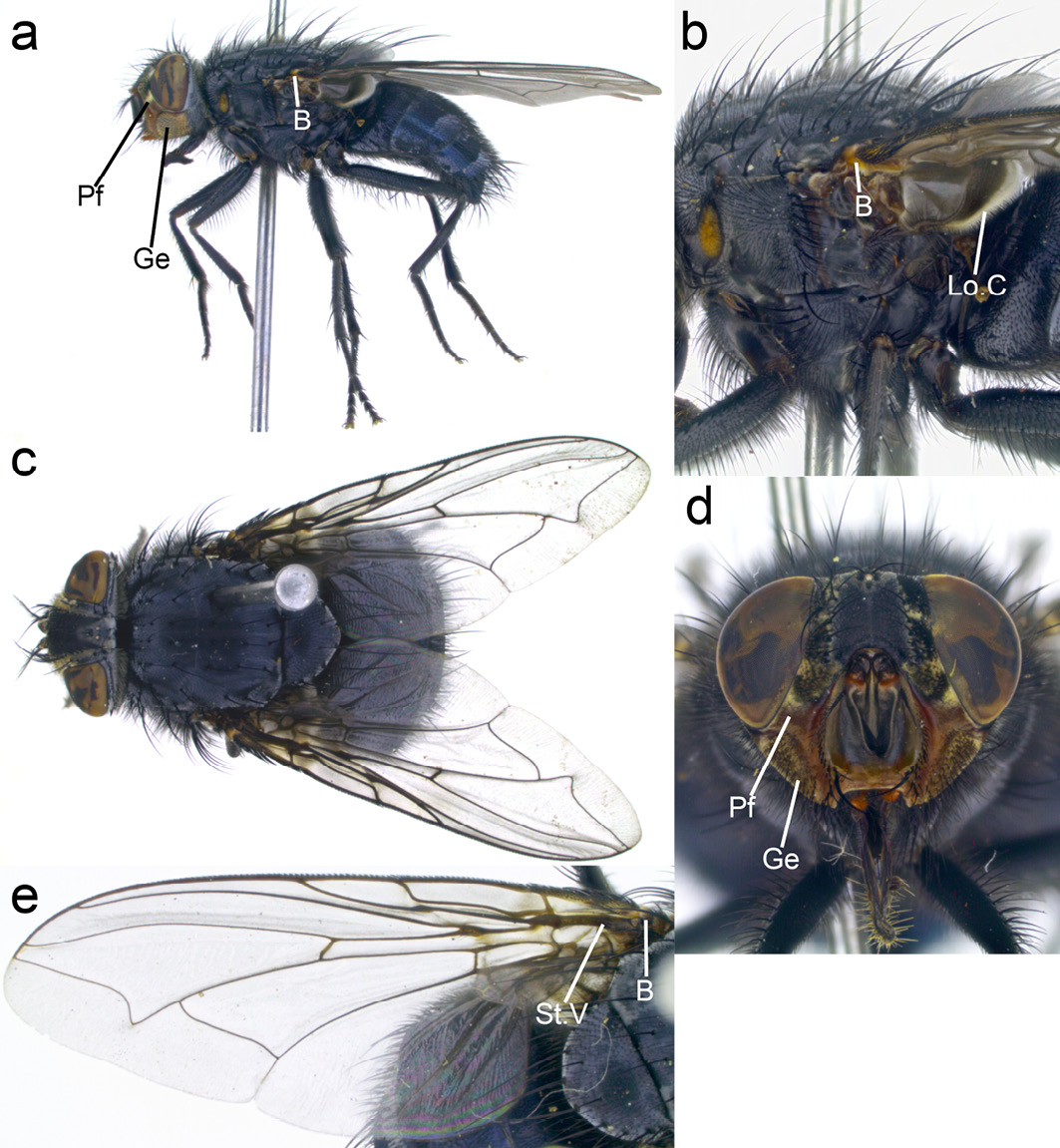
Figure 13.6 Calliphora vicina. Pf: parafacials, Ge: genae, B: basicosta, Lo.C: lower calypter. CC BY-NC.
Genus Lucilia
Genus Diagnosis. Flies of the genus Lucilia can be distinguished from Calliphora by the bright metallic green, blue or copper thorax and abdomen without any significant powdery coating (Figure 13.7c, 13.8c). In addition, this genus can be distinguished from Chrysomya and Cochliomya by the combination of wing stem vein without hairs on dorsal surface (Figure 13.7e, 13.8e) and lower calypter without hairs on dorsal surface (Figure 13.7b, 13.8b).
Lucilia cuprina. This fly has an abdomen and thorax ranging in colour from metallic green to copper (Figure 13.7a, b) with a white head (fronto-orbital and parafacial regions) (Figure 13.7f). Wings are clear (Figure 13.7e), basicosta yellow not fuscous or black (Figure 13.7a–c). This species closely resembles L. sericata but can be distinguished from this species through the combination of occipital area with one (rarely 0 or 2) paravertical hairs (Figure 13.7d) and humeral callus (anterior lateral region of the thorax) with three strong bristles and very few hairs (0–4) (Figure 13.7c).
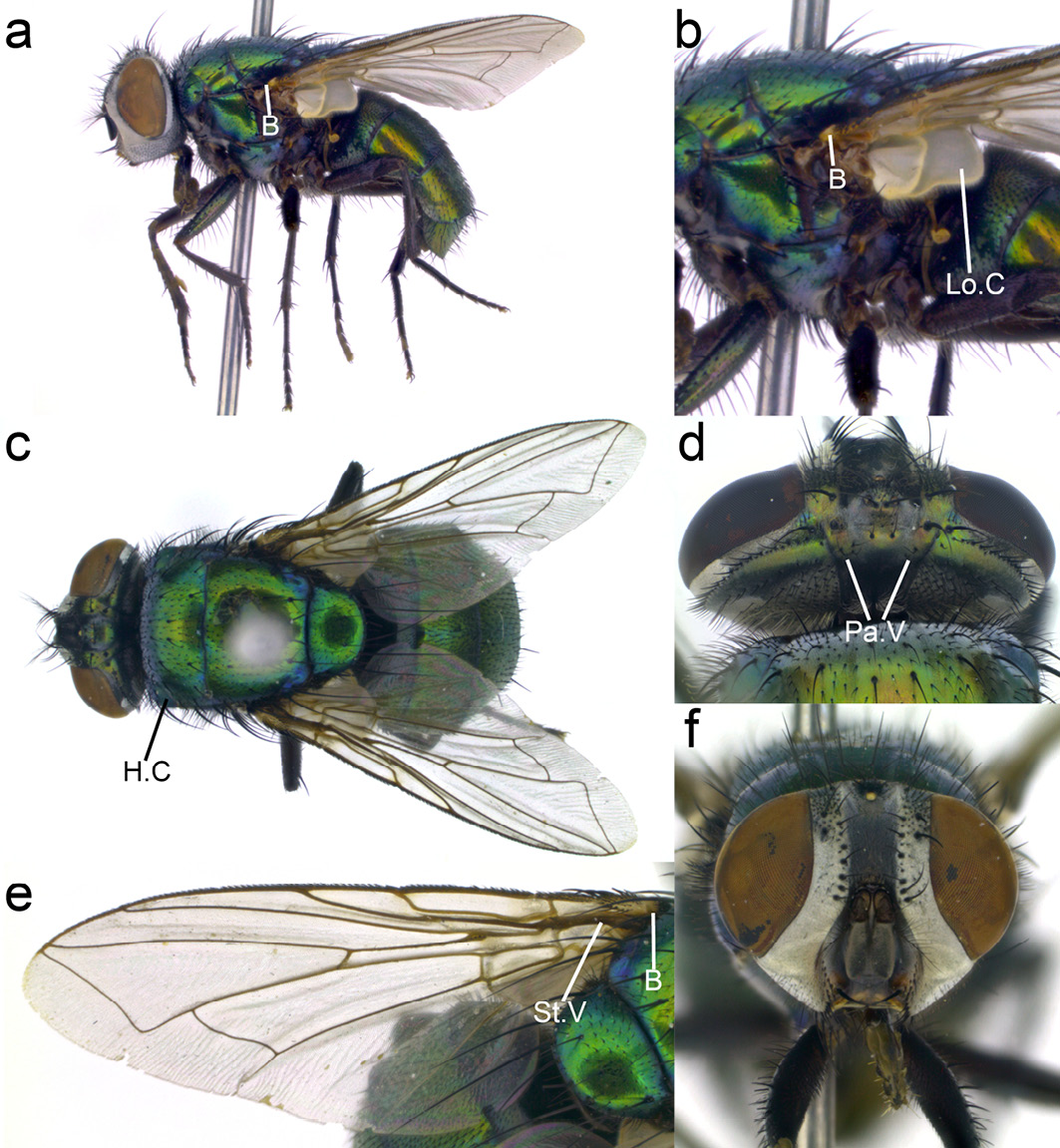
Figure 13.7 Lucilia cuprina. B: basicosta, Lo.C: lower calypter, H.C: humeral calli, Pa.V: paravertical, St.V: stem vein. CC BY-NC.
Lucilia sericata. This fly has an abdomen and thorax ranging from metallic green to copper in colour with a white head (fronto-orbital and parafacial regions) (Figure 13.8a, c, e). Wings are clear (Figure 13.8c), basicosta yellow not fuscous or black (Figure 13.8a-c). This species closely resembles L. cuprina but can be distinguished from it by the combination of central occipital area with many hairs (2–8) (Figure 13.8d) and humeral callus (anterior lateral region of the thorax) with three strong bristles and many fine hairs (>5) (Figure 13.8c).
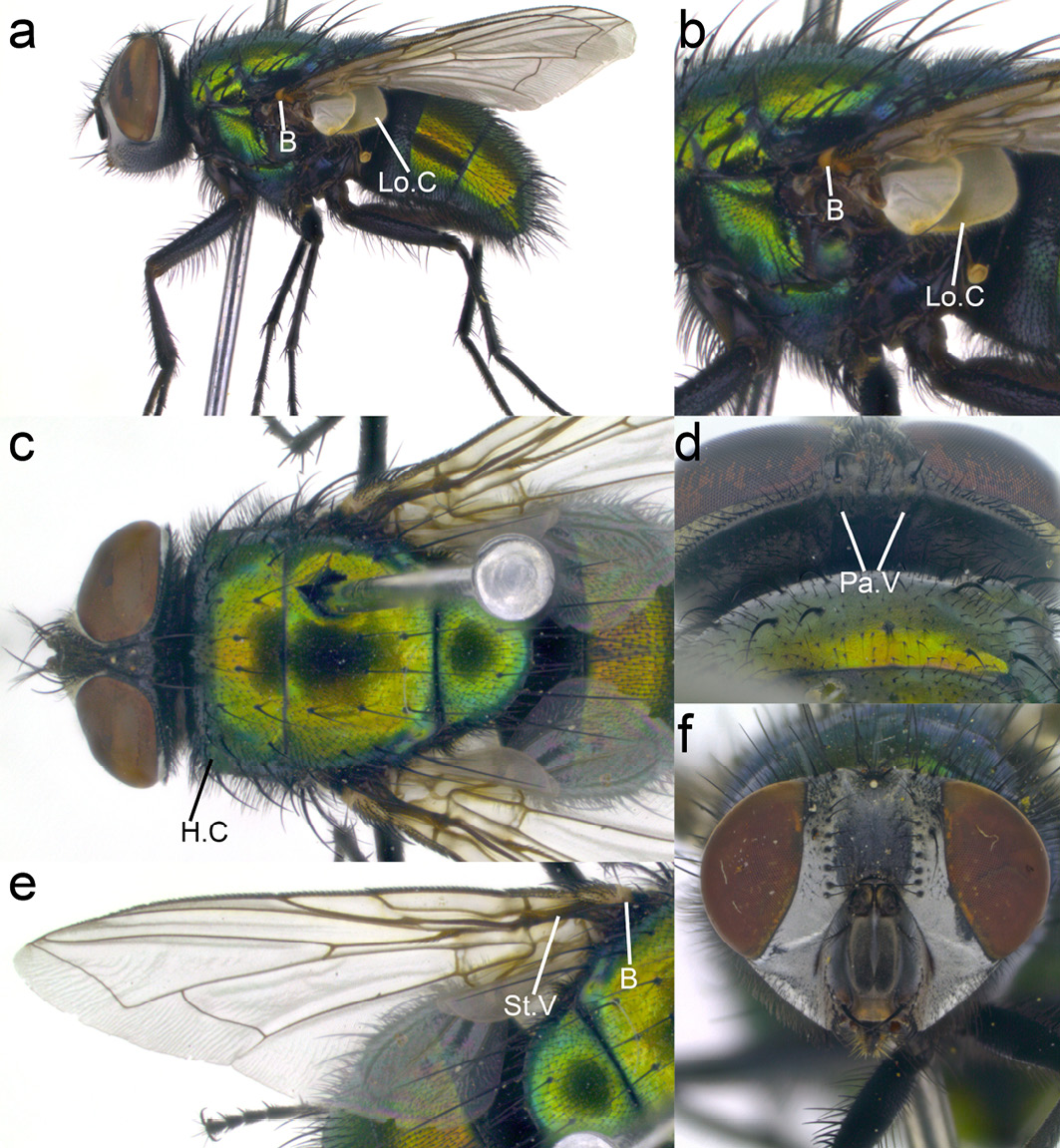
Figure 13.8 Lucilia sericata. B: basicosta, Lo.C: lower calypter, H.C: humeral calli, Pa.V: paravertical, St.V: stem vein. CC BY-NC.
Genus Cochliomyia
Genus Diagnosis. The genus Cochliomyia can be distinguished from Calliphora, Chrysomya and Lucilia by the combination of three black longitudinal stripes on the thorax (Figure 13.9c), short thread-like palpi (Figure 13.9a, f), and at least some yellow hairs on the genae (Figure 13.9a, f). It is most closely related to Chrysomya and both genera can be distinguished from Calliphora and Lucilia through the presence of a row of hairs on the dorsal surface of the stem vein (Figure 13.9e).
Cochliomya macellaria. This species can be distinguished from all other species of Cochliomya by the combination of genae with only yellow hairs (Figure 13.9a, f), fronto-orbital area with both a row of black bristles (Figure 13.9f) and fine pale hairs, particularly in the lower half (Figure 13.9a, d, f) (if fine black hairs are present, proceed with caution—the specimen could be Co. hominivorax and should NOT be used for maggot debridement therapy). The entire abdomen is metallic green or blue (with each segment being identical in colour) (Figure 13.9c).
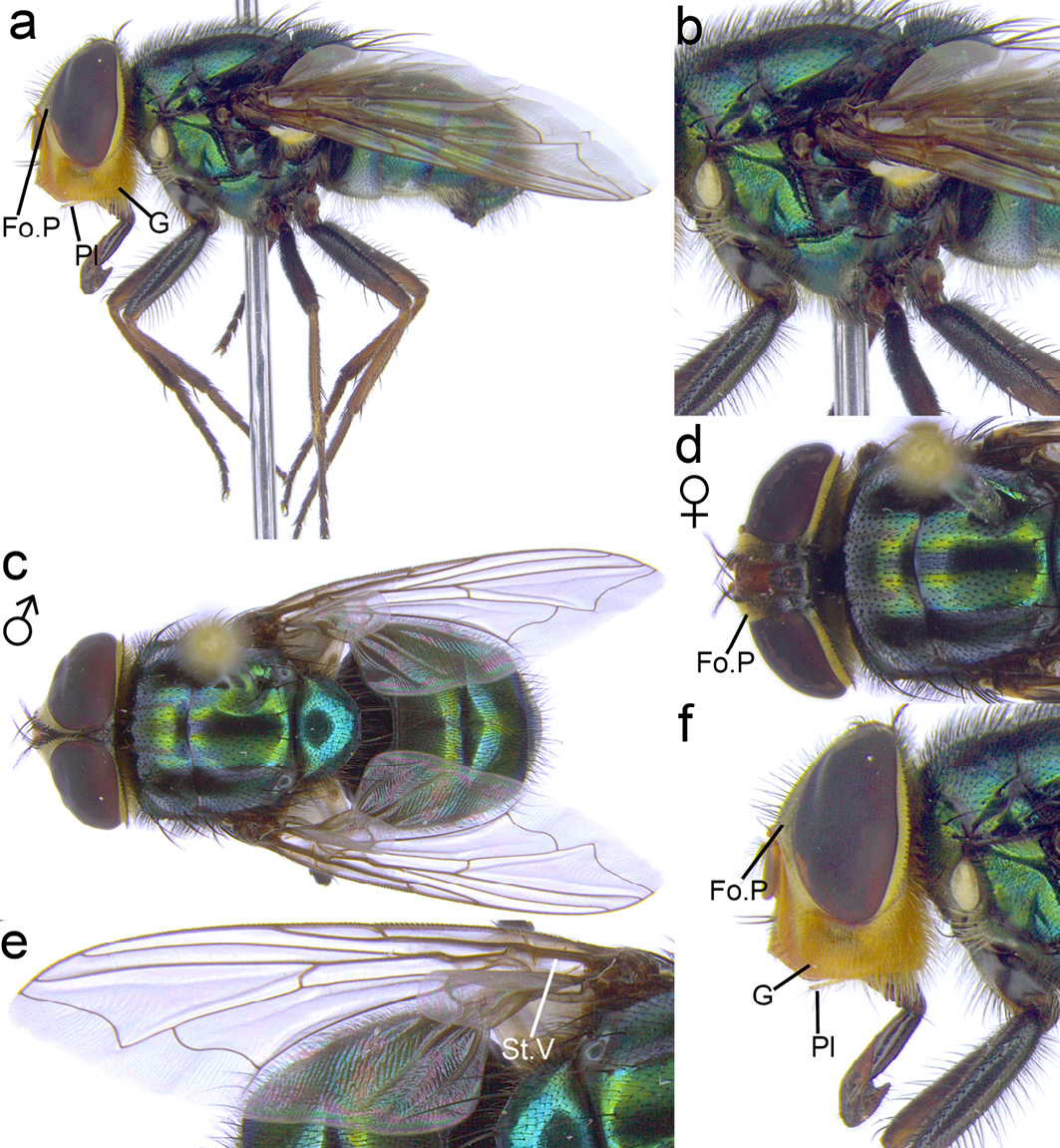
Figure 13.9 Cochliomyia macellaria. Fo.P: fronto-orbital plate, Pl: palpi, G: genae, St.V: stem vein. CC BY-NC.
Genus Chrysomya
Genus Diagnosis. The genus Chrysomya is most closely related to Cochliomya and both genera can be distinguished from Calliphora and Lucilia by the presence of a row of hairs on the dorsal surface of the stem vein (Figure 13.10e). Chrysomya can be distinguished from Cochliomya by the absence of any longitudinal black stripes on the thorax (Figure 13.10c).
Chrysomya megacephala. This species can be distinguished from other Chrysomya by the combination of entirely clear wings (Figure 13.10e), a blackish-brown anterior spiracle (Figure 13.10b), lower calypter brownish (not white or black) (Figure 13.10b), and many black vibrissal setulae on the face and parafacialia (Figure 13.10f). Males of this species possess touching eyes (holoptic) with sharply distinguished upper and lower facets (Figure 13.10c). In the female, the eyes are separated (dichoptic) (Figure 13.10d), and the fronto-orbital plate is dark rather than red (Figure 13.10d).
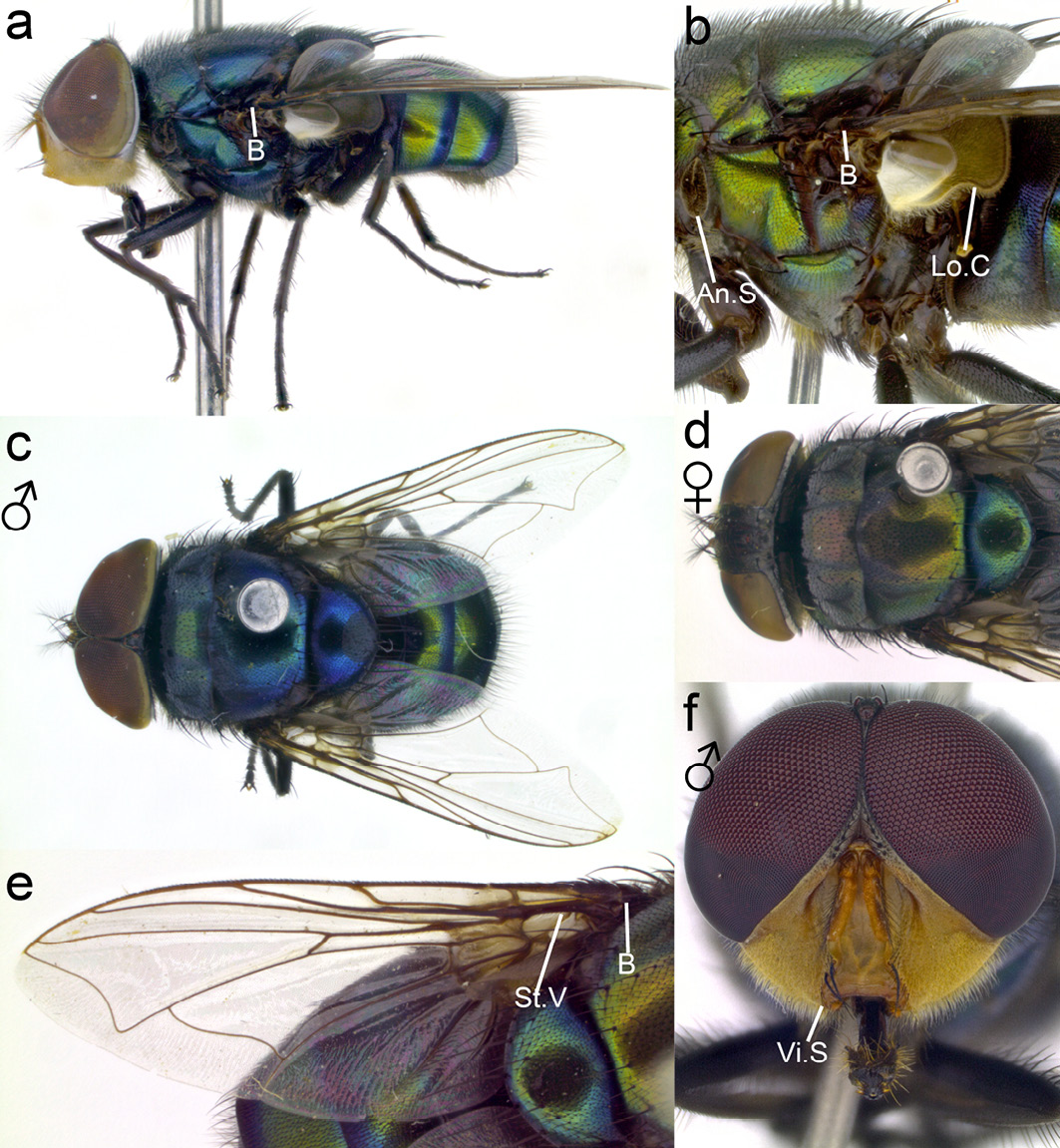
Figure 13.10 Chrysomya megacephala. B: basicosta, An.S: anterior spiracle, Lo.C: lower calypter, St.V: stem vein, Vi.S: vibrissal setulae. CC BY-NC.
Colony Replenishment
While some laboratory colonies of fly species that are of interest to forensic, agricultural, or medical entomologists have been kept successfully for many years without adding wild flies to the colonies [4], it may be necessary to periodically restock in order to replenish colony genetics and vigour. For consistent performance of production colonies, it will be best to always collect replenishment stock from the same location as that of the founder colony [79–81]. This will avoid introduction of vastly different genetics and resulting life history traits. The aim is to maintain uniform fly performance characteristics in the laboratory.
When collecting from the wild, there is always a danger that flies have been exposed to toxins, and carry disease or parasites, making preliminary quarantine necessary. Careless introduction of these newly caught flies into the laboratory environment can harm established colonies. Many flies including L. sericata are also prone to pupal parasites [82] and various fungi and nematodes that kill insects. These diseases must not be introduced into the laboratory and insectary [83, 84]. In practical terms this means that newly collected flies should be kept apart from the established laboratory colonies for at least one life-cycle until their health has been confirmed.
Domestication of Fly Stock
Domestication is the process of cultivating or rearing plant and animal species for human use, pleasure or companionship. During domestication, the animal or plant population adjusts to the new environmental conditions under human cultivation. This is also the case when wild flies are brought into the laboratory. They will need to adjust to the laboratory environment, constant temperature and light regimens, diets, cages, and higher population densities. Initially, individuals of newly collected fly stock will vary in their life history characteristics such as development times, number of eggs produced and timing of maturation. Over a number of generations and as laboratory processes impose selection pressure on the fly population, this variation will diminish, performance will become more and more uniform, and fitness may even increase [85], provided the negative effects of inbreeding can be managed.
The objectives regarding colony performance may vary depending on the purpose of the colony. For example, if flies are to be reared in the laboratory for the purpose of controlled-release field experiments or pest control, it will be important to maintain their natural fitness and resilience in the field [86]. In contrast, the objectives for medicinal fly production are:
- Optimisation of the therapeutic performance of medicinal fly species or strains.
- Reliability of colony performance via homogenous life history traits, meaning that the individuals in the colony have very similar development times and growth rates, for example.
- Maximisation of the number of eggs produced by females.
Unfortunately, there has not been much published research on optimal domestication and management of medicinal fly populations in the laboratory and insectary [87]. Much of this information and practical knowledge, as far as it exists, is of proprietary nature and held by medicinal maggot producers. Chapter 14 provides comprehensive guidance on how medicinal flies are best maintained in the insectary and how medicinal maggots are prepared for treatment [88].
Quality Control
Newly collected flies and established colonies should be carefully monitored, and their life history parameters measured on a regular basis. Species-specific characteristics such as morphology, life-history, physiology, and genetic characteristics can all be used as performance indicators. Which sampling regimen and performance indicators are chosen will depend on the producer’s resources. For example, it is fairly easy to measure how many eggs females produce, how many of these hatch, how fast maggots grow and how many survive to adulthood. Table 13.4 lists some easily monitored quality control activities that may be included in the standard operating procedures. However, wing morphology or genetic investigations are more complex and demand sophisticated instrumentation, software, and skilled laboratory technicians that may not be available to all producers [89–91].
The easiest way for a producer to monitor therapeutic efficacy of medicinal maggots over time is to communicate frequently with maggot therapy practitioners to receive feedback on clinical performance. This must also include an adverse-events reporting system so that any potential quality issues that have led to adverse treatment outcomes can be addressed quickly. The therapeutic performance with respect to debridement can also be tested in the laboratory with a meat-based test that simulates debridement in a wound. A protocol for such a debridement activity assay is given by Thyssen and Masiero [13] in Chapter 11 based on studies published in the literature.
Table 13.4 Quality control activities.
Life history characteristic |
Measurement |
Adult fly performance |
|
Sex ratio |
Ratio of males to females. Determine the sex of 100 randomly sampled adult flies. |
Adult weight |
Measure the weight of adults directly after emergence and before first meal. There should be a strong correlation between this weight and that of pupae. |
First oviposition |
Time (hours) it takes from emergence for females to lay their first egg mass (assuming that males are present for mating as soon as females are receptive) |
Number of oviposition events |
Number of times a female lays eggs (oviposition). |
Egg mass size |
Number of eggs per oviposition (per egg batch). |
Fecundity |
Total number of eggs laid in a lifetime. |
Adult longevity |
Life span (days) of an adult fly from emergence. |
Egg development |
|
Development time |
Time (hours) from oviposition to emergence of larvae at a specific temperature. |
Survival rate (in %) |
Number of emerged larvae divided by the total number of eggs incubated and multiplied by 100. |
Larval development |
|
Development time |
Time (hours) from hatching to post-feeding period (when they leave the larval food substrate). |
Body length at post-feeding |
Length of maggots (mm) at the beginning of the post-feeding period. Immerse maggots in boiling water to rapidly kill them and then preserve in 70–80% ethanol prior to measurement [92]. |
Weight at post-feeding |
Weight of maggots (mg) at beginning of post-feeding period. |
Time to pupariation |
Time (hours) it takes for post-feeding maggots to pupariate. |
Pupariation rate (in %) |
Number of pupariated maggots divided by the total number of maggots studied, multiplied by 100. |
Pupal development |
|
Pupal weight |
Weight (mg) of pupae just after pupariation (when puparia have hardened and darkened to a glossy brown colour). |
Development time |
Time (hours) it takes for the fly to emerge after pupariation. |
Emergence rate (in %) |
Number of healthy flies divided by the number of pupae studied, multiplied by 100. |
Strain Improvement
In addition to selecting suitable species with maggots that are benign and highly effective in debriding necrotic tissue, clinical performance could also be improved, and new therapeutic benefits introduced to maggot therapy, if fly strains were to be genetically enhanced. Traditionally, such enhancement is done through animal breeding based on selecting individuals with favourable characteristics. There have been recent attempts to genetically engineer L. sericata so that the flies express human growth hormone in larval excretions and secretions. Such genetically modified strains could deliver a variety of growth factors and anti-microbial substances during maggot therapy and thereby enhance wound healing [93]. However, due to the negative perception of genetic modification, regulatory approval for genetically modified flies may be difficult to obtain even if in vitro and clinical trials demonstrate efficacy. Therefore, until regulatory barriers have been overcome, the best strategy will be to improve medicinal maggot performance and efficacy through conventional animal breeding approaches.
Summary
There are potentially many fly species that could be utilised for maggot therapy and several species have been successfully applied to treat wounds. Nevertheless, it is recommended that producers and researchers starting medicinal fly colonies only collect species of known efficacy. For the most part this will involve the production of L. sericata and L. cuprina thanks to their near-global distribution. Producers and therapists interested in developing maggot therapy with other fly species will find Chapter 11 on the identification and testing of new maggot therapy species essential reading [13].
All life stages are suitable for collection, except pupae that are generally hidden from view because maggots bury into the ground for pupariation. Correct identification of the species that are collected and the correct selection of breeding stock is critical. Identification guides provided in this chapter and identification keys published in the literature should be consulted. In addition, identification services offered by natural history museums or molecular barcoding methods should be used where accessible to confirm species identity.
Domestication of the newly established fly colony will proceed via adaptation to the laboratory/insectary environment and the producer’s operating procedures. Producers must implement routine quality-monitoring protocols during colony establishment and, beyond that, track life history characteristics like the ones suggested in Table 13.4. There is largely unexplored scope for selective breeding of desirable characteristics of medicinal fly species and molecular tools may also offer opportunities for genetic enhancement of medicinal flies. However, the latter will require considerable research, regulatory approval and patient acceptance before transgenic maggots reach the bedside.
References
1. Firoozfar, F., et al., Mass Rearing of Lucilia sericata Meigen (Diptera: Calliphoridae). Asian Pacific Journal of Tropical Biomedicine, 2011. 1(1): pp. 54–56, https://doi.org/10.1016/S2221-1691(11)60068-3.
2. Firoozfar, F., et al., Laboratory Colonization of Lucilia sericata Meigen (Diptera: Caliphoridae) strain from Hashtgerd, Iran. Journal of Vector Borne Diseases, 2012. 49(1): pp. 23–26.
3. Sanei-Dehkordi, A., et al., Anti Leishmania Activity of Lucilia sericata and Calliphora vicina Maggots in Laboratory Models. Experimental Parasitology, 2016. 170: pp. 59–65, https://doi.org/10.1016/j.exppara.2016.08.007.
4. Leopold, R.A., R.R. Rojas, and P.W. Atkinson, Post Pupariation Cold Storage of Three Species of Flies: Increasing Chilling Tolerance by Acclimation and Recurrent Recovery Periods. Cryobiology, 1998. 36(3): pp. 213–224, https://dx.doi.org/10.1006/cryo.1998.2081.
5. Pinilla, Y.T., M.A. Patarroyo, and F.J. Bello, Sarconesiopsis magellanica (Diptera: Calliphoridae) Life-cycle, Reproductive and Population Parameters Using Different Diets under Laboratory Conditions. Forensic Science International, 2013. 233(1–3): pp. 380–386, https://doi.org/10.1016/j.forsciint.2013.10.014.
6. Anderson, G.S., Minimum and Maximum Development Rates of Some Forensically Important Calliphoridae (Diptera). Journal of Forensic Sciences, 2000. 45(4): pp. 824–832.
7. Grassberger, M. and C. Reiter, Effect of Temperature on Lucilia sericata (Diptera: Calliphoridae) Development with Special Reference to the Isomegalen- and Isomorphen-diagram. Forensic Science International, 2001. 120(1–2): pp. 32–36, https://doi.org/10.1016/S0379-0738(01)00413-3.
8. Blake, F.A.S., et al., The Biosurgical Wound Debridement: Experimental Investigation of Efficiency and Practicability. Wound Repair and Regeneration, 2007. 15(5): pp. 756–761, https://doi.org/10.1111/j.1524-475x.2007.00298.x.
9. McGuiness, W., E. Vella, and D. Harrison, Influence of Dressing Changes on Wound Temperature. Journal of Wound Care, 2004. 13(9): pp. 383–385, 10.12968/jowc.2004.13.9.26702.
10. Dini, V., et al., Correlation between Wound Temperature Obtained with an Infrared Camera and Clinical Wound Bed Score in Venous Leg Ulcers. Wounds, 2015. 27(10): pp. 274–278.
11. US Army, ST 31–91B US Army Special Forces Medical Handbook. 1982: United States Army Institute for Military Assistance.
12. Sherman, R.A. and M.R. Hetzler, Maggot Therapy for Wound Care in Austere Environments. Journal of Special Operations Medicine, 2017. 17(2): pp. 154–162.
13. Thyssen, P.J. and F.S. Masiero, Bioprospecting and Testing of New Fly Species for Maggot Therapy, in A Complete Guide to Maggot Therapy: Clinical Practice, Therapeutic Principles, Production, Distribution, and Ethics, F. Stadler (ed.). 2022, Cambridge: Open Book Publishers, pp. 195–234, https://doi.org/10.11647/OBP.0300.11.
14. Chan, Q.E., M.A. Hussain, and V. Milovic, Eating out of the Hand, Maggots — Friend or Foe? Journal of Plastic, Reconstructive and Aesthetic Surgery, 2012. 65(8): pp. 1116–1118, https://doi.org/10.1016/j.bjps.2012.01.014.
15. Giri, S.A., et al., Cerebral Myiasis Associated with Artificial Cranioplasty Flap: A Case Report. World Neurosurg, 2016. 87: p. 661.e13–6, https://doi.org/10.1016/j.wneu.2015.09.046.
16. Terterov, S., et al., Posttraumatic Human Cerebral Myiasis. World Neurosurgery, 2010. 73(5): pp. 557–559, https://doi.org/10.1016/j.wneu.2010.01.004.
17. Bickel, D.J., T. Pape, and R. Meier, Appendix. Diptera per Family for All Regions, in Diptera Diversity: Status Challenges and Tools, D.J. Bickel, T. Pape, and R. Meier, Editors. 2009, Brill: Boston; Leiden. pp. 439–444.
18. Paul, A.G., et al., Maggot Debridement Therapy with Lucilia cuprina: A Comparison with Conventional Debridement in Diabetic Foot Ulcers. International Wound Journal, 2009. 6(1): pp. 39–46, https://doi.org/10.1111/j.1742-481X.2008.00564.x.
19. Yeong, Y.S., et al., Scanning Electron Microscopic Evaluation of the Successful Sterilization of Lucilia cuprina (Wiedemann) Utilized in Maggot Debridement Therapy (mdt). Tropical Biomedicine, 2011. 28(2): pp. 325–332. https://www.msptm.org/files/325_-_332_Yeong_Y_S.pdf.
20. Tantawi, T.I., K.A. Williams, and M.H. Villet, An Accidental but Safe and Effective Use of Lucilia cuprina (Diptera: Calliphoridae) in Maggot Debridement Therapy in Alexandria, Egypt. Journal of Medical Entomology, 2010. 47(3): pp. 491–494, https://doi.org/10.1093/jmedent/47.3.491.
21. Nassu, M.P. and P.J. Thyssen, Evaluation of Larval Density Cochliomyia macellaria F. (Diptera: Calliphoridae) for Therapeutic Use in the Recovery of Tegumentar Injuries. Parasitology Research, 2015. 114(9): pp. 3255–3260, https://doi.org/10.1007/s00436-015-4542-8.
22. Alvarez Garcia, D.M., A. Pérez-Hérazo, and E. Amat, Life History of Cochliomyia macellaria (Fabricius, 1775) (Diptera, Calliphoridae), a Blowfly of Medical and Forensic Importance. Neotropical Entomology, 2017. 46(6): pp. 606–612, https://doi.org/10.1007/s13744-017-0496-0.
23. Dallavecchia, D.L., R.G. da Silva Filho, and V.M. Aguiar, Sterilization of Chrysomya putoria (Insecta: Diptera: Calliphoridae) Eggs for Use in Biotherapy. Journal of Insect Science, 2014. 14, https://doi.org/10.1093/jisesa/ieu022.
24. Diaz-Roa, A., et al., Evaluating Sarconesiopsis magellanica Blowfly-derived Larval Therapy and Comparing it to Lucilia sericata-derived Therapy in an Animal Model. Acta Tropica, 2016. 154: pp. 34–41, https://doi.org/10.1016/j.actatropica.2015.10.024.
25. Teich, S. and R.A.M. Myers, Maggot Therapy for Severe Skin Infections. Southern Medical Journal, 1986. 79(9): pp. 1153–1155.
26. Baer, W.S., The Treatment of Chronic Osteomyelitis with the Maggot (Larva of the Blow Fly). The Journal of Bone and Joint Surgery. American Volume, 1931. 13: pp. 438–475.
27. Sherman, R.A. and E.A. Pechter, Maggot Therapy: A Review of the Therapeutic Applications of Fly Larvae in Human Medicine, Especially for Treating Osteomyelitis. Medical and Veterinary Entomology, 1988. 2(3): pp. 225–230, https://doi.org/10.1111/j.1365-2915.1988.tb00188.x.
28. Andersen, A.S., et al., A Novel Approach to the Antimicrobial Activity of Maggot Debridement Therapy. The Journal of Antimicrobial Chemotherapy, 2010. 65(8): pp. 1646–1654, https://doi.org/10.1093/jac/dkq165.
29. Sherman, R.A., M.J.R. Hall, and S. Thomas, Medicinal Maggots: An Ancient Remedy for Some Contemporary Afflictions. Annual Review of Entomology, 2000. 45(1): pp. 55–81, https://doi.org/10.1146/annurev.ento.45.1.55.
30. Sun, X., et al., A Systematic Review of Maggot Debridement Therapy for Chronically Infected Wounds and Ulcers. International Journal of Infectious Diseases, 2014. 25: pp. 32–37, https://doi.org/10.1016/j.ijid.2014.03.1397.
31. Nair, H.K.R., et al., Maggot Debridement Therapy in Malaysia. The International Journal of Lower Extremity Wounds, 2020: p. 1534734620932397, https://doi.org/10.1177/1534734620932397.
32. Paul, A.G., et al., Maggot Debridement Therapy with Lucilia cuprina: A Comparison with Conventional Debridement in Diabetic Foot Ulcers. International Wound Journal, 2009. 6(1): pp. 39–46, https://doi.org/10.1111/j.1742-481X.2008.00564.x.
33. Yeong, Y.S., et al., Scanning Electron Microscopic Evaluation of the Successful Sterilization of Lucilia cuprina (Wiedemann) Utilized in Maggot Debridement Therapy (mdt). Tropical Biomedicine, 2011. 28(2): pp. 325–332.
34. Horn, K.L., A.H. Cobb, and G.A. Gates, Maggot Therapy for Subacute Mastoiditis. Archives of otolaryngology (1960), 1976. 102(6): pp. 377–379, https://doi.org/10.1001/archotol.1976.00780110089013.
35. Durán, D., et al., Histological and Immunohistochemical Study of Wounds in Sheep Skin in Maggot Therapy by Using Protophormia terraenovae (Diptera: Calliphoridae) Larvae. Journal of Medical Entomology, 2019. 57(2): pp. 369–376, https://doi.org/10.1093/jme/tjz185.
36. Pinheiro, M.A.R.Q., et al., Use of Maggot Therapy for Treating a Diabetic Foot Ulcer Colonized by Multidrug Resistant Bacteria in Brazil. The Indian Journal of Medical Research, 2015. 141(3): pp. 340–342, https://doi.org/10.4103/0971-5916.156628.
37. Masiero, F.S., et al., First Report on the Use of Larvae of Cochliomyia macellaria (Diptera: Calliphoridae) for Wound Treatment in Veterinary Practice. Journal of Medical Entomology, 2019. 57(3): pp. 965–968, https://doi.org/10.1093/jme/tjz238.
38. Grantham-hill, C., Preliminary Note on the Treatment of Infected Wounds with the Larva of Wohlfartia nuba. Transactions of the Royal Society of Tropical Medicine and Hygiene, 1933. 27(1): pp. 93–98, https://doi.org/10.1016/S0035-9203(33)90138-8.
39. Li, Q., et al., Maggots of Musca domestica in Treatment of Acute Intractable Wound. Surgery, 2009. 145(1): pp. 122–123, https://doi.org/10.1016/j.surg.2008.08.016.
40. Gallagher, M.B., S. Sandhu, and R. Kimsey, Variation in Developmental Time for Geographically Distinct Populations of the Common Green Bottle Fly, Lucilia sericata (Meigen). Journal of Forensic Sciences, 2010. 55(2): pp. 438–442, https://doi.org/10.1111/j.1556-4029.2009.01285.x.
41. El-Moaty, Z.A. and A.E.M. Kheirallah, Developmental Variation of the Blow Fly Lucilia sericata (Meigen, 1826) (diptera: Calliphoridae) by Different Substrate Tissue Types. Journal of Asia-Pacific Entomology, 2013. 16(3): pp. 297–300, https://doi.org/10.1016/j.aspen.2013.03.008.
42. Warren, J.A. and G.S. Anderson, Effect of Fluctuating Temperatures on the Development of a Forensically Important Blow Fly, Protophormia terraenovae (Diptera: Calliphoridae). Environmental Entomology, 2013. 42(1): pp. 167–172, https://doi.org/10.1603/EN12123.
43. Klong-Klaew, T., et al., Impact of Abiotic Factor Changes in Blowfly, Achoetandrus rufifacies (Diptera: Calliphoridae), in Northern Thailand. Parasitology Research, 2014. 113(4): pp. 1353–1360, https://doi.org/10.1007/s00436-014-3774-3.
44. Lindsay, T.C., et al., Development of Odour-Baited Flytraps for Sampling the African Latrine Fly, Chrysomya putoria, a Putative Vector of Enteric Diseases. PLoS ONE, 2012. 7(11), https://doi.org/10.1371/journal.pone.0050505.
45. Warren, J.A. and G.S. Anderson, The Development of Protophormia terraenovae (Robineau-Desvoidy) at Constant Temperatures and Its Minimum Temperature Threshold. Forensic Science International, 2013. 233(1–3): pp. 374–379, https://doi.org/10.1016/j.forsciint.2013.10.012.
46. Harvey, M., et al., Dipteran Attraction to a Variety of Baits: Implications for Trapping Studies as a Tool for Establishing Seasonal Presence of Significant Species. Journal of Medical Entomology, 2019. 56(5): pp. 1283–1289, https://dx.doi.org/10.1093/jme/tjz050.
47. Brodie, B.S., et al., Is Aggregated Oviposition by the Blow Flies Lucilia sericata and Phormia regina (Diptera: Calliphoridae) Really Pheromone-mediated? Insect Science, 2015. 22(5): pp. 651–660, https://doi.org/10.1111/1744-7917.12160.
48. Hwang, C. and B.D. Turner, Spatial and Temporal Variability of Necrophagous Diptera from Urban to Rural Areas. Medical and Veterinary Entomology, 2005. 19(4): pp. 379–391, https://doi.org/10.1111/j.1365-2915.2005.00583.x.
49. Zabala, J., B. Díaz, and M.I. Saloña-Bordas, Seasonal Blowfly Distribution and Abundance in Fragmented Landscapes. Is It Useful in Forensic Inference about Where a Corpse Has Been Decaying? PloS one, 2014. 9(6): e99668, https://doi.org/10.1371/journal.pone.0099668.
50. Hall, M.J.R., Trapping the Flies that Cause Myiasis: Their Responses to Host-stimuli. Annals of Tropical Medicine and Parasitology, 1995. 89(4): pp. 333–357.
51. BioGlobal. LuciLure Sheep Blowfly Attractant. www.bioglobal.com.au/images/stories/lucilow.pdf.
52. Akbarzadeh, K., et al., Species Identification of Middle Eastern Blowflies (Diptera: Calliphoridae) of forensic importance. Parasitology Research, 2015. 114(4): pp. 1463–1472, https://doi.org/10.1007/s00436-015-4329-y.
53. Langer, S.V., C.J. Kyle, and D.V. Beresford, Using Frons Width to Differentiate Blow Fly Species (Diptera: Calliphoridae) Phormia regina (Meigen) and Protophormia terraenovae (Robineau-Desvoidy). Journal of Forensic Sciences, 2017. 62(2): pp. 473–475, https://doi.org/10.1111/1556-4029.13281.
54. Szpila, K. and J.F. Wallman, Morphology and Identification of First Instar Larvae of Australian Blowflies of the Genus Chrysomya of Forensic Importance. Acta Tropica, 2016. 162: pp. 146–154, https://doi.org/10.1016/j.actatropica.2016.06.006.
55. Tourle, R., D. Downie, and M.H. Villet, Flies in the Ointment: A Morphological and Molecular Comparison of Lucilia cuprina and Lucilia sericata (Diptera: Calliphoridae) in South Africa. Medical and Veterinary Entomology, 2009. 23(1): pp. 6–14, http://dx.doi.org/10.1111/j.1365-2915.2008.00765.x.
56. Vairo, K.P.e., C.A.d. Mello-Patiu, and C.J.B.d. Carvalho, Pictorial Identification Key for Species of Sarcophagidae (Diptera) of Potential Forensic Importance in Southern Brazil. Revista Brasileira de Entomologia, 2011. 55(3): pp. 333–347, https://doi.org/10.1590/S0085-56262011005000033.
57. Waterhouse, D.F. and S.J. Paramonov, The Status of the Two Species of Lucilia (Diptera: Calliphoridae) Attacking Sheep in Australia. Australian Journal of Biological Sciences, 1950. 3(3): pp. 310–336, http://dx.doi.org/10.1071/BI9500310.
58. Sivell, O., Blow Flies (Diptera: Calliphoridae, Polleniidae, Rhiniidae), Handbooks for the Identification of British Insects 10 (16). 2021, St Albans: Royal Entomological Society.
59. Erzinclioglu, Y.Z., The Early Larval Instars of Lucilia sericata and Lucilia cuprina (Diptera, Calliphoridae) — Myiasis Blowflies of Africa and Australia. Journal of Natural History, 1989. 23(5): pp. 1133–1136, https://doi.org/10.1080/00222938900771021.
60. Niederegger, S., K. Szpila, and G. Mall, Muscle Attachment Site (MAS) Patterns for Species Determination in European Species of Lucilia (Diptera: Calliphoridae). Parasitology Research, 2015. 114(3): pp. 851–859, https://doi.org/10.1007/s00436-014-4248-3.
61. Niederegger, S., et al., Connecting the Dots: From an Easy Method to Computerized Species Determination. Insects, 2017. 8(2): pp. 1–16, https://doi.org/10.3390/insects8020052.
62. Niederegger, S., K. Szpila, and G. Mall, Muscle Attachment Site (MAS) Patterns for Species Determination in Five Species of Sarcophaga (Diptera: Sarcophagidae). Parasitology Research, 2016. 115(1): pp. 241–247, https://doi.org/10.1007/s00436-015-4740-4.
63. Samerjai, C., et al., Morphology of Puparia of Flesh Flies in Thailand. Tropical Biomedicine, 2014. 31(2): pp. 351–361 https://www.msptm.org/files/351_-_361_Sukontason_KL.pdf.
64. Holloway, B.A., Morphological Characteristics to Identify Adult Lucilia sericata (Meigen, 1826) and L. cuprina (Wiedemann, 1830) (Diptera, Calliphoridae). New Zealand Journal of Zoology, 1991. 18(4): pp. 415–420, https://dx.doi.org/10.1080/03014223.1991.10422847.
65. Olekšáková, T., et al., DNA Extraction and Barcode Identification of Development Stages of Forensically Important Flies in the Czech Republic. Mitochondrial DNA Part A: DNA Mapping, Sequencing, and Analysis, 2017. 29(3): pp. 427–430, https://doi.org/10.1080/24701394.2017.1298102.
66. Salem, A.M., F.K. Adham, and C.J. Picard, Survey of the Genetic Diversity of Forensically Important Chrysomya (diptera: Calliphoridae) from Egypt. Journal of Medical Entomology, 2015. 52(3): pp. 320–328, https://doi.org/10.1093/jme/tjv013.
67. Williams, K.A., et al., Identifying Flies Used for Maggot Debridement Therapy. South African Medical Journal, 2008. 98(3): pp. 196–197.
68. Shayya, S., et al., Forensically Relevant Blow Flies in Lebanon Survey and Identification Using Molecular Markers (Diptera: Calliphoridae). Journal of medical entomology, 2018. 55(5): pp. 1113–1123, https://doi.org/10.1093/jme/tjy068.
69. Lutz, L., et al., Species Identification of Adult African Blowflies (Diptera: Calliphoridae) of Forensic Importance. International Journal of Legal Medicine, 2018. 132(3): pp. 831–842, https://doi.org/10.1007/s00414-017-1654-y.
70. Kurahashi, H. and N. Bunchu, The Blow Flies Recorded from Thailand, with the Description of a New Species of Isomyia WALKER (Diptera, Calliphoridae). Japanese Journal of Systematic Entomology, 2011. 17: pp. 237–278.
71. Kurahashi, H. and L. Chowanadisai, Blow Flies (Insecta: Diptera: Calliphoridae) from Indochina. Species Diversity, 2001. 6(3): pp. 185–242.
72. Yang, S.-T., H. Kurahashi, and S.-F. Shiao, Keys to the Blow Flies of Taiwan, with a Checklist of Recorded Species and the Description of a New Species of Paradichosia Senior-White (Diptera, Calliphoridae). ZooKeys, 2014(434): pp. 57–109, https://doi.org/10.3897/zookeys.434.7540.
73. Rognes, K., Blowflies (Diptera, Calliphoridae) of Fennoscandia and Denmark. Vol. 24. 1991: Brill.
74. Whitworth, T., Keys to the Genera and Species of Blow Flies (Diptera: Calliphoridae) of America North of Mexico. Proceedings of the Entomological Society of Washington, 2006. 108: pp. 689–725.
75. Whitworth, T., Keys to the Genera and Species of Blow Flies (Diptera: Calliphoridae) of the West Indies and Description of a New Species of Lucilia Robineau-Desvoidy. Zootaxa, 2010. 2663: pp. 1–35, https://doi.org/10.11646/zootaxa.2663.1.1.
76. Dear, J.P., Calliphoridae (Insecta: Diptera). Fauna of New Zealand, 1986. 8.
77. Wallman, J.F., A Key to the Adults of Species of Blowflies in Southern Australia Known or Suspected to Breed in Carrion. [corrigendum in Medical and Veterinary Entomology 16(2): 223] Medical and Veterinary Entomology, 2001. 15(4): pp. 433–437, https://doi.org/10.1046/j.0269-283x.2001.00331.x.
78. Carvalho, C.J.B.d. and C.A.d. Mello-Patiu, Key to the Adults of the Most Common Forensic Species of Diptera in South America. Revista Brasileira de Entomologia, 2008. 52: pp. 390–406.
79. Hwang, C.C. and B.D. Turner, Small-scaled Geographical Variation in Life-history Traits of the Blowfly Calliphora vicina between Rural and Urban Populations. Entomologia Experimentalis et Applicata, 2009. 132(3): pp. 218–224, https://doi.org/10.1111/j.1570-7458.2009.00891.x.
80. Martínez-Sánchez, A., et al., Geographic Origin Affects Larval Competitive Ability in European Populations of the Blow Fly, Lucilia sericata. Entomologia Experimentalis et Applicata, 2007. 122(2): pp. 93–98, https://doi.org/10.1111/j.1570-7458.2006.00497.x.
81. Picard, C.J. and J.D. Wells, The Population Genetic Structure of North American Lucilia sericata (Diptera: Calliphoridae), and the Utility of Genetic Assignment Methods for Reconstruction of Postmortem Corpse Relocation. Forensic Science International, 2010. 195(1–3): pp. 63–67, https://doi.org/10.1016/j.forsciint.2009.11.012.
82. Voss, S.C., H. Spafford, and I.R. Dadour, Temperature-dependant Development of Nasonia vitripennis on Five Forensically Important Carrion Fly Species. Entomologia Experimentalis et Applicata, 2010. 135(1): pp. 37–47, https://doi.org/10.1111/j.1570-7458.2010.00966.x.
83. Tóth, E.M., et al., Evaluation of Efficacy of Entomopathogenic Nematodes against Larvae of Lucilia sericata (Meigen, 1826) (Diptera: Calliphoridae). Acta Veterinaria Hungarica, 2005. 53(1): pp. 65–71, https://doi.org/10.1556/AVet.53.2005.1.7.
84. Wright, C., A. Brooks, and R. Wall, Toxicity of the Entomopathogenic Fungus, Metarhizium anisopliae (Deuteromycotina: Hyphomycetes) to Adult Females of the Blowfly Lucilia sericata (Diptera: Calliphoridae). Pest Management Science, 2004. 60(7): pp. 639–644, https://doi.org/10.1002/ps.808.
85. Hoffmann, A.A. and P.A. Ross, Rates and Patterns of Laboratory Adaptation in (Mostly) Insects. Journal of Economic Entomology, 2018. 111(2): pp. 501–509, https://doi.org/10.1093/jee/toy024.
86. Berkebile, D.R., et al., Laboratory Environment Effects on the Reproduction and Mortality of Adult Screwworm (Diptera: Calliphoridae). Neotropical Entomology, 2006. 35(6): pp. 781–786, https://doi.org/10.1590/S1519-566X2006000600010.
87. Stadler, F., The Maggot Therapy Supply Chain: A Review of the Literature and Practice. Med Vet Entomol, 2020. 34(1): pp. 1–9, https://doi.org/10.1111/mve.12397.
88. Stadler, F. and P. Takáč, Medicinal Maggot Production, in A Complete Guide to Maggot Therapy: Clinical Practice, Therapeutic Principles, Production, Distribution, and Ethics, F. Stadler (ed.). 2022, Cambridge: Open Book Publishers, pp. 289–330, https://doi.org/10.11647/OBP.0300.14.
89. Laparie, M., et al., Wing Morphology of the Active Flyer Calliphora vicina (Diptera: Calliphoridae) during its Invasion of a Sub-Antarctic Archipelago Where Insect Flightlessness Is the Rule. Biological Journal of the Linnean Society, 2016. 119(1): pp. 179–193, https://doi.org/10.1111/bij.12815.
90. Hayes, E.J., R. Wall, and K.E. Smith, Measurement of Age and Population Age Structure in the Blowfly, Lucilia sericata (Meigen) (Diptera: Calliphoridae). Journal of Insect Physiology, 1998. 44(10): pp. 895–901, https://doi.org/10.1016/S0022-1910(98)00067-5.
91. Diakova, A.V., et al., Assessing Genetic and Morphological Variation in Populations of Eastern European Lucilia sericata (Diptera: Calliphoridae). European Journal of Entomology, 2018. 115: pp. 192–197, https://doi.org/10.14411/eje.2018.017.
92. Adams, Z.J.O. and M.J.R. Hall, Methods Used for the Killing and Preservation of Blowfly Larvae, and Their Effect on Post-mortem Larval Length. Forensic Science International, 2003. 138: pp. 50–61.
93. Linger, R.J., et al., Towards Next Generation Maggot Debridement Therapy: Transgenic Lucilia sericata Larvae that Produce and Secrete a Human Growth Factor. BMC Biotechnology, 2016. 16:30, pp. 1–12, https://doi.org/10.1186/s12896-016-0263-z.