14. Medicinal Maggot Production
© 2022 Stadler & Takáč, CC BY-NC 4.0 https://doi.org/10.11647/OBP.0300.14
This chapter discusses the requirements for adult fly rearing, high-volume egg production, larval rearing and pupariation, and it explains the production of disinfected medicinal maggots for maggot therapy, the quality control procedures that are required to ensure safe and efficacious maggot therapy, and supply chain management considerations arising from the perishability of medicinal maggots. The chapter draws on a broad range of sources including the literature on maggot therapy, forensic entomology, and general entomology. For compromised healthcare settings with limited resources, point-of-care production solutions are discussed that do not rely on sophisticated laboratory and logistics infrastructure.
Introduction
Having covered the laboratory and insectary infrastructure and equipment needs in Chapter 12 [1], as well as the process of medicinal fly colony establishment in Chapter 13 [2], the next step is to provide the information necessary to produce high-quality, efficacious, and safe medicinal maggots. This chapter discusses the requirements for adult fly rearing, high-volume egg production, larval rearing and pupariation. The chapter also explains the production of disinfected medicinal maggots for maggot therapy, the quality control procedures that are required to ensure safe and efficacious maggot therapy, and the supply chain management considerations arising from the perishability of medicinal maggots. The information presented has been drawn from a broad range of sources such as the literature on maggot therapy, forensic entomology, and general entomology. Much of the information relates to the most commonly used medicinal fly species Lucilia sericata and Lucilia cuprina but literature on other calliphorid fly species with comparable life histories was also consulted. The information presented in this chapter will equip the novice producer with all that is needed to produce high-quality, safe, and efficacious medicinal maggots. Although the guidance provided here should ensure appropriate husbandry that optimises fly health and minimises any fly suffering, Chapter 19 of this book will discuss in more detail the animal ethics of medicinal maggot production [3].
How to Care for Adult Flies
Cages
Cage set-ups for adult flies vary from wire and gauze to cages constructed from various plastic containers covered with mesh fabric or nylon stocking, and rearing cages offered by specialist entomology suppliers (Figure 14.1). The size of cages used and, therefore, the space available for flight also varies depending on what the keeper of flies has in mind. Researchers have reared flies in cages as small as 15 cm diameter by 9 cm deep, up to large setups of 120 x 60 x 60 cm [4, 5].
Flies in the wild occur at low densities with plenty of room to avoid each other, which is impractical in captive rearing. Therefore, the relationship between fly density in cages and performance needs to be considered. Laboratory experiments seem to suggest that there is little evidence for adult competition in L. sericata [6]. It appears that high larval density (rather than high adult density) has a detrimental impact on adult longevity and reproductive output [6]. This is because the level of larval nutrition and larval size at the point of pupation determines adult body size and the number of eggs a female can produce. Large adult fly cages mean increased flight activity and therefore increased energy expenditure by flies when the objective is to direct as much of the metabolic energy as possible into egg production. In addition, the laboratory infrastructure and space availability, as well as convenience of maintenance and handling, will place limits on the practical size of cages. An important consideration in the design and size of adult fly cages is the ease with which they can be cleaned and disinfected because, over the course of a colony’s short lifetime, the cage gets badly soiled with fly faeces [7]. Considering then the health and welfare needs of flies and the practical aspects of insectary management, we recommend a stocking density of one fly per approximately 100 to 150 cm3 of cage space [8]. For example, a commercially available, off-the-shelf 47.5 x 47.5 x 47.5 cm insect cage can be stocked with approximately 1000 flies (Figure 14.1).
Cage designs for adult flies must support maintenance which means that daily changes of food and water, and the harvesting of eggs can be done quickly and easily. For this purpose, cages must have a net sleeve that is easy to open, accommodates an adult hand holding a food dish, and is easy to close once the work has been done. As opposed to hinged cage doors, the tried and tested net sleeves maintain a snug fit around hand and arm and thus largely preventing the escape of flies during maintenance. In addition, a light source at the opposite side to the cage access will draw flies away from the opening and therefore also reduce escapes.
A cost-effective option for fly cages is a plastic storage container of convenient size in which the plastic lid is replaced with a nylon panty hose (Figure 14.1). The panty hose is slipped over the container and its elastic nature provides a secure hold around the rim of the plastic container. In order to create a sleeve for maintenance work, one leg of the panty hose is cut off to leave a stump of about 20 cm and the other leg is shortened and tied off with a knot. The container can be placed either on one of its sides offering front access or placed upright as intended which will facilitate top access for maintenance. After use, the container can be easily dismantled, and the nylon stocking can be washed and sterilised in bleach for re-use. From experience, this plastic container cage works well, provided the ambient relative humidity is low and condensation or build-up of fly-generated moisture on the container walls can be avoided. If the container walls become moist the flies lose grip of the plastic surface and crash-land continuously when flying about. This leads to stress, injury, and premature colony decline.
In contrast, cages that have mesh-covered sides are well-ventilated which prevents moisture build-up. They also provide flies with sufficiently secure footing and prevent frequent crash landing. When cages are used that do not have a solid impervious floor surface then it might be necessary to place cages onto a tray of sorts to keep shelving clean, capture dust generated by fly activity and permit the handling and relocation of cages without having to remove food, water and oviposition containers.
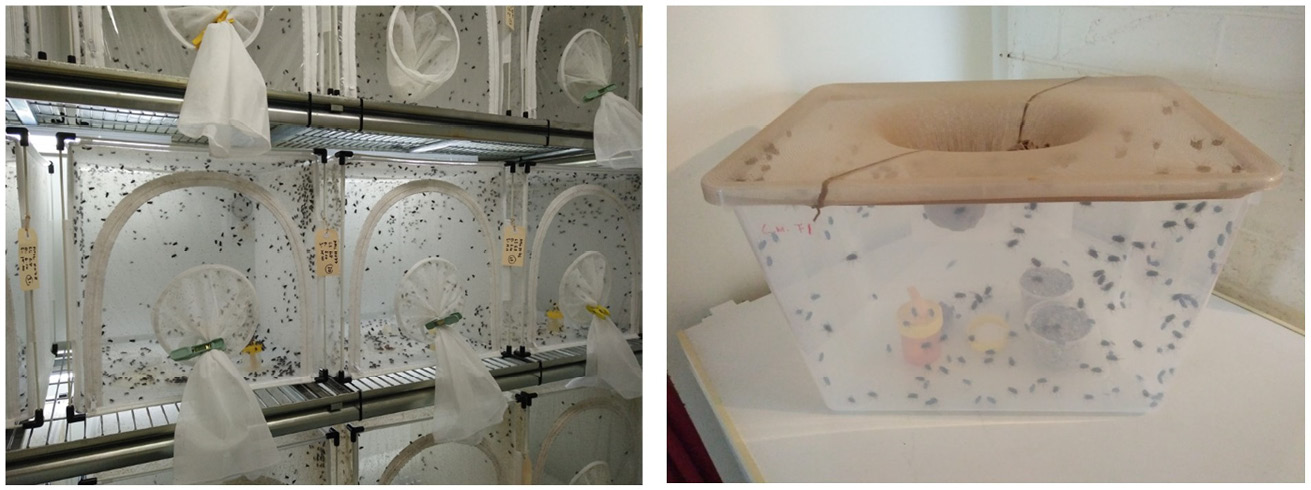
Figure 14.1 Examples of cages for the maintenance of adult fly colonies. A) A suitable cage model from an entomological equipment supplier (BugDorm Insect Cage, MegaView Science Co. Ltd.). B) A low-cost cage made of a household plastic storage box fitted with a nylon panty hose lid. Cut one panty hose leg for sleeved access to the cage. Photos by F. Stadler, MedMagLabs and Creating Hope in Conflict: A Humanitarian Grand Challenge, CC BY-ND.
Environmental Conditions
Like all organisms, medicinal fly species have their preferred environmental conditions as dictated by their evolutionary and ecological history. However, the various species used for maggot therapy (predominantly, but not exclusively, L. sericata and L. cuprina) exhibit remarkable robustness and adaptability in the laboratory environment and can be maintained at a wide range of environmental conditions, at least short-term over a few generations. However, the objective for a medicinal maggot producer is to establish and maintain highly productive fly colonies that are well-adapted to the laboratory environment and retain vigour over many generations. It is therefore important to meet the environmental and physiological needs of the flies as much as is practical.
As mentioned earlier, adult flies are best kept in cages that are well-ventilated and successful colony maintenance has been reported for relative humidity ranges from 30 to 70%. It is therefore advisable to maintain a steady humidity of 50 to 60% for adult flies, with constant provision of drinking water [7, 9, 10]. Temperature modulates activity levels in blowflies. Higher temperatures result in greater activity but shorter lifespan, and lower temperatures in less activity and decreased fertility, but a longer lifespan. In the laboratory a suitable compromise must be found that maximises the production of eggs over a relatively long period before flies age and die. The demand for reliable and predictable egg production makes it necessary to avoid any interruptions to reproductive activity. If flies are subjected to short day lengths and low temperatures, they may enter reproductive diapause and stop laying eggs. This can be avoided by providing summer climatic and photoperiodic conditions, meaning sufficiently high temperature and long day lengths [11]. A common regime is to maintain L. sericata adult colonies between 25℃ and 27℃ and at 12 to 16 hours light per day [4, 12–17]. The nature of the light provided also influences the wellbeing of flies. While regular incandescent and fluorescent light sources suffice, light sources are recommended that resemble as closely as is practical the natural daylight, particularly regarding the quantum of UV radiation.
Diet
Without access to energy-rich foods either in the form of lipids or carbohydrates, L. sericata flies expend all their energy reserves within a matter of days and die [18]. It is essential that adult flies have access at all times to carbohydrates such as table sugar or honey. In addition, females require protein meals in order to be able to mature ovaries and produce viable eggs [19–21]. Protein for ovary maturation and egg production is provided directly after adult emergence. Under natural conditions blowflies obtain protein from a variety of sources including from live animals, carcasses, or faeces. In the laboratory, flies are best fed with sugar, water, and a protein source. There is a great range of possible human foods that could be used (Table 14.1). Convenient foods include table sugar and a blend of dried protein sources such as milk powder, whey protein, and brewer’s yeast. If meat such as liver is offered, it may be presented in pieces, or blended and mixed 1:1 with wheat bran and some water. However, continuous presentation of a moist, high-protein diet will lead to females depositing eggs on these feeding stations prior to planned egg harvesting. If this is a common occurrence, then it might be best to only offer dry protein sources between egg harvests. This is perfectly feasible because flies can regurgitate digestive secretions and dissolve dry food matter for ingestion. Feeding flies with a limited range of foods may result in vitamin deficiency which may be compensated by offering the flies a weak solution of multi-vitamin supplements as used for human consumption with a feeding station as described in Figure 14.2.
There have also been efforts to develop plant-based, heat-sterilisable diets for adult L. sericata [17]. Ingredients include dried yeast, wheat germ, agar powder and water, with or without whole milk powder (Table 14.2). Although absence of a high-protein meat diet delays ovary development and oviposition, all other performance indices in relation to longevity and reproductive success are much the same. Therefore, it is important to settle on one diet, whether meat-based or not, and consistently use it in order to establish a routine with predictable life-cycle and laboratory-process timing.
It is important that flies always have access to plenty of water. However, in order to prevent drowning in water and other liquid foods, these should be offered via small plastic containers (70–200 mL) furnished with absorbent wicks or inverted on tissue paper pads as shown in Figure 14.2. Note that moist and liquid fly foods will become quickly soiled and contaminated with microbial growth. These food stations should be replaced every two to three days and either disposed of or thoroughly washed and disinfected.
Table 14.1 Food types for adult flies according to physiological need.
Protein diets |
Carbohydrate diets |
Hydration |
|
|
|
Table 14.2 Synthetic adult fly diets [17]
Meat-free adult diet with whole milk powder |
Plant-based adult diet without milk powder |
50g whole milk powder 50g dried yeast/brewer’s yeast 50g wheat germ 5g agar powder 1000 mL water |
75g dried yeast/brewer’s yeast 75g wheat germ 5g agar powder 1000 mL water |
Note: All ingredients are mixed and boiled for ten minutes. To facilitate rapid cooling and convenient handling, the diet is best divided up into portions stored in single or multi-use plastic containers. To prevent spoilage, diets are stored in the refrigerator.
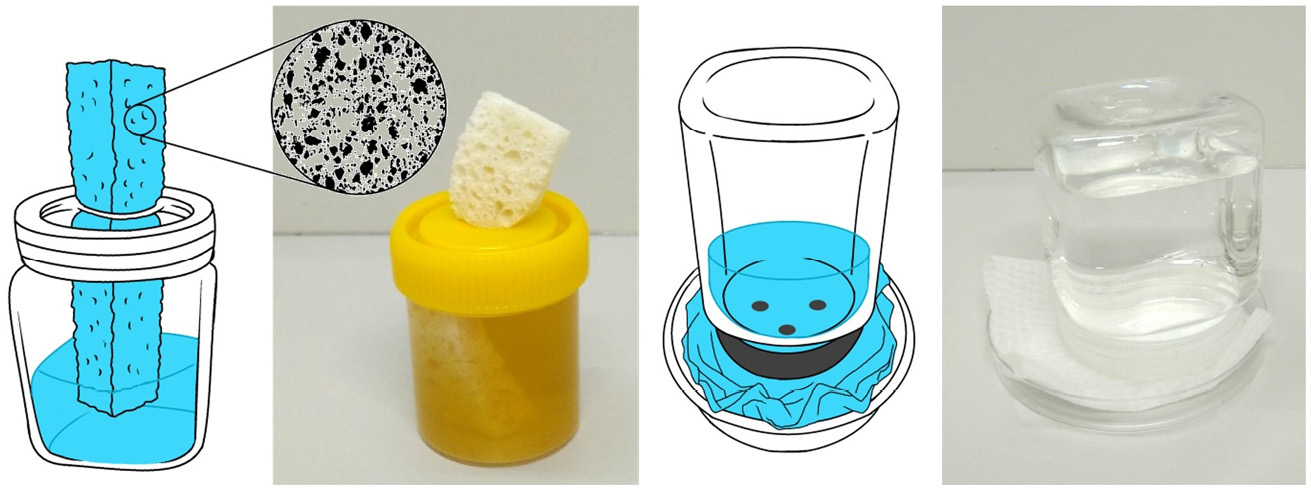
Figure 14.2 Liquid food dispensers (including water) for adult flies. A) Water and other liquids can be offered conveniently with a container fitted with a sponge wick. B) Alternatively, an inverted container with holes in the lid can be placed on a shallow dish lined with tissue paper. Solid foods like sugar or meat are best placed on shallow dishes such as reused food container lids or petri dishes. Illustrations by P. Busana and photos by F. Stadler, MedMagLabs and Creating Hope in Conflict: A Humanitarian Grand Challenge, CC BY-ND.
Egg Production
As discussed, L. sericata, L. cuprina, and other species used for maggot therapy rely on protein meals for egg development. In the case of L. cuprina, females need to consume at least 3.6 mg of liver exudate for completion of one ovarian cycle, and it is likely that similar amounts of protein are also required by closely related L. sericata flies [22]. In the event that L. sericata females cannot find enough protein as they enter reproductive maturity, they have the ability to partially invest in egg development by either depositing some yolk in all eggs followed by a pause in egg development, or by favouring the maturation of only a small number of eggs [20]. While this is an important ecological adaptation to resource insecurity and unpredictability, from an insect-rearing and egg-production standpoint, protein limitation must be avoided to maximise egg production over a female’s lifetime. Moreover, reproductive output in blowfly females not only depends on the nutrition of the adult fly, it will also vary in response to nutrition during larval life, for example in the presence of larval competition over a limited food source [23].
Female L. cuprina usually mate only once, storing the sperm required for all their eggs [24]. Males, on the other hand, seek to mate soon after emergence and throughout their lifetime [25]. Consequently, the operational sex ratio in L. cuprina is heavily male-biased, meaning that there are far more males for any one receptive female. In captivity this leads to increased sexual harassment of females, a common issue in lab-reared colonies of blowflies [26]. It would be beneficial to skew the sex ratio toward females to maximise reproductive output and longevity of females [27], but this is not practical.
There is a great variation in reproductive output between species, and between strains within species. Research on the reproductive output of L. sericata and L. cuprina found lifetime reproductive outputs between 500 and over 2000 eggs per female [28]. Eggs are deposited in batches forming egg masses. The number of batches varied between six and 13 egg masses per female. Each egg mass contains around 200 or so eggs [29]. In the laboratory at 25℃ constant temperature L. sericata could theoretically produce their first egg batch at around four to five days after emergence, and then at two- to three-day intervals, provided nutrition and all other environmental conditions are optimal [30]. However, delays to first oviposition and other life history events are to be expected because each fly strain and laboratory setup is different. Regardless, the female fly physiology has practical implications for the maintenance of medicinal flies and production of medicinal maggots: firstly, a protein-rich diet has to be offered to adult flies as soon as they emerge; secondly, colonies produce reasonable numbers of eggs around two weeks after emergence (personal experience with L. sericata); and thirdly, harvesting of eggs from the same flies may be repeated every three days, or twice per week.
Blowflies, and in particular L. sericata and L. cuprina, have a diurnal activity pattern which means that in the laboratory egg harvesting should be undertaken during daylight hours [31]. Egg laying has been observed between 17.5℃ and 40℃ [32] but this range is of little relevance to laboratory rearing of medicinal flies because it is necessary to maintain optimal and predictable rearing conditions. For this reason, the temperature in the insectary should be kept at a constant temperature between 25℃ and 27℃.
The most common method for the harvesting of eggs is to place non-sterile decomposing liver in the adult fly cage [7], but this can vary between species with Chrysomya species preferring minced and wool-covered meat (personal communication). Blowflies like to deposit their eggs on animal carcasses in dark, protected places. In order to simulate the carcass environment and to stimulate egg laying in the laboratory, this behaviour can be encouraged by placing an opaque container with sufficiently large holes on top of the protein bait. The females promptly climb in and lay eggs. Once the first egg masses have been deposited on or near the bait, other females are encouraged to follow suit [7, 33]. Harvesting is best done first thing in the morning to ensure there is sufficient time in the day to disinfect the eggs and place them on sterile media if they are destined for maggot therapy.
Egg development has been studied for a variety of calliphorid fly species as part of general developmental studies. Investigations with L. cuprina and L. sericata have shown that optimal embryo development after oviposition and subsequent successful eclosion (hatching) of eggs needs to occur in a moist environment with a relative humidity in excess of 80%, and that relative humidity below 50% results in no eggs hatching [34, 35]. If eggs should accidentally dehydrate in the laboratory, their hatching rate will greatly improve if they are soaked in water for a minute or kept for an hour or so in a container lined with wet tissue paper, creating a high-humidity environment. In L. sericata, as in other calliphorid flies, higher temperatures lead to faster egg development post oviposition and earlier hatching. For L. sericata at 25℃ to 27℃ hatching takes place after approximately 18 hours from oviposition [7]. In nature on a living sheep, the wound temperature does not drop below 31℃, and at such temperatures over 30℃, egg development is greatly accelerated, taking only 12 hours or less. To conclude, for rearing of medicinal flies in the laboratory it is critical that egg harvest is a speedy process so that eggs are not allowed to dehydrate. After harvest, eggs should be kept close to 100% relative humidity or at the very least on a moist substrate. In addition, the temperature at which eggs are incubated may be varied to speed up or slow down egg development to suit laboratory work processes. Low temperatures around 5 or 6℃ can be utilised to store eggs for two to three days, with up to 50% mortality to be expected at 5℃ [34, 36].
How to Care for Maggots
Rearing Cage Setup
The captive rearing setup for blowfly larvae has to facilitate their rapid growth and feeding over a period of three to five days, and subsequent pupariation. For the latter, post-feeding maggots in the wild wander off the carcass and burrow into soil or leaf litter [37]. To facilitate this process in the insectary, the most convenient method is to house maggots in a double container system where a smaller, open-topped container is placed into a larger container which is covered tightly with a fine-weave fabric mesh. The smaller container holds the diet and feeding larvae whilst the larger container is partially filled with a pupariation medium that can be wheaten chaff, sawdust, sand, or vermiculite (Figure 14.3). Sawdust and vermiculite are inhalation irritants and should be avoided if other substrates are available. Sand is a convenient substrate because it facilitates the separation and collection of pupae with a sieve. It has the added advantage that it can be periodically washed, dried, and heat-sterilised, although it might be more convenient to discard the sand after several maggot-rearing cycles. The use of cat litter made from extruded recycled paper (e.g. Breeders Choice, FibreCycle™, https://fibrecycle.com/breederschoice) has also proven to be a convenient, clean, and largely dust-free alternative (Figure 14.4). It is highly absorbent, which maintains a dry pupariation environment. Like sand, it also facilitates easy separation of the pupae from the substrate because the extruded, sausage-like litter particles are much larger than the pupae.
Larvae will feed on the diet inside the diet container until they are fully-grown or the food is exhausted. Upon entering the post-feeding stage, the maggots leave the food and wander up the side of the diet container and drop onto the pupariation substrate into which they bury themselves ahead of pupariation.
Blowfly maggots have a scrambling, exploitative feeding strategy that sees them compete intensely for food resources [38]. Therefore, food limitation is an undesirable consequence of overstocking and underfeeding and must be avoided. Should larvae be starved of food, they will enter the post-feeding stage and pupate prematurely, which leads to smaller adult flies with reduced fitness that produce fewer offspring [6, 23]. In medicinal fly rearing, laboratory standard operating procedures need to establish procedures for larval rearing regarding the amount of larval diet and stocking density. Irrespective of such protocols, insectary workers must regularly monitor larval development to spot food shortages early enough before they lead to post-feeding behaviour.
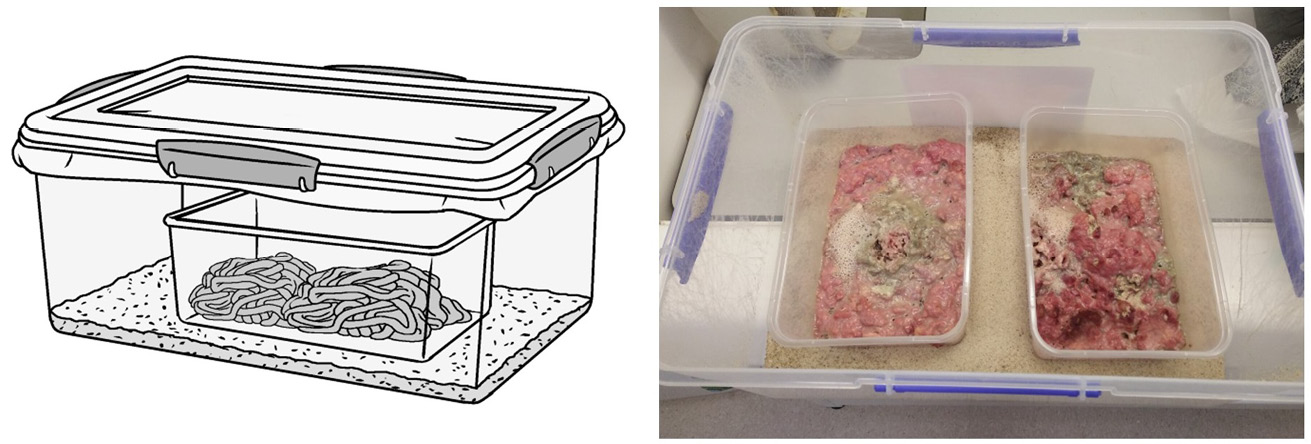
Figure 14.3 Setup for the rearing of maggots. A) and B) Meat-filled smaller containers are placed into a larger container that has been partially filled with sand or another medium for maggots to pupariate in when leaving the diet. A) The outer container is securely closed with a tight-fitting but well-ventilated lid. A large hole cut into the lid with a fine-weave fabric sandwiched between lid and outer container is an easy way to provide such ventilation. Illustration by P. Busana and photo by F. Stadler, MedMagLabs and Creating Hope in Conflict: A Humanitarian Grand Challenge, CC BY-ND.
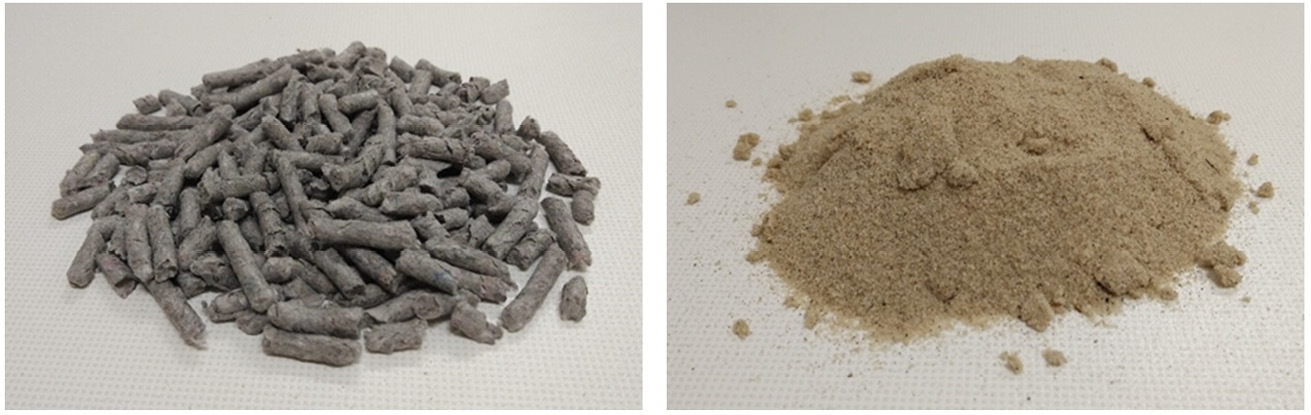
Figure 14.4 Suitable pupariation substrates. A) Extruded cat litter pellets made from recycled paper. B) Fine sand. Both substrates make separation of pupae with a sieve or strainer easy. Mesh or hole size of the sieve/strainer may be chosen to either capture pupae when sand is used, or the cat litter pellets which are larger than pupae. The same basic principle applies to other substrates as well. Photos by F. Stadler, MedMagLabs and Creating Hope in Conflict: A Humanitarian Grand Challenge, CC BY-ND.
Types of Maggot Diet
Many researchers advocate the use of liver as a suitable larval food. However, the evidence from feeding experiments shows that ovine, bovine or porcine liver are nutritionally inferior compared with various other tissues such as heart, lung, brain and meat which result in better larval growth performance [12] [10]. There are many reasons why it might not be feasible to use heart, lung or brain for maggot rearing. In this case commercially available liver or minced meat from a range of species including cattle, pig, and poultry will suffice. Once again, it is important to be consistent with the nature and quality of the larval food in order to be able to predict consumption and development rates. Interestingly in forensic entomology, a feeding study with Calliphora vicina found no developmental differences between pork and human tissue [39]. This raises the question of whether medicinal flies might be best reared on pork to breed fly strains that are better adapted for human maggot therapy.
Artificial diets for the rearing of blowflies have been developed over the years, not least because there was the need to reduce the odour associated with larvae rearing on putrid raw meat [7, 40]. Moreover, the plethora of diets used in forensic and medical entomology make it difficult to compare results between studies [41]. More recently, research investigating the antimicrobial activity of larval excretions and secretions has led to the development of various agar-based artificial diets [11]. However, the use of sterilisable semisynthetic diets for the aseptic rearing of medicinal maggots can be traced back to William S. Baer, who pioneered maggot therapy in the 1930s and ’40s [42]. Semi-synthetic sterilised diets for fly larvae contain animal protein, various other nutritional ingredients and an agar base [40, 41]. The diet can be stored at room temperature and it generates a less bad odour when rearing maggots.
Rearing of large numbers of flies for maggot therapy and other purposes, including pollination services, may turn out to be rather expensive, or impractical if ingredients are unavailable in resource-constrained settings. An alternative diet was developed for Lucilia caesar in West Africa, using locally available ingredients such as garri (processed cassava root, Manihot esculenta), soybeans, palm wine and liver [43]. In India a cheap diet for the rearing of Chrysomya megacephala was developed using soya flour, milk powder, and egg [44].
Completely meat-free diets have also been developed for L. sericata containing whole milk powder, dried yeast, wheat germ, agar powder, propionic acid, and distilled water (Table 14.3). Apart from a slightly longer larval development time, there appears to be no difference in mortality or pupal weight and pupal development compared with that of a liver diet [4]. Producers are encouraged to experiment with locally available and affordable resources to develop convenient diets that meet the nutritional needs of the medicinal fly species chosen. Synthetic or semi-synthetic diet performance for each fly species and strain should be tested against a known, reliable meat diet such as minced pork meat [12].
The therapeutic use of living organisms poses cultural, religious, and clinical challenges. To make the treatment acceptable to the widest possible patient cohort, it is critical that cultural and faith-appropriate medicinal maggots are available. For example, Malaysian biotech firms consider their halal-appropriate technologies as a market differentiator [45]. Allergic reactions to flies themselves, as well as to the dietary ingredients on which the flies have been reared, may occur [46]. In an ideal world, producers would offer allergen-free medicinal maggots on demand. It is also advisable that producers maintain excellent customer relationship management systems and stay in close contact with medical practitioners, to obtain feedback on medicinal maggot quality, performance and adverse reactions. Should clusters or patterns of complications such as allergic reactions be identified, then alternative rearing methods may be used, and quality control and quality assurance improvements made. The various diets already developed and the tolerance of medicinal flies to a range of rearing conditions make such customisation possible if it is required.
Table 14.3 Synthetic maggot diet [4].
Meat-free maggot diet with whole milk powder |
50g whole milk powder 50g dried yeast/brewer’s yeast 50g wheat germ 14g agar powder 5mL propionic acid 1000 mL water |
Note: All ingredients are mixed and boiled for ten minutes. Propionic acid is added once the diet has cooled but not set. To facilitate rapid cooling and convenient handling, the diet is best divided up into portions stored in single- or multi-use plastic containers. To prevent spoilage, diets are stored in the refrigerator.
Pupariation
As with all life stages, pupariation is dependent on adequate ambient temperature. L. sericata require temperatures above 20℃ for consistently high pupariation rates [35]. Once most larvae have left the food and have pupated in the pupariation substrate, the pupae are collected by sieving. The pupae are then stored within the temperature-controlled insectary environment until adult eclosion. If necessary, pupae can be refrigerated at 4℃ for some time in order to delay development [47].
Dehydration is a concern during pupal development, particularly where the ambient relative humidity is difficult to control. Studies have shown that L. sericata pupae contain about 70% water and tolerate up to 28% dehydration [48]. Emergence of flies is also determined by the temperature during development, and it increases to approximately 80% for a temperature range between 20℃ and 30℃. Extremely high temperatures above 35℃ are lethal for pupae who would naturally reside in temperature-buffered soil for the period of pupal development [35].
Pupae are a convenient life stage for shipment between laboratories. L. sericata pupae require 0.2 mL of air per day per 1 mg of pupae, which needs to be provided either via ventilation or provision of sufficient air volume in sealed containers [49].
Disinfection of Medicinal Maggots
Adult flies and maggots inhabit many unsanitary environments and utilise microbe-rich food sources. Therefore, blowflies can harbour a range of harmful microbes, such as Staphylococcus aureus, Escherichia coli, Corynebacteria dipththeria, Aspergillus and Fusarium, which they potentially introduce to wounds during myiasis [50]. Consequently, there is a need to ensure that fly larvae used for medicinal wound treatment are sterile. However, it must be noted that anecdotes and academic case reports of myiasis [51, 52] point toward highly effective microbial control under most austere and unhygienic circumstances. Nevertheless, it is necessary to minimise the microbial burden of medicinal maggots to avoid the introduction of harmful microbes that can exacerbate already-existing infections or even lead to bloodstream infection [53].
There is a growing realisation among the maggot therapy research community that completely sterile maggots are unachievable and perhaps undesirable from a therapeutic efficacy point of view [54–56]. There is good evidence to suggest that bacteria are internally transferred from adult fly to egg, from egg to larva, from one larval stage to another [55]. This means that currently practiced ‘sterilisation’ methods for medicinal maggots achieve at best surface sterilisation [57]. In other words, recommended rapid sterility testing over 24 to 48 hours for disinfected eggs and emerging maggots has its limits because some microbes may be difficult to culture and/or hide in internal maggot tissues and organs, and thus remain undetected. The exact relationship and ecology of the microbes inside and on the body of medicinal fly species and their environment is still little understood and requires further investigation. However, what current evidence and centuries-long practice of maggot therapy in tribal and modern wound care can tell us is that medicinal maggot species effectively reduce the microbial burden in their wound environment and control microbes that impair wound healing [58]. This should also include the microbes introduced to the wound by medicinal maggots unless they are co-adapted symbionts [55], which are likely to enhance the therapeutic activity of maggots. Beneficial cases of myiasis provide further support for this hypothesis [42, 51, 52].
In summary, current practice of disinfecting the eggs and subsequent aseptic incubation of emerging maggots [59] has proven to be safe for both maggots and patients. The maggots remain therapeutically efficacious and do not cause or exacerbate wound infections.
Disinfection Methods
The most convenient and most common way to produce largely germ-free maggots involves the disinfection of the fly eggs and subsequent rearing of larvae on sterile media. Conversely, some workers advocate the disinfection of only the larvae [60], or a two-stage process involving sterilisation of both the eggs and young larvae [61]. A range of successful sterilisation procedures have been reported in the literature.
Because eggs in an egg mass are glued together as they are deposited by the female, the eggs must be first mechanically separated from each other in order to permit total surface disinfection. The importance of this step cannot be overemphasised if complete surface disinfection is to be achieved. The deglutination process can be enhanced with chemical reagents such as sodium hydroxide, potassium hydroxide, or sodium carbonate [62]. Sterilisation protocols vary regarding the chemicals used but also regarding the efficacy of the disinfection process and the egg survival rate [59]. The protocol described in Box 14.1 is a synthesis of protocols reported in the literature. They describe the same general process which includes separation and prewash of eggs, followed by the actual disinfection step. After each treatment, eggs must be washed or rinsed to remove treatment chemicals, liberated debris, and microbes. Although this generic method is based on reliable disinfection protocols published in the literature, it is recommended that producers try a few and finetune their own protocol. A summary of common disinfection methods and their efficacy is given by Brundage and colleagues [59]. Finally, it is important to use generous amounts of solution for the deglutination, disinfection, and rinsing steps because the chemicals in solution lose potency proportional to the number of eggs and amount of contamination present, and because the greater the dilution of contaminants and chemicals, the more effective the cleansing process.
In line with Good Manufacturing Practices, the entire sterilisation process ought to be conducted in a Grade A zone clean room [63]. Instead of an entire room being maintained as a Grade A zone, the same microbial protection can be achieved for much cheaper with a laminar flow cabinet that prevents contamination of eggs and larvae from germs suspended in the laboratory air. Each production batch of sterilised eggs and emerging sterile larvae must be tested for sterility before it can be released for maggot therapy.
Box 14.1 Synthesised protocol for the sterilisation of medicinal fly eggs.
Incubation of Eggs
For the incubation of eggs and rearing of larvae to late-first or early-second instar, the sterilised eggs are placed on a sterile larval diet which may be chicken egg-yolk, a semi-synthetic diet, or a fully artificial larval diet [4, 7, 11, 13, 40, 65]. For instance, a basic semi-synthetic diet consisting of 20 g/L agar, 20% horse blood, and added yeast supports L. sericata larval growth comparable to that of a meat diet [11, 13]. Chocolate agar, sabouraud dextrose agar, and brucella blood agar also provide adequate nutrition to hatching maggots.
At 25–27℃ eggs hatch within 18 hours and reach the second-instar life stage within 24–48 hours of egg incubation. Therefore, rearing at 25–27℃ allows for harmonisation of production timing with quality control testing. If need be, first- and second-instar larval development can be paused with refrigeration at 6–8℃ to await test results or in anticipation of maggot therapy [66]. Temperatures below 6℃ may cause unsustainable mortality of fragile first- and early second-stage maggots.
Quality Control
Testing of disinfection efficacy. Microbial quality control may occur at all stages of the disinfection and rearing process for maggots destined for maggot therapy to minimise the microbes carried by medicinal maggots and to avoid harmful microbial contamination. In line with aseptic laboratory practice, all disinfection processes are undertaken in the laboratory inside a laminar flow cabinet or clean room with sterile reusable or disposable laboratory equipment and consumables in order to minimize contamination of solutions, media, and samples via the laboratory air [63]. In addition, it is good practice to also monitor the laboratory environment, especially the clean room facilities, on a regular basis for microbial contamination [67].
Disinfection is tested at the very least directly after the disinfection of eggs and ideally again after the eclosion of maggots. Because medicinal maggots are highly perishable, and cannot be stored over extended periods, there is insufficient time to conduct sterility testing as advised by the Pharmacopoeia [68] and expected in medical goods manufacturing [69]. Therefore, microbial testing may be done using blood agar (trypticase soy agar) plates in an incubator at 35℃ for 24–48 hours [7, 70]. Alternatively, the sterility testing methodology specified in the Pharmacopoeia and related guidelines may be employed but test samples are assessed after 24 and 48 hours to facilitate rapid dispatch of medicinal maggots. Personal experience suggests that if culturable microbes have been sampled, they proliferate rapidly and are readily detectable after 24 to 48 hours. The precise quality assurance and quality control procedures for medicinal maggot production demanded by the regulators may vary from jurisdiction to jurisdiction as will the regulators’ general approach to maggot therapy [71].
Colony and medicinal maggot performance monitoring. Blowflies in general, and L. sericata and L. cuprina in particular, have proven to be well-suited for laboratory maintenance as they tolerate a wide variety of diets and rearing conditions. However, declines in laboratory fly strains kept at constant conditions have been reported, and have manifested in reduced fecundity, cessation of egg production and abnormal pupation [72]. This is not acceptable in a production context where reliable and consistent colony performance is required. Therefore, there is a need to monitor life history parameters on a regular basis. Species-specific characteristics such as morphological and life-history, physiological, and genetic characteristics can all be used to monitor colony performance [73, 74]. The sampling regimen and parameters to obtain these performance measurements must be carefully considered but may include data on adult fly performance, egg development, larval development, and pupal development.
The capacity of medicinal maggots to debride necrotic tissue may also differ from fly strain to fly strain or over time. The debridement activity of medicinal maggots can be assessed clinically by monitoring their therapeutic action in the wound or in the laboratory with a simple meat-based assay [75–77]. Recently, a radial diffusion enzymatic assay (RDEA) has been developed in order to obtain more reliable performance data in medicinal maggot production and to support good manufacturing and laboratory practice [78].
Supply Management and Perishability of Medicinal Maggots
It would be desirable to store eggs, pupae, or adult flies over extended periods of time. This would support supply and demand management of medicinal maggots, particularly regarding regular demand fluctuations and rapid demand spikes in the aftermath of, for example, an earthquake which would bring about many injuries and related wound infections that can be treated with maggot therapy [79]. Unfortunately, longer-term cold storage or cryopreservation of eggs, maggots, or flies is not yet available. Indeed, medicinal maggots are a highly perishable commodity in terms of their vulnerability to adverse environmental conditions such as hot temperatures, very cold temperatures, or low humidity. In addition, medicinal maggots grow rapidly and have corresponding energetic requirements. Provided with enough food, the development rate of medicinal maggots is largely determined by the temperature. This has far-reaching implications for production scheduling, supply management, distribution, and clinical practice. In the context of supply-chain management, medicinal maggots can be considered perishable [80] because:
- if environmental conditions are not conducive, or if nutrition is withheld from the maggots for too long, they will lose vitality and efficacy and may even die;
- the longer medicinal maggots are held in cold storage and transit, the less time is available to the healthcare provider to arrange for treatment in the clinical setting which reduces convenience and thus value of medicinal maggots as a commodity;
- to safeguard against the use of lower-quality medicinal maggots and associated reputational damage, and also to assist in treatment scheduling, producers set a safe expiry or best-before-date which also influences perishability and its implications for supply chain management.
In the absence of long-term egg storage technology and fluctuating demand, the response time for a single facility to increase production is in excess of 20 days, unless the facility maintains production capacity buffer in the form of surplus fly stock of all development stages. This incurs additional costs in feed and maintenance which under a lean production strategy would have to be avoided. It is then a matter for the producer to reconcile the benefits of responsiveness and agility with the additional costs incurred by the maintenance of surplus inventory in the form of fly stock. In other words, a more suitable supply chain model would be ‘agile’ rather than ‘lean’.
Seamless delivery of medicinal maggots to healthcare providers requires careful management of the disinfection and quality control process. Hence these disinfection and quality control procedures potentially form a bottleneck in the production of sterile maggots. If sterility testing with agar plates is to be performed at the time of egg disinfection and again when maggots hatch, then sterile maggot production (from egg harvest to shipment of maggots) takes two to three days. Given that larval growth is rapid and full larval development can take less than 2 days under optimal conditions, there may be a need to refrigerate first- or second-instar larvae at 6–8℃ in order to arrest their development until sterility is confirmed. Likewise, maggots are shipped overnight at between 6℃ and 25℃ to healthcare providers and may be stored within this temperature range for up to 24 hours at the point of care [66, 77, 81].
There may be instances where delivery times are expected to exceed 48 hours or there is reason to believe that treatment cannot proceed within 48 hours from dispatch of maggots from the lab, even if delivered in time. If this is the case and there is a strong argument in favour of maggot therapy treatment, then producers can send disinfected eggs rather than first- or second-instar maggots. For this purpose, disinfected eggs may be placed on a sterile, moist gauze pad for shipment. This buys some time, especially when shipped with extra supply of cool elements to prolong the cool period, but the eggs will hatch eventually. The emerging maggots require food immediately and there is a risk that they will perish if not applied to the wound shortly thereafter. To extend the safe shipment and storage duration even further, it will be necessary to provide the first-instar maggots with a sterile protein food source. This combination of egg shipment, longer cool conditions during transit, and the provision of food for emerging maggots has been shown to extend the shelf-life of medicinal maggots to five days (personal communication). One drawback of this shipment method is that both the chorion (‘egg shell’) from which maggots emerge and any uneaten food will be applied to the wound along with the medicinal maggots. Although there is no evidence of adverse treatment outcomes when disinfected eggs have been applied directly [82], healthcare providers may nevertheless be concerned. Moreover, producers must be careful to operate within the licence conditions for the production and supply of medicinal maggots as negotiated with their ministry of health or other regulator. It appears that the extension of medicinal maggot shelf-life in this manner, although practiced from time to time (personal communication), has not yet received any formal attention in the scientific literature. For a detailed discussion of the distribution logistics for medicinal maggots please consult Chapter 17 of this book [83].
Scheduling of Production Activities
Scheduling production activities to ensure reliable supply from week to week and throughout the year is no trivial undertaking. However, with available information from the literature and existing producers, it is possible to draw up a weekly production schedule [84]. The schedule in Table 14.4A considers a 7-day production week and assumes that courier and distribution services are available on the weekend. In this case medicinal maggots can be delivered daily for application on any day of the week. However, adherence to a Monday-to-Friday working week as in Table 14.4B will interrupt production and dispatch. Consequently, treatment will not be possible on Mondays. In practice, variations to these schedules are likely depending on local circumstances [84].
Table 14.4 Sterile maggot production schedules. The theoretical schedule for a 7-day production operation with harvesting of eggs, quality control, and shipment taking place every day of the week is shown in Table 14.4A. Importantly, this schedule allows for maggot therapy every day of the week. In contrast, the schedule in Figure 14.4B shows the impact of weekend-interruptions (Saturday and Sunday) to production and distribution. As a consequence, maggot therapy treatment may not be possible on Sundays if couriers don’t operate on the weekend, and will not be possible on Mondays. These are only example schedules and actual schedules will vary from producer to producer depending on local circumstances, operating procedures, and demand fluctuations.
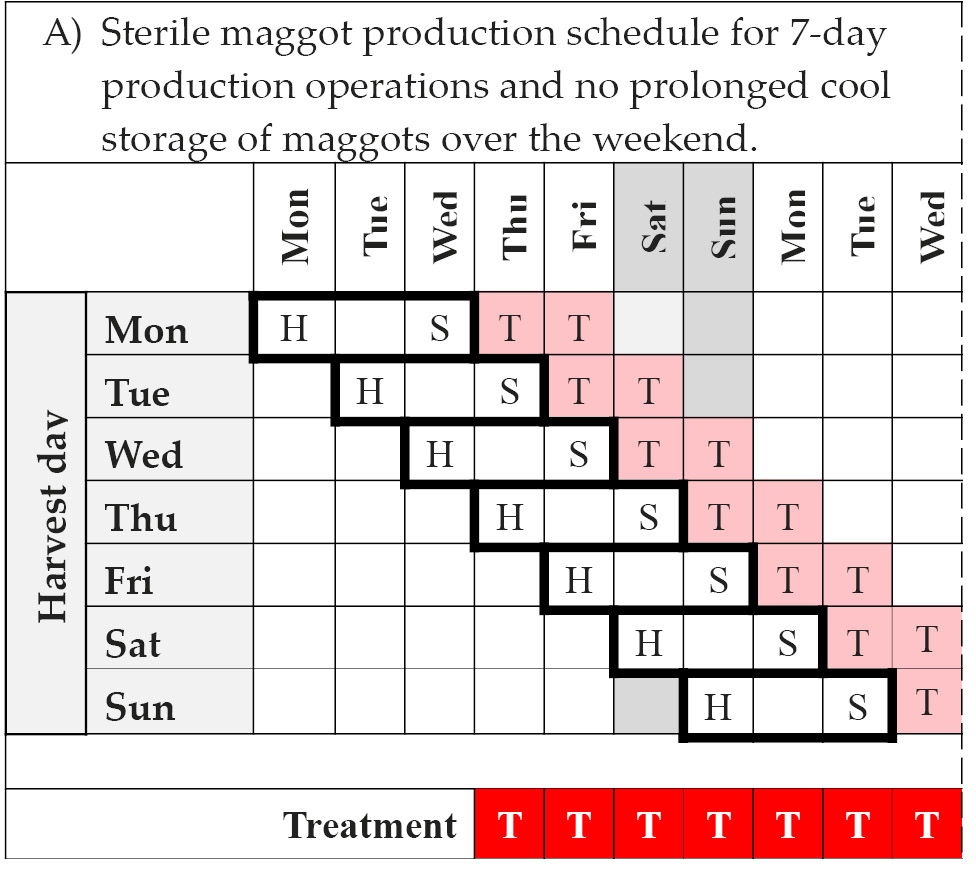
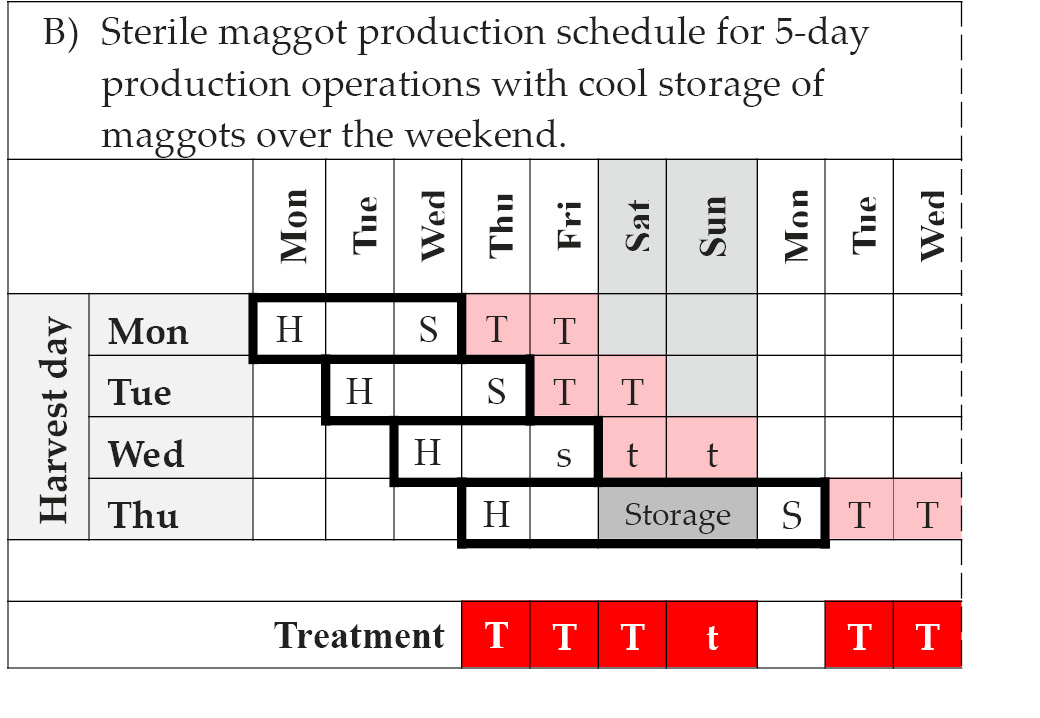
Note: H = harvest of eggs and disinfection; S = quality control clearance and shipment; T = treatment; Storage = cool storage of maggots in-house; s = potential shipment and t = potential treatment, provided courier service is available for delivery on Saturday and care provider / patient is willing to pay surcharge. © F. Stadler, https://doi.org/10.25904/1912/3170.
Quality Management
Laboratory operation. Of the many ISO standards relevant to medicines and medical devices, ISO 9001:2015 Quality management systems, and ISO 15189:2012 Medical laboratories—Requirements for quality and competence are of particular importance. ISO 9001 specifies requirements for consistent delivery of products and services according to statutory and regulatory requirements. Many pre-existing organisations operating laboratories such as clinics, pathology, and research laboratories, may well comply with ISO 9001 and ISO 17025:2005 General requirements for the competence of testing and calibration laboratories but may also need, depending on the maggot therapy approval process, to consider accreditation for ISO 15189. Moreover, if medicinal maggots are considered a medical device by the respective regulator, then ISO 9001 may need to be replaced with a more comprehensive quality assurance standard. For example, Malaysian medical device manufacturers had successfully adopted ISO 9001:2000 but were compelled to transition to ISO 13485:2003 Medical devices—Quality management systems—Requirements for regulatory purposes [85] in order to access the harmonised export markets of Europe and elsewhere [86]. Since producers mostly supply medicinal maggots to national or regional healthcare providers (e.g. within the EU), such regulatory pressure may not apply in all cases, but it illustrates the complexity of the regulatory landscape for producers. Moreover, regulatory compliance can be a large burden to a producer, both in terms of time commitment and cost, and full accreditation may not be affordable to many laboratories in low- and middle-income countries [84]
Barriers aside, there are clear benefits to accreditation and adherence to standards. For example, ISO 9001 enforces consistency and efficiency of processes which increases quality and reduces errors. Customers and international regulators look to ISO 9001 as a quality assurance for supplied products, and accreditation can convey competitive advantage. Implementation of ISO 9001 will also assist in solving and avoiding problems in the manufacturing process and will lead to leaner, more efficient companies [87].
Occupational Health and Safety
The health and wellbeing of insectary and laboratory workers throughout the rearing, quality control and packaging process is of utmost importance. Occupational health and safety (OH&S) considerations specific to the rearing of medicinal flies include the potential of allergies and/or fly-borne diseases. If air purification systems cannot be fitted in the insectary due to practical or financial constraints, then dust allergens from adult fly colonies can be managed by frequent wet wiping of insectary surfaces and by suspending fly cages over trays of water to catch the fly-generated dust.
Adult wild calliphorid flies are potential animal- and human-disease vectors and may deliver pathogens via their body surface or excretions, including anthrax, Clostridium botulinum, and the causal agents for paratuberculosis and avian influenza [88–90]. Long-term culturing of flies in captivity should, over time, lead to less harmful bacterial burdens, particularly when synthetic and semi-synthetic diets are used in the rearing of larvae. Nevertheless, it is important to minimise the potential for transmission of fly-borne diseases with good insectary and laboratory hygiene, protective clothing and best-practice hand-hygiene. This goes hand-in-hand with dust control as insectary shelving, benchtops and floors should be wet-wiped with disinfectant surface cleaner (e.g. domestic bleach) several times per week. Production workers should use two sets of protective clothing, one dedicated to the insectary and the other to lab work. This is to avoid cross-contamination between the two production environments. Protective clothing includes hair caps, face masks, and gloves in order to reduce dust and germ exposure in the insectary and to minimise the potential for contamination of medicinal maggots during the sterilisation process.
Sustainable Production and Waste Management
From a medical and therapeutic point of view, maggot therapy is highly sustainable because it is a low-tech therapy that is effective under the most austere conditions and it significantly reduces the need for antibiotic treatment of wound infections. Thus, it does not contribute to the growing problem of antibiotic resistance in human pathogens. Indeed, maggot therapy effectively controls antibiotic-resistant bacteria [91].
As far as the sustainability of medicinal maggot production itself is concerned, it depends among other things on producer location, available resources and infrastructure, and general awareness. It cannot be assumed that producers globally have guaranteed access to clean water, reliable energy, ready supply of equipment and consumables, and a reliable municipal sewage and waste service, especially in the case of impoverished and remote locations. Out of necessity, this provides an opportunity to implement sustainable water and energy solutions. Paradoxically it may be harder for producers in high-resources settings to become more sustainable. For example, the reuse of materials, as opposed to the use of disposable items, is associated with more time-consuming cleaning and maintenance which results in higher labour costs, especially in high-income, high-resource economies. Therefore, a producer must carefully weigh up whether it is of benefit financially, operationally, and safety-wise to re-use materials. Indeed, the additional labour, electricity, water, and reagent inputs required for on-site cleaning and disinfection may be more costly and environmentally unsustainable than opting for disposable materials. For commercial producers, increased costs associated with sustainable production may either reduce the profit margin or will be passed on to the consumer through higher product prices. However, for producers aiming to supply cheap and affordable medicinal maggots to the poor and underserved, any production cost increase is difficult to pass on and may undermine their business model and viability.
Fortunately, medicinal maggot production is not very resource intensive compared with other industries. Setting up a production facility in a building with high environmental performance (insulation, air conditioning, lighting, plumbing, etc.) immediately increases operational sustainability. Where possible (or necessary), solar energy generation may be utilised to meet all or at least some of the production requirements, and to reduce reliance on the grid or generator power.
Waste management is an important component of sustainability. Neither liquid nor solid waste streams in production can be classified as containing clinical infectious waste since no clinical treatment is being performed and no patient-generated waste is being disposed. However, leftover putrid meat and other fly-diet waste is undoubtedly laden with pathogens and must therefore be disposed of in a safe manner according to local regulations. In practice, production waste does not differ significantly from municipal waste. Odour is of course a concern when collecting insectary waste prior to disposal, but it can be minimised by storing putrid meat waste in a dedicated freezer or cool room until the day of collection by waste services. The added benefit of this is that any eggs, maggots or flies among the waste are euthanised prior to disposal. For peace of mind, producers may want to adhere to the World Health Organisation’s Safe Management of Wastes from Health-care Activities guidelines [92] and of course any local laboratory management guidelines where they exist.
In the insectary, most materials can be re-used after thorough cleaning including the maggot-rearing containers, the adult diet-dispensing dishes and containers, as well as the fly cages themselves. This is also true for the laboratory materials and equipment. However, as mentioned earlier, producers should carefully evaluate whether re-use of lab materials makes financial sense and is indeed more sustainable. When it comes to disposable personal protective gear such as gloves, gowns and face masks, there is room to be less wasteful. Here, waste is mostly due to the behaviour of lab and insectary workers. For example, thorough planning and streamlining of activities will reduce the need for frequent removal and disposal of gloves. The same face mask may be re-used several times throughout a day if convenient and safe to do so. Replacing single-use gloves with multi-use heavy duty gloves for some insectary work such as cleaning activities will also reduce waste.
The greywater waste generated during regular insectary and laboratory operations does not require special segregation or treatment. It can be disposed of in municipal sewage and stand-alone septic systems, provided chemicals such as from egg disinfection and lab cleaning activities are heavily diluted during disposal. Please note that irrespective of this advice, it is the responsibility of producers to comply with local rules and regulations.
Medicinal Maggot Production in Healthcare Settings with Poor Medical Services
The approaches and methods for medicinal maggot production described in this chapter assume that the materials and resources such as laboratory equipment, cages, and rearing diets can be readily purchased from suppliers either in shops or online, and that they are delivered in a timely fashion. It has also been assumed that the producers of medicinal maggots and their staff have qualifications in entomology and laboratory sciences. Unfortunately, this is not the case everywhere and the impacts of war, disasters, and deep economic depression create conditions in many parts of the world that result in the collapse of ordinary trade and commerce, broken supply chains, and the departure of scientists and other educated workers seeking opportunities elsewhere. Even emergency and development aid from governments and non-governmental organisations may fail to reach affected communities. If isolated communities want to take advantage of highly efficacious maggot therapy to treat patients, they must produce the medicinal maggots themselves. Highly perishable as they are, a reliable supply of medicinal maggots can only be guaranteed with local production.
To that end, MedMagLabs (www.medmaglabs.com) has developed instructions that allow communities in compromised healthcare settings to establish and operate do-it-yourself laboratories (DIY-Labs) and produce safe medicinal maggots. The guidance includes a Production Manual that explains the production process in some detail, and a User Manual that mirrors the content of the Production Manual (Figure 14.5). However, the difference is that the User Manual and its 20 fact sheets provide step-by-step descriptions of the production processes with clear illustrations in order to make the information more accessible.
The DIY-Lab is not a radical reimagining of medicinal maggot production. Instead, the MedMagLabs team have translated best-practice know-how as reported in the scientific literature into methods that can be implemented with very few locally available resources and without prior knowledge of fly husbandry. First, assumptions regarding the availability of resources that can be appropriated for medicinal maggot production were triangulated with five interviews of people who have lived or worked in conflict-affected communities. Then, a set of basic visual and textual instructions were prepared for lay producers. Without access to communities in conflict zones, MedMagLabs sought proxy communities to collaborate with. Four teams of citizen scientists (Year 9 and 10 high-school students) helped to establish whether lay persons can produce medicinal maggots and what guidance is required [93]. The students built adult fly cages, cared for fly colonies, reared maggots, disinfected eggs, and incubated young medicinal maggots with only basic resources such as repurposed water drums or jam jars. The outcomes of this collaboration had a material influence on what guidance was included in the Production and User Manuals, and how instructions were presented.
Both the Production and User Manual are creative commons (CC BY-ND) and can be accessed for free via www.medmaglabs.com along with a Treatment Manual for healthcare providers and lay carers (Figure 14.5).
.png)
.png)
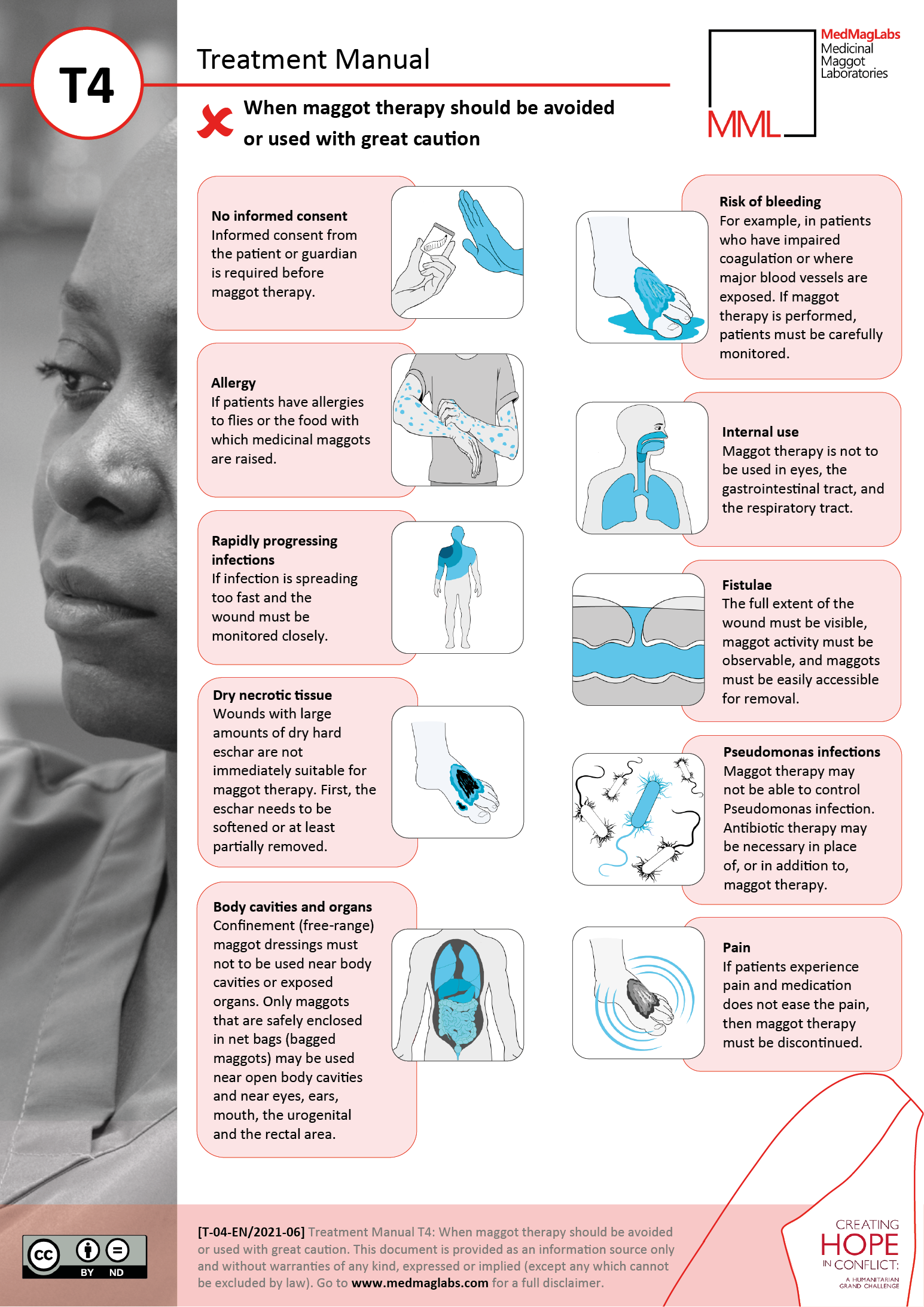
Figure 14.5 Example pages from the MedMagLabs medicinal maggot Production Manual (b5), the corresponding highly-visual User Manual (B5), and the Treatment Manual (T4). These resources were developed to empower isolated, conflict-affected communities to produce medicinal maggots and perform maggot therapy with local resources and expertise.
Summary
This chapter provides a detailed description of both insectary and laboratory activities for the maintenance of fly colonies and the production of medicinal maggots. It describes how the care of adult flies and maggots depends on caging, environmental conditions, and diet. Of critical importance to the reliable and responsive production of high-quality, safe maggots for maggot therapy is the thorough knowledge of the reproductive and developmental performance of medicinal flies. Producers must have a reliable quality-management system in place and implement routine quality control not only to ensure the sterility of medicinal maggots destined for treatment but also for colony performance overall. Paramount to any workplace is the health and wellbeing of its workers. Insectaries provide special challenges, particularly regarding allergies and respiratory health, that need to be carefully managed. It is the objective of the producer as the focal organisation in the supply chain to ensure supply security and responsiveness to demand fluctuations which requires scheduling of production activities. Unfortunately, there is a tradeoff between responsiveness and lean supply management. In other words, the limited availability of cool or cold storage of eggs or other life stages makes lean and responsive supply management difficult to achieve. Moreover, it is incumbent on producers to limit their environmental footprint in terms of resource consumption and waste. This may be associated with savings and increased operational resilience but may also incur greater costs, which would need to be passed on to patients or absorbed by the producer.
Finally, the guidance provided here is based on available production information from the scientific literature and producers. Adherence is not mandatory, and it is indeed expected that producers will experiment and tailor methods to their own needs and local circumstances. Communities in compromised healthcare settings are no longer excluded. Free Production and User Manuals are available to empower lay persons to produce safe medicinal maggots with local resources. However, this does not mean that producers have complete freedom. There are rules and regulations that apply to and govern the production of medicines and medical goods in all but the most austere and isolated healthcare settings.
References
1. Stadler, F., Laboratory and Insectary Infrastructure and Equipment, in A Complete Guide to Maggot Therapy: Clinical Practice, Therapeutic Principles, Production, Distribution, and Ethics, F. Stadler (ed.). 2022, Cambridge: Open Book Publishers, pp. 237–256, https://doi.org/10.11647/OBP.0300.12.
2. Stadler, F., et al., Fly Colony Establishment, in A Complete Guide to Maggot Therapy: Clinical Practice, Therapeutic Principles, Production, Distribution, and Ethics, F. Stadler (ed.). 2022, Cambridge: Open Book Publishers, pp. 257–388 (p. 269), https://doi.org/10.11647/OBP.0300.13.
3. Stadler, F., The Ethics of Maggot Therapy, in A Complete Guide to Maggot Therapy: Clinical Practice, Therapeutic Principles, Production, Distribution, and Ethics, F. Stadler (ed.). 2022, Cambridge: Open Book Publishers, pp. 405–430, https://doi.org/10.11647/OBP.0300.19.
4. Tachibana, S.I. and H. Numata, An Artificial Diet for Blow Fly Larvae, Lucilia sericata (Meigen) (Diptera: Calliphoridae). Applied Entomology and Zoology, 2001. 36(4): pp. 521–523.
5. Bugelli, V., et al., Effects of Different Storage and Measuring Methods on Larval Length Values for the Blow Flies (Diptera: Calliphoridae) Lucilia sericata and Calliphora vicina. Science and Justice, 2017. 57(3): pp. 159–164, https://doi.org/10.1016/j.scijus.2016.10.008.
6. Moe, S.J., N.C. Stenseth, and R.H. Smith, Density Dependence in Blowfly Populations: Experimental Evaluation of Non-parametric Time-series Modelling. Oikos, 2002. 98(3): pp. 523–533, https://doi.org/10.1034/j.1600-0706.2002.980317.x.
7. Sherman, R.A. and F.A. Wyle, Low-cost, Low-maintenance Rearing of Maggots in Hospitals, Clinics, and Schools. American Journal of Tropical Medicine and Hygiene, 1996. 54(1): pp. 38–41, https://doi.org/10.4269/ajtmh.1996.54.38.
8. Parry, N.J., E. Pieterse, and C.W. Weldon, Longevity, Fertility and Fecundity of Adult Blow Flies (Diptera: Calliphoridae) Held at Varying Densities: Implications for Use in Bioconversion of Waste. Journal of Economic Entomology, 2017. 110(6): pp. 2388–2396, https://doi.org/10.1093/jee/tox251.
9. Rosati, J.Y., et al., Estimating the Number of Eggs in Blow Fly (Diptera: Calliphoridae) Egg Masses Using Photographic Analysis. Journal of Medical Entomology, 2015. 52(4): pp. 658–662, https://doi.org/10.1093/jme/tjv053.
10. El-Moaty, Z.A. and A.E.M. Kheirallah, Developmental Variation of the Blow Fly Lucilia sericata (Meigen, 1826) (Diptera: Calliphoridae) by Different Substrate Tissue Types. Journal of Asia-Pacific Entomology, 2013. 16(3): pp. 297–300, https://doi.org/10.1016/j.aspen.2013.03.008.
11. Barnes, K.M. and D.E. Gennard, Rearing Bacteria and Maggots Concurrently: A Protocol Using Lucilia sericata (Diptera: Calliphoridae) as a Model Species. Applied Entomology and Zoology, 2013. 48(3): pp. 247–253, https://doi.org/10.1007/s13355-013-0181-7.
12. Clark, K., L. Evans, and R. Wall, Growth Rates of the Blowfly, Lucilia sericata, on Different Body Tissues. Forensic Science International, 2006. 156(2–3): pp. 145–149, https://doi.org/10.1016/j.forsciint.2004.12.025.
13. Daniels, S., K. Simkiss, and R.H. Smith, A Simple Larval Diet for Population Studies on the Blowfly Lucilia sericata (Diptera: Calliphoridae). Medical and Veterinary Entomology, 1991. 5(3): pp. 283–292, https://doi.org/10.1111/j.1365-2915.1991.tb00554.x.
14. Gallagher, M.B., S. Sandhu, and R. Kimsey, Variation in Developmental Time for Geographically Distinct Populations of the Common Green Bottle Fly, Lucilia sericata (Meigen). Journal of Forensic Sciences, 2010. 55(2): pp. 438–442, https://doi.org/10.1111/j.1556-4029.2009.01285.x.
15. Gasz, N.E. and M.L. Harvey, A New Method for the Production of Sterile Colonies of Lucilia sericata. Medical and Veterinary Entomology, 2017. 31(3): pp. 299–305, https://doi.org/10.1111/mve.12232.
16. Moe, S.J., N.C. Stenseth, and R.H. Smith, Density-dependent Compensation in Blowfly Populations Give Indirectly Positive Effects of a Toxicant. Ecology, 2002. 83(6): pp. 1597–1603, https://dx.doi.org/10.1890/0012-9658(2002)083[1597:DDCIBP]2.0.CO;2.
17. Zhang, B., et al., A Simple, Heat-sterilizable Artificial Diet Excluding Animal-derived Ingredients for Adult Blowfly, Lucilia sericata. Medical and Veterinary Entomology, 2009. 23(4): pp. 443–447, https://doi.org/10.1111/j.1365-2915.2009.00835.x.
18. Muntzer, A., et al., Temperature-dependent Lipid Metabolism in the Blow Fly Lucilia sericata. Medical and Veterinary Entomology, 2015. 29(3): pp. 305–313, https://doi.org/10.1111/mve.12111.
19. Linhares, A.X. and R.P. Avancini, Ovarian Development in the Blowflies Chrysomya putoria and C. megacephala on Natural Diets. Medical and Veterinary Entomology, 1989. 3(3): pp. 293–295, https://dx.doi.org/10.1111/j.1365-2915.1989.tb00231.x.
20. Wall, R., V.J. Wearmouth, and K.E. Smith, Reproductive Allocation by the Blow Fly Lucilia sericata in Response to Protein Limitation. Physiological Entomology, 2002. 27(4): pp. 267–274, https://doi.org/10.1046/j.1365-3032.2002.00296.x.
21. Wardhaugh, K.G., et al., The Relationship between Dung Quality and Oocyte Resorption in Laboratory and Field Populations of Lucilia cuprina. Entomologia Experimentalis et Applicata, 2008. 126(3): pp. 179–193, https://doi.org/10.1111/j.1570-7458.2007.00659.x.
22. Wall, R., N. French, and K.L. Morgan, Effects of Temperature on the Development and Abundance of the Sheep Blowfly Lucilia sericata (Diptera: Calliphoridae). Bulletin of Entomological Research, 1992. 82(1): pp. 125–131.
23. Williams, H. and A.M.M. Richardson, Life History Responses to Larval Food Shortages in Four Species of Necrophagous Flies (Diptera: Calliphoridae). Australian Journal of Ecology, 1983. 8(3): pp. 257–263, https://dx.doi.org/10.1111/j.1442-9993.1983.tb01323.x.
24. Smith, P.H., L. Barton Browne, and A.C.M. van Gerwen, Sperm Storage and Utilisation and Egg Fertility in the Sheep Blowfly, Lucilia cuprina. Journal of Insect Physiology, 1988. 34(2): pp. 125–129, https://doi.org/10.1016/0022-1910(88)90164-3.
25. Browne, L.B., A.C.M. Vangerwen, and P.H. Smith, Relationship between Mated Status of Females and Their Stage of Ovarian Development in Field Populations of the Australian Sheep Blowfly, Lucilia cuprina (Wiedemann) (Diptera, Calliphoridae). Bulletin of Entomological Research, 1987. 77(4): pp. 609–615, https://doi.org/10.1017/S0007485300012116.
26. Butterworth, N.J., P.G. Byrne, and J.F. Wallman, The Blow Fly Waltz: Field and Laboratory Observations of Novel and Complex Dipteran Courtship Behavior. Journal of Insect Behavior, 2019. 32(2): pp. 109–119, https://doi.org/10.1007/s10905-019-09720-1.
27. Queiroz, M.M.D.C., R.P. De Mello, and N.M. Da Serra Freire, The Effect of Different Proportions of Males and Females over the Chrysomya albiceps (Wiedemann 1819) (Diptera, Calliphoridae) Biotic Potential and Longevity under Laboratory Conditions. Memorias do Instituto Oswaldo Cruz, 1996. 91(2): pp. 243–247, https://dx.doi.org/10.1590/S0074-02761996000200023.
28. Mackerras, M.J., Observations on the Life-histories, Nutritional Requirements and Fecundity of Blow-flies. Bulletin of Entomological Research, 1933. 24: pp. 353–362, https://dx.doi.org/10.1017/S0007485300031680.
29. Wall, R., The Reproductive Output of the Blowfly Lucilia sericata. Journal of Insect Physiology, 1993. 39(9): pp. 743–750, https://doi.org/10.1016/0022-1910(93)90049-W.
30. Hayes, E.J., R. Wall, and K.E. Smith, Mortality Rate, Reproductive Output, and Trap Response Bias in Populations of the Blowfly Lucilia sericata. Ecological Entomology, 1999. 24(3): pp. 300–307, https://doi.org/10.1046/j.1365-2311.1999.00194.x.
31. Wooldridge, J., L. Scrase, and R. Wall, Flight Activity of the Blowflies, Calliphora vomitoria and Lucilia sericata, in the Dark. Forensic Science International, 2007. 172(2–3): pp. 94–97, https://doi.org/10.1016/j.forsciint.2006.12.011.
32. Ody, H., M.T. Bulling, and K.M. Barnes, Effects of Environmental Temperature on Oviposition Behavior in Three Blow Fly Species of Forensic Importance. Forensic Science International, 2017. 275: pp. 138–143, https://doi.org/10.1016/j.forsciint.2017.03.001.
33. Archer, M.S. and M.A. Elgar, Female Breeding-site Preferences and Larval Feeding Strategies of Carrion-breeding Calliphoridae and Sarcophagidae (Diptera): A Quantitative Analysis. Australian Journal of Zoology, 2003. 51(2): pp. 165–174, https://doi.org/10.1071/ZO02067.
34. Vogt, W.G. and T.L. Woodburn, The Influence of Temperature and Moisture on the Survival and Duration of the Egg Stage of the Australian Sheep Blowfly, Lucilia cuprina (Wiedemann) (Diptera, Calliphoridae). Bulletin of Entomological Research, 1980. 70(4): pp. 665–671, https://dx.doi.org/10.1017/S0007485300007951.
35. Wall, R., K.M. Pitts, and K.E. Smith, Pre-adult Mortality in the Blowfly Lucilia sericata. Medical and Veterinary Entomology, 2001. 15(3): pp. 328–334, https://doi.org/10.1046/j.0269-283X.2001.00316.x.
36. Bo, Z., et al., Short-term Cold Storage of Blowfly Lucilia sericata Embryos. Insect Science, 2008. 15(3): pp. 225–228, https://doi.org/10.1111/j.1744-7917.2008.00204.x.
37. Gomes, L., W.A.C. Godoy, and C.J. Von Zuben, A Review of Postfeeding Larval Dispersal in Blowflies: Implications for Forensic Entomology. Naturwissenschaften, 2006. 93(5): pp. 207–215, https://doi.org/10.1007/s00114-006-0082-5.
38. von Zuben, C.J., F.J. von Zuben, and W.A.C. Godoy, Larval Competition for Patchy Resources in Chrysomya megacephala (Dipt., Calliphoridae): Implications of the Spatial Distribution of Immatures. Journal of Applied Entomology, 2001. 125(9–10): pp. 537–541, https://doi.org/10.1046/j.1439-0418.2001.00586.x.
39. Bernhardt, V., et al., Of Pigs and Men-Comparing the Development of Calliphora vicina (Diptera: Calliphoridae) on Human and Porcine Tissue. International Journal of Legal Medicine, 2017. 131(3): pp. 847–853, https://doi.org/10.1007/s00414-016-1487-0.
40. Sherman, R.A. and J.M. My-Tien Tran, A Simple, Sterile Food Source for Rearing the Larvae of Lucilia sericata (Diptera: Calliphoridae). Medical and Veterinary Entomology, 1995. 9(4): pp. 393–398, https://doi.org/10.1111/j.1365-2915.1995.tb00011.x.
41. Rabêlo, K.C.N., et al., Bionomics of Two Forensically Important Blowfly Species Chrysomya megacephala and Chrysomya putoria (Diptera: Calliphoridae) Reared on Four Types of Diet. Forensic Science International, 2011. 210(1–3): pp. 257–262, https://doi.org/10.1016/j.forsciint.2011.03.022.
42. Baer, W.S., The Treatment of Chronic Osteomyelitis with the Maggot (Larva of the Blow Fly). The Journal of Bone and Joint Surgery. American Volume, 1931. 13: pp. 438–475, https://doi.org/10.1007/s11999-010-1416-3.
43. Okorie, T.G. and J. Okeke, Comparative Studies on the Blowfly Lucilia caesar (Diptera, Calliphoridae) Reared from 3 Rearing Media Prepared from Local Materials and the Standard Snyder Medium. Insect Science and Its Application, 1990. 11(2): pp. 143–148, https://dx.doi.org/10.1017/S1742758400010493.
44. Reddy, P.V.R., V.V. Rajan, and A. Verghese, A Non-meat-based Artificial Diet and Protocol for Mass searing of Chrysomya megacephala (Fab.) (Diptera: Calliphoridae), an Important Pollinator of Mango. Current Science, 2015. 108(1): pp. 17–19.
45. Anonymous. Malaysian Biotechnology Corporation: Interview With The CEO, Iskandar Mizal Mahmood. Scientific American worldVIEW. A Global Biotechnology Perspective. 2011.
46. Carreno, S.P., et al., Protophormia terraenovae. A New Allergenic Species in Amateur Fishermen of Caceres, Spain. Allergologia et Immunopathologia, 2009. 37(2): pp. 68–72, http://dx.doi.org/10.1016/S0301-0546(09)71107-3.
47. Wolff, H. and C. Hansson, Rearing Larvae of Lucilia sericata for Chronic Ulcer Treatment — An Improved Method. Acta Dermato-Venereologica, 2005. 85(2): pp. 126–131, https://doi.org/10.1080/00015550510025533.
48. Rivers, D.B., et al., Water Balance Characteristics of Pupae Developing in Different Size Maggot Masses from Six Species of Forensically Important Flies. Journal of Insect Physiology, 2013. 59(5): pp. 552–559, https://doi.org/10.1016/j.jinsphys.2013.03.002.
49. Mądra-Bielewicz, A., K. Frątczak-Łagiewska, and S. Matuszewski, Blowfly Puparia in a Hermetic Container: Survival under Decreasing Oxygen Conditions. Forensic Science, Medicine, and Pathology, 2017. 13(3): pp. 328–335, https://doi.org/10.1007/s12024-017-9892-3.
50. Aigbodion, F.I., I.N. Egbon, and A.J. Obuseli, Pathogens of Medical Importance Isolated from Phaenicia (Lucilia) sericata (Diptera:Calliphoridae) in Benin City, Nigeria. Pakistan Journal of Biological Sciences, 2013. 16(23): pp. 1791–1795, https://doi.org/10.3923/pjbs.2013.1791.1795.
51. Chan, Q.E., M.A. Hussain, and V. Milovic, Eating out of the Hand, Maggots — Friend or Foe? Journal of Plastic, Reconstructive and Aesthetic Surgery, 2012. 65(8): pp. 1116–1118, https://doi.org/10.1016/j.bjps.2012.01.014.
52. Terterov, S., et al., Posttraumatic Human Cerebral Myiasis. World Neurosurgery, 2010. 73(5): pp. 557–559, https://doi.org/10.1016/j.wneu.2010.01.004.
53. Connelly, K., et al., Wohlfahrtiimonas chitiniclastica Bloodstream Infection Due to a Maggot-infested Wound in a 54-year-old Male. Journal of Global Infectious Diseases, 2019. 11(3): pp. 125–126, http://dx.doi.org/10.4103/jgid.jgid_58_18.
54. Kawabata, T., et al., Induction of Antibacterial Activity in Larvae of the Blowfly Lucilia sericata by an Infected Environment. Medical and Veterinary Entomology, 2010. 24(4): pp. 375–381, https://doi.org/10.1111/j.1365-2915.2010.00902.x.
55. Maleki-Ravasan, N., et al., New Insights Into Culturable and Unculturable Bacteria Across the Life History of Medicinal Maggots Lucilia sericata (Meigen) (Diptera: Calliphoridae). Frontiers in Microbiology, 2020. 11(505), https://doi.org/10.3389/fmicb.2020.00505.
56. Jiang, K.C., et al., Excretions/Secretions from Bacteria-pretreated Maggot Are More Effective against Pseudomonas aeruginosa Biofilms. PLoS ONE, 2012. 7(11): e49815, https://doi.org/10.1371/journal.pone.0049815.
57. Yeong, Y.S., et al., Scanning Electron Microscopic Evaluation of the Successful Sterilization of Lucilia cuprina (Wiedemann) Utilized in Maggot Debridement Therapy (mdt). Tropical Biomedicine, 2011. 28(2): pp. 325–332, https://www.msptm.org/files/325_-_332_Yeong_Y_S.pdf.
58. Kaplun, O., M. Pupiales, and G. Psevdos, Adjuvant Maggot Debridement Therapy for Deep Wound Infection Due to Methicillin-resistant Staphylococcus aureus. Journal of Global Infectious Diseases, 2019. 11(4): pp. 165–167, https://doi.org/10.4103/jgid.jgid_30_19.
59. Brundage, A.L., T.L. Crippen, and J.K. Tomberlin, Methods for External Disinfection of Blow Fly (Diptera: Calliphoridae) Eggs prior to Use in Wound Debridement Therapy. Wound Repair and Regeneration, 2016. 24: pp. 384–393, https://doi.org/10.1111/wrr.12408.
60. Paul, A.G., et al., Maggot Debridement Therapy with Lucilia cuprina: A Comparison with Conventional Debridement in Diabetic Foot Ulcers. International Wound Journal, 2009. 6(1): pp. 39–46, https://doi.org/10.1111/j.1742-481X.2008.00564.x.
61. Wang, S.Y., et al., Clinical Research on the Bio-debridement Effect of Maggot Therapy for Treatment of Chronically Infected Lesions. Orthopaedic Surgery, 2010. 2(3): pp. 201–206, https://doi.org/10.1111/j.1757-7861.2010.00087.x.
62. Berkebile, D.R. and S.R. Skoda, Chemicals Useful for Separating Egg Masses of the Screwworm (Diptera : Calliphoridae). Southwestern Entomologist, 2002. 27(3–4): pp. 297–299.
63. PIC/S, Guide to Good Manufacturing Practice for Medicinal Products — Annexes. 2017, Pharmaceutical Inspection Convention Pharmaceutical Inspection Co-operation Scheme. https://picscheme.org/en/publications?tri=gmp#zone.
64. Sherman, R.A., C.E. Shapiro, and R.M. Yang, Maggot Therapy for Problematic Wounds: Uncommon and Off-label Applications. Advances in Skin & Wound Care, 2007. 20(11): pp. 602–610, https://doi.org/10.1097/01.ASW.0000284943.70825.a8.
65. Limsopatham, K., et al., Sterilization of Blow Fly Eggs, Chrysomya megacephala and Lucilia cuprina, (Diptera: Calliphoridae) for Maggot Debridement Therapy Application. Parasitology Research, 2017. 116(5): pp. 1581–1589, https://doi.org/10.1007/s00436-017-5435-9.
66. Nuesch, R., et al., Clustering of Bloodstream Infections during Maggot Debridement Therapy Using Contaminated Larvae of Protophormia terraenovae. Infection, 2002. 30(5): pp. 306–309, https://doi.org/10.1007/s15010-002-3067-0.
67. Merck. Wound Treatment with Larvae — Stories. https://www.merckgroup.com/en/stories/wound-treatment-with-larvae.html.
68. WHO. The International Pharmacopoeia, 3.2 Test for sterility. http://apps.who.int/phint/en/p/docf/.
69. TGA. TGA Guidelines for Sterility Testing of Therapeutic Goods. 2006. https://www.tga.gov.au/sites/default/files/manuf-sterility-testing-guidelines.pdf.
70. Thyssen, P.J., et al., Sterilization of Immature Blowflies (Calliphoridae) for Use in Larval Therapy. Journal of Medicine and Medical Sciences, 2013. 4(10): pp. 405–409 https://www.interesjournals.org/articles/sterilization-of-immature-blowflies-calliphoridae-for-use-in-larval-therapy.pdf.
71. Collier, R., Medicinal Maggots Cross Border at a Crawl. Canadian Medical Association Journal, 2010. 182(2): pp. E123-E124, https://doi.org/10.1503/cmaj.109-3134.
72. Vinogradova, E.B., Some Principles of Selecting Natural Material for Rearing and the Endogenous Processes in Laboratory Strains of the Blowfly Calliphora vicina R.-D. (Diptera, Calliphoridae). Entomological Review, 2011. 91(1): pp. 1–6, https://doi.org/10.1134/S0013873811010015.
73. Allen, M.L., D.R. Berkebile, and S.R. Skoda, Postlarval Fitness of Transgenic Strains of Cochliomyia hominivorax (Diptera: Calliphoridae). Journal of Economic Entomology, 2004. 97(3): pp. 1181–1185.
74. Allen, M.L. and P.J. Scholl, Quality of Transgenic Laboratory Strains of Cochliomyia hominivorax (Diptera: Calliphoridae). Journal of Economic Entomology, 2005. 98(6): pp. 2301–2306.
75. Blake, F.A.S., et al., The Biosurgical Wound Debridement: Experimental Investigation of Efficiency and Practicability. Wound Repair and Regeneration, 2007. 15(5): pp. 756–761, https://doi.org/10.1111/j.1524-475x.2007.00298.x.
76. Wilson, M.R., et al., The Impacts of Larval Density and Protease Inhibition on Feeding in Medicinal Larvae of the Greenbottle Fly Lucilia sericata. Medical and Veterinary Entomology, 2016. 30(1): pp. 1–7, https://doi.org/10.1111/mve.12138.
77. Čičková, H., et al., Growth and Survival of Bagged Lucilia sericata Maggots in Wounds of Patients Undergoing Maggot Debridement Therapy. Evidence-based Complementary and Alternative Medicine, 2013. https://doi.org/10.1155/2013/192149.
78. Pickles, S.F. and D.I. Pritchard, Quality Control of a Medicinal Larval (Lucilia sericata) Debridement Device Based on Released Gelatinase Activity. Medical and Veterinary Entomology, 2017. 31(2): pp. 200–206, https://doi.org/10.1111/mve.12220.
79. Stadler, F., R.Z. Shaban, and P. Tatham, Maggot Debridement Therapy in Disaster Medicine. Prehospital and Disaster Medicine, 2016. 31(1): pp. 79–84, https://doi.org/10.1017/s1049023x15005427.
80. Amorim, P., et al., Managing Perishability in Production-distribution Planning: A Discussion and Review. Flexible Services and Manufacturing Journal, 2013. 25(3): pp. 389–413, https://doi.org/10.1007/s10696-011-9122-3.
81. BioMonde. BioBag Ordering Guide. http://biomonde.com/images/US_Linked_Docs/BM029_US_02_0215.pdf.
82. Bohac, M., et al., Maggot Therapy in Treatment of a Complex Hand Injury Complicated by Mycotic Infection. Bratislava Medical Journal, 2015. 116(11): pp. 671–673, https://doi.org/10.4149/bll_2015_128.
83. Stadler, F., Distribution Logistics, in A Complete Guide to Maggot Therapy: Clinical Practice, Therapeutic Principles, Production, Distribution, and Ethics, F. Stadler (ed.). 2022, Cambridge: Open Book Publishers, pp. 363–382, https://doi.org/10.11647/OBP.0300.17.
84. Stadler, F., Supply Chain Management for Maggot Debridement Therapy in Compromised Healthcare Settings. 2018. Unpublished doctoral dissertation, Griffith University, Queensland, https://doi.org/10.25904/1912/3170.
85. ISO. ISO 13485:2003 Medical Devices — Quality Management Systems — Requirements for Regulatory Purposes. https://www.iso.org/standard/36786.html.
86. Hamimi Abdul Razak, I., et al., ISO 13485:2003: Implementation Reference Model from the Malaysian SMEs Medical Device Industry. The TQM Journal, 2009. 21(1): pp. 6–19, https://doi.org/10.1108/17542730910924718.
87. Robitaille, D.E. and I. ebrary, ISO 9001:2008 for Small and Medium-sized Businesses. Vol. 2nd. 2010, Milwaukee: ASQ Quality Press.
88. Blackburn, J.K., et al., The Necrophagous Fly Anthrax Transmission Pathway: Empirical and Genetic Evidence from Wildlife Epizootics. Vector-Borne and Zoonotic Diseases, 2014. 14(8): pp. 576–583, https://doi.org/10.1089/vbz.2013.1538.
89. Böhnel, H., Household Biowaste Containers (Bio-bins) — Potential Incubators for Clostridium botulinum and Botulinum Neurotoxins. Water, Air, and Soil Pollution, 2002. 140(1–4): pp. 335–341, https://doi.org/10.1023/A:1020169520369.
90. Fischer, O.A., et al., Blowflies Calliphora vicina and Lucilia sericata as Passive Vectors of Mycobacterium avium subsp. avium, M. a. paratuberculosis and M. a. hominissuis. Medical and Veterinary Entomology, 2004. 18(2): pp. 116–122, http://dx.doi.org/10.1111/j.0269-283X.2004.00477.x.
91. Laurie, R., Larval Therapy: Is It Effective against MRSA? Journal of Community Nursing, 2010. 24(4): pp. 10–12.
92. WHO. Safe Management of Wastes from Health-care Activities — 2nd edition. 2014; 2nd: http://apps.who.int/iris/bitstream/10665/85349/1/9789241548564_eng.pdf?ua=1.
93. Stadler, F., et al., Maggot Menageries: High School Student Contributions to Medicinal Maggot Production in Compromised Healthcare Settings. Citizen Science: Theory and Practice, 2021. 6(1): 36, p. 1–16, http://doi.org/10.5334/cstp.401.