7. Framing the Problem and Identifying Potential Solutions
© 2022 Chapter Authors, CC BY-NC 4.0 https://doi.org/10.11647/OBP.0321.07
The creation of effective policy and practice starts by framing the problem to be solved. This requires deciding what is important, identifying the current and potential future threats, diagnosing the actual cause of the problems, and identifying solutions, including innovating to create new ones when required. In this chapter we describe various techniques that can be used to frame the problem including horizon scanning, situation models and theory of change diagrams. These can be used to identify the analytical questions and specific assumptions that underpin the assessment of evidence and decision making.
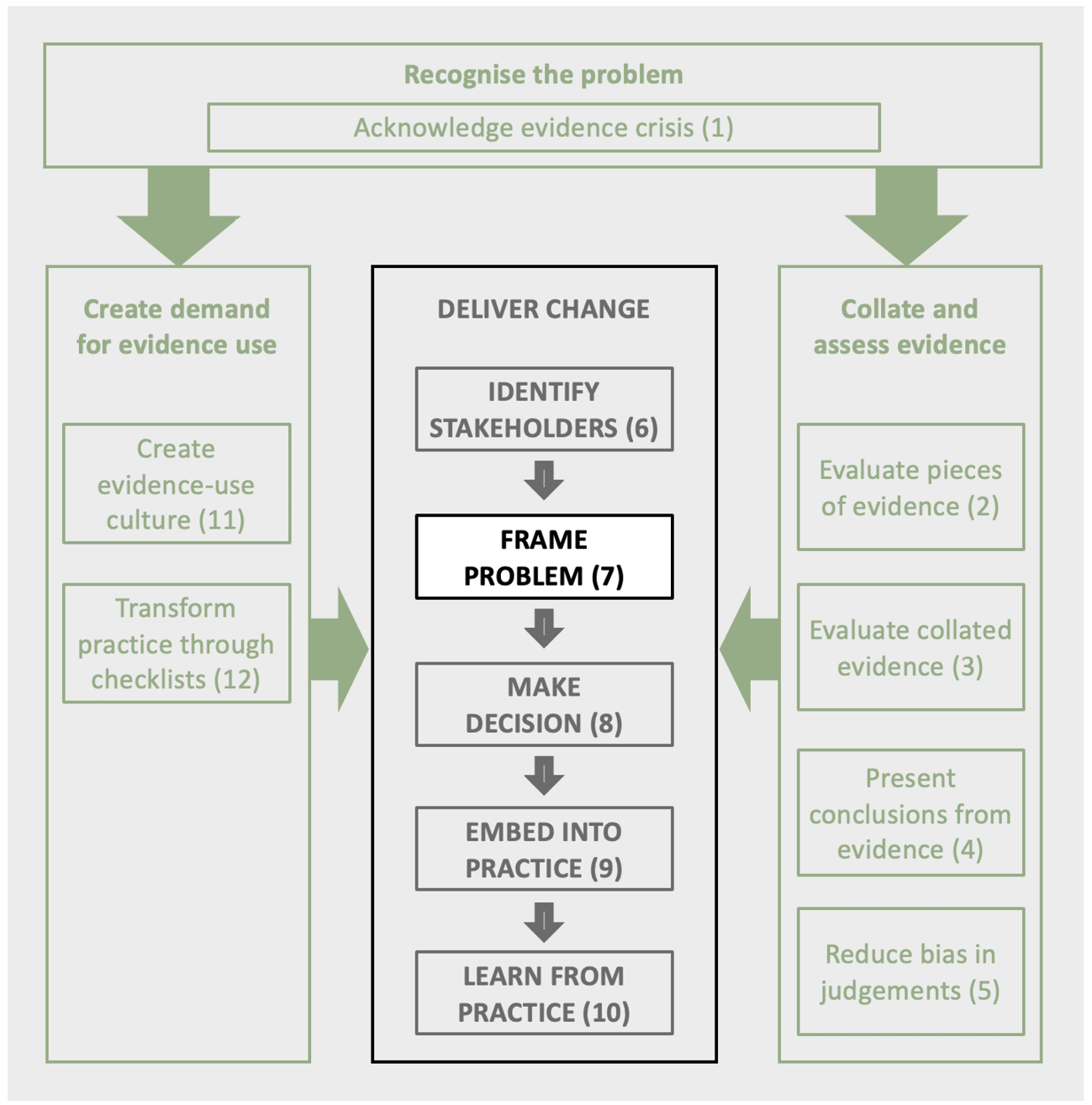
1 The Wilder Institute, 1300 Zoo Road NE, Calgary, Alberta, Canada
2 Women for the Environment Africa (WE Africa) Fellow
3 Conservation Science Group, Department of Zoology, University of Cambridge, The David Attenborough Building, Pembroke Street, Cambridge, UK
4 Biosecurity Research Initiative at St Catharine’s (BioRISC), St Catharine’s College, Cambridge, UK
5 Foundations of Success, 4109 Maryland Avenue, Bethesda, Maryland, USA
6 RSPB Centre for Conservation Science, 2 Lochside View, Edinburgh, UK
7.1 The Approach to Identifying Problems and Potential Solutions
As a basis for this chapter, we are assuming that the practice of conservation (which includes natural resource management) takes place through specific projects and broader programmes (CMP, 2020, Salafsky et al., 2022). Conservation is a process that (i) involves a defined project team understanding the threats, drivers, and opportunities in a given situation, (ii) agreeing on a desired future state for the situation, and then (iii) deciding on, implementing, and managing one or more strategies that involve taking particular actions that are designed to achieve these goals and objectives. This process, which can be applied at any spatial or temporal scale, is implemented through various planning and decision support frameworks (Schwartz et al., 2017). Although it may seem like every organisation has its distinct proprietary framework, at their core, most of these approaches involve a common iterative series of steps that include analysing the situation, identifying and comparing different potential intervention strategies, implementing these strategies, monitoring and evaluating the results of these strategies, and then adaptively managing and learning based on the findings.
A typical process for initiating a project would be:
- Decide upon the scope of the project and identify features of interest.
- Collate knowledge about the species, habitat, cultural and environmental features present.
- Identify current and future threats and opportunities relevant to the project, for example using diagnosis techniques, horizon scanning or scenario planning.
- Define goals and objectives for the project.
- Identify possible responses to the threats and opportunities. This may involve solution scanning to list existing options and innovation to develop new options, then using theory of change and assessing evidence of effectiveness to refine the list of options.
- Identify questions for which further evidence is needed.
These elements seem obvious, but there are numerous examples of projects selecting peculiar goals or objectives, failing to identify the real problem or selecting inefficient options for implementation. For example, many conservation projects undertake mangrove planting as an action, yet this often fails because the goal is framed in terms of the number of trees planted rather than the number surviving, the focus is on planting trees in new areas rather than improving and expanding surviving mangrove forest patches using targeted planting, or because planting is carried out in inappropriate topography or using poorly adapted species for the local conditions (Kodikara et al., 2017).
In contrast, when these elements are followed, they can lead to justifiable, targeted and effective conservation action. Following news of the rapid loss of shorebirds in the east Asian flyway, and the imminent extinction of the spoon-billed sandpiper Calidris pygmaea, the IUCN commissioned a situation analysis of the Yellow Sea (MacKinnon et al., 2012). This 70-page IUCN publication documented the evidence for the importance of the Yellow Sea, the evidence of declines in biodiversity and various threats including habitat loss by land reclamation. This was a critical stage in laying down the problem and led to the eventual designation of the Yellow Sea as a World Heritage Site (Crockford, 2018).
This chapter discusses each of these six elements in turn and, by outlining the problem, sets the stage for subsequent chapters that describe how to resolve the problem and deliver change. There will often be some iteration between the steps. For example, although objectives will be based on an initial understanding of the system, they may raise further specific questions to investigate and be refined as these questions are answered. The process will be facilitated by an individual or team, consulting stakeholders and experts as appropriate with workshops, interviews, or questionnaires.
7.2 Defining the Scope of the Project and the Conservation Targets
The team behind a conservation project should start by defining its scope: spatially (e.g. a specific protected area, a geographic region) or thematically (e.g. a specific species, all the economic actors in a given fishery) or often by some combination of these (CMP, 2020). Within this scope, the team then identifies the specific features of interests that represent its goals. These typically include specific species and broader ecosystems for which the area is important, or even cultural features or elements of human wellbeing linked to biodiversity. In identifying features, it is important to take into account global, national, and local listings of endangered and threatened species and ecosystems. For example, a species that is locally abundant, but rare elsewhere, is a likely feature of interest. In the end, however, selecting both a project scope and its features of interest is at least as much a values-based decision as it is a scientific decision but the reasoning and the process should be transparent.
7.3 Understanding the Biological and Human System
7.3.1 Determining feature status
Once features of interest have been identified, it is important to assess their current status. This assessment is typically done by identifying one or more key attributes that help define the viability of the feature (CMP, 2020). Examples of key attributes include water chemistry for aquatic habitats, biological community composition, and access to food resources or recreational opportunities for humans.
Statements of significance identify the important features in the area, such as ‘high quality grassland habitat is located within the project site’, ‘it is predicted that in 10 years the site will be suitable for rainforest ecosystems’, or ‘the watershed ecosystem is essential for maintaining water quality and regulating flows for the town downstream’. These are all statements that can be backed up by evidence of the status of features, including: species ranges, presence/absence, population size or abundance; habitat presence/absence, quality or distribution; or ecosystem services provided.
Understanding the status of species and habitats is an important step in the design of subsequent actions (Pimm et al., 2014). For example, effectively avoiding and minimising the impact of new energy infrastructure (e.g. power lines, wind turbines) on bird species requires information on the species present in the area, the habitat quality, and their susceptibility to impact before decisions can be made on what actions may be appropriate to undertake (Bennun et al., 2021).
Evidence can also be compiled on the status of ecosystem services in the area of interest, and the uses of various components of the environment by different stakeholders. For example, a claim may be made in the design of a marine conservation project that 100 tons of fishery catch are currently landed per annum from the project area; that the mangrove habitats present are vital for the provision of firewood to local stakeholders; and the mangroves act as nursery habitats that play a major role in the fishery stock. Obtaining information on these uses and ecosystem services can be important to ensure that claims made in the project conception are backed up by appropriate evidence, thus reducing the risk of tackling inappropriate features of interest or having an incorrect theory of change resulting in an ineffective project.
7.3.2 Assessing changes in feature status
Designing a conservation project may also require evidence of changes in status — a key component in identifying the threats and opportunities that the project could address. For example, this may be changes in species populations over time, or changes in habitat quality or integrity.
Considering the change in status of the features of interest usually involves combining wide-scale and local information (Table 7.1) to assess if any changes observed are part of a broader pattern or specific to the site. In some cases, there is regular monitoring that provides the required information. Moussy et al. (2022) provide a database of nearly 1,200 monitoring schemes, ranging in start date from 1800 to 2018. In most cases, however, the evidence comprises a mixture of survey counts, old records and knowledge of local experts. Museum collections, old maps, photos, descriptions, and paintings (which may not be accurate or biologically correct) can all be useful for assessing longer-term changes over decades or centuries. In a particularly innovative study, Belgian researchers assessed changes in tree and shrub phenology over 35 years using archived television footage from the Tour of Flanders bicycle race (De Frenne et al., 2018).
Table 7.1 Potential actions concerning wide-scale and local changes.
Declining widely |
Stable/increasing widely |
|
Declining locally |
Decide if local action will be effective, or if impossible to reduce or reverse decline due to changes elsewhere |
Identify the local problem and consider whether feasible or necessary to resolve |
Stable/increasing locally |
Identify solutions within the local context and inform others |
Monitor |
7.3.3 Understanding how the system functions
To correctly identify threats, opportunities and possible actions it is necessary to understand the functioning of systems around the features of interest. For example, the key to determining why a species has declined usually lies in a fundamental appreciation of its ecology and local context. This can be achieved by carrying out a literature review and looking at existing empirical studies to understand: (1) the ecological requirements such as habitat preferences, diet, breeding sites, or germination requirements; (2) which external factors affect demographic rates such as reproduction and mortality; and (3) large-scale correlations between distribution and abundance, and key resources or environmental factors. New observation, experiments and analyses may be needed to confirm theories or fill knowledge gaps.
As a specific example, the conservation of the large blue butterfly Phenagris arion in Britain required an understanding of the species’ larval dependence on a single host-ant species whose own ecological requirements are so specialised that subtle changes in grassland management and vegetation structure could cause its replacement by ecologically similar but unsuitable species. Only when this was understood could appropriate amendments to grassland management be recommended to drive recovery or re-establishment of the butterfly (Thomas et al., 2009). This in turn prompted wider recognition that diagnosis of larval habitat quality and its environmental drivers is central to the evidence-based conservation of many butterflies (Thomas et al., 2011). Section 7.4.2 explores diagnosis of declines in more detail.
Understanding socioeconomic systems can also inform conservation management. This is especially true for invasive alien species, where knowledge of how the species is introduced (the pathways and vectors involved) can highlight areas for intervention. Researchers in North America worked out that shipping activity was a major driver of invasions in the Great Lakes basin, with ballast water release being responsible for around 65% of invasions in the late 20th Century. Canada and the United States subsequently introduced strict ballast water exchange regulations, which reduced the rate of discovery of new non-native species in the Great Lakes by 85% (Ricciardi and MacIsaac, 2022).
7.4 Identifying Threats and Opportunities
7.4.1 Characterising current threats
Building on an understanding of the relevant systems, the project team can identify the threats that are affecting their features of interest. Following CMP (2020), this includes both direct threats (aka pressures) and indirect threats (aka root causes or underlying causes). The aim is to produce a reasonably comprehensive list, including those threats that may not be obvious and not only those that the project team (currently) has the capacity to address.
Direct threats are primarily human activities that immediately degrade a feature of interest (e.g. unsustainable fishing, unsustainable hunting, oil drilling, construction of roads, release of industrial wastewater, or introduction of invasive alien species). Direct threats can also be natural phenomena, perhaps altered by human activities (e.g. increase in extreme storm events or increased evaporation due to global climate change) or, in rare cases, with an impact increased by other human activities (e.g. a potential tsunami that threatens the last remaining population of an Asian rhino already endangered and range-restricted due to poaching). Direct threats can range from obvious and rapid (e.g. deforestation) to subtle and long-term (e.g. changes in grazing) and they often interact and combine to produce cumulative and more complex outcomes. For example, habitat fragmentation, invasive species and climate change can interact to increase impacts from each threat (Macinnis-Ng et al., 2021). Understanding how specific threats are likely to develop enables appropriate planning.
Indirect threats are drivers of direct threats, such as policies around logging or prescribed burning, or market forces that influence fishing pressure. It is usually easier to work backwards from targets, identifying direct threats then considering possible underlying causes for these.
In many cases, there may be a complex series of biophysical factors that link a given threat to a feature of interest. For example, the threat of land use change (e.g. ploughing of former grassland sites) may result in increased sediment input to waterways, which can affect biological features through eutrophication and resulting in changes in oxygen or light availability. It can be useful to explicitly map out these factors to clarify the logic linking threats to targets, and to identify possible intervention options.
7.4.2 Diagnosis: Identifying the likely cause of declines
Based on evidence of the timing and location of change, as well as ecological knowledge of the system in which these changes are taking place, candidate causes of species decline, habitat deterioration or other processes can be listed to inform investigation and determine which causes are most important (Green, 2002; Daszak et al., 2003). If the quality of knowledge is high and causal links are well understood, then this list may be short and arrived at with confidence. If the quality of knowledge is low, then careful judgement is needed: a conservative approach may inadvertently exclude an important cause, whereas a more inclusive approach risks creating an impractically long list. Diagnosis then proceeds by seeking to understand for which of the candidate causes on the list is the strongest evidence that they have driven the observed decline. Sometimes — for instance if a species is declining rapidly — a decision must be made based on the currently available evidence, even if imperfect, then monitored and revised if and when new evidence comes to light.
Here we use a range of species examples to illustrate some of the approaches that can be employed. We first consider approaches that can be used when we are in the fortunate position of being able to call upon spatially and temporally extensive distribution, population and demographic rate data to support diagnosis. Secondly, we consider approaches where such data are unavailable and instead we may need to rely upon primary field studies at one or a small number of sites.
The key issue is usually not whether a threat is causing some change but the magnitude of its impact, whether it is a major contributor to overall status change and how it is changing over time. Claims, such as ‘songbird species at our site are severely impacted by overexploitation due to the pet trade’ or ‘habitat degradation caused by dredging activities outside the marine protected area are a significant threat to marine biodiversity’, need estimates of the frequency, magnitude and overall consequence of threatening processes in order to be meaningfully quantified and addressed by actions.
Evidence on threats can come from a variety of sources including scientific studies, databases of threat data or expert and local knowledge. Evidence of threats can also be collected from project sites directly, and during project implementation. Increasingly, new technologies are providing near real-time information on threatening processes that can be used as a source of evidence. For example, GLAD (Global Analysis and Discovery) deforestation alerts are issued for areas where deforestation activities have been detected, Global Fishing Watch offers near real-time monitoring of fishing activity globally, and Firecast provides near real-time alerts of fires occurring in natural habitats. Stephenson and Stengel (2020) provide a synthesis of databases with information on threats to biodiversity.
Lastly, some actors in conservation may also be working with those impacting biodiversity as a result of their actions and thus investigating threats or the likely changes in status in the absence of action. For example, businesses may be developing a new infrastructure project and designing mitigation measures to try and avoid, minimise and compensate for negative impact. Similarly, businesses may wish to enact mitigation measures in their supply chains to minimise impacts on biodiversity. In these instances, their mitigation strategies will be making claims about their impacts, which need to be based on appropriate evidence. Information can be collected on the extent and severity of impacts, so that action taken is appropriate and likely to achieve desired outcomes.
Diagnosis based on large-scale data
In some cases, basic historical information about the population, habitat distribution and trends can reveal so much about causes of decline or endangerment that little further diagnostic work is needed. For example, the historical extirpation of the Eurasian bittern Botaurus stellaris in Britain was caused by the drainage of land for agriculture that removed the extensive reedbed habitats on which the species wholly depends. In this case, the cause of loss is clear and recent research has focused on understanding precisely how to restore and manage reedbeds with appropriate habitat structure and fish populations, as the basis for the successful recovery of the bittern (Brown et al., 2012).
Where the loss of carrying capacity is less clear-cut than in the bittern case, human impact may still generate measurable additional mortality or reduction in reproductive success. The effect on carrying capacity can then be estimated using ‘counterfactual simulation models’. These incorporate assumptions about density dependence which compare modelled population sizes with and without the effect of that impact (e.g. see Green et al., 2022, for the impact of lead poisoning on some raptor species across the European continent).
Where data for candidate external causes of decline are available at the same spatial and temporal scales as for trends in the species of interest then the diagnosis can also be assisted by testing for correlations between those causes and species trends. For example, measures of trends in agricultural practices and bird population indices were available for countries across Europe and were used to reveal strong spatial correlations between bird population declines and agricultural intensification (Donald et al., 2001).
Data on demographic rates can also be used as supplementary tests to strengthen the diagnosis. For example, studies of trends found that a decline in survival rates was the demographic mechanism driving population decline for many farmland songbirds (Siriwardena et al., 1999). This implicated reductions in over-winter food supply due to changes in agriculture as a likely external cause, and consequently measures to ensure seed-rich habitats in winter on farmland are now commonplace in European agri-environment schemes. More rigorously, a ‘sufficiency test’ can be used to test whether the magnitude of the effect of the external factor on a demographic rate is enough to account for an observed population decline. In some cases, this may be a matter of simple arithmetic. For example, the maximum possible reproductive rate of the endangered California condor Gymnogyps californianus is so low that the documented high adult mortality rate must have driven the observed species decline (Snyder and Snyder, 2000). Given that lead poisoning was the known cause of the increased mortality rate, then this could be diagnosed with high confidence as the cause of the population decline and thus targeted by remedial actions.
Diagnosis based on field studies
Studies at single sites may struggle to separate causal from irrelevant factors that may be changing simultaneously, and demographic responses may be confounded by the impacts of flows of individuals to and from the surrounding environment (Green, 1995). As Sutherland (2000) put it, ‘…the main reason for failing to diagnose problems is the myopic concentration on single sites, where factors cannot be unravelled, rather than looking for general patterns across sites’. Wherever possible, diagnostic field studies should seek to be as representative of a species’ distribution and range as possible and should try to make comparisons among study sites with differing trends in populations and potential drivers. This favours working collaboratively across networks of sites where the same problem is apparent. Some drivers of population change may be difficult to detect and study (e.g. impacts of pollutants and disease across multiple sites) and, for mobile taxa, some factors may operate over long-distance migration routes or in remote, perhaps even unknown, migration destinations.
Across sites, comparative studies of patterns of occupancy, abundance and demographic rates between places where a species is declining and places where it is not can be especially enlightening (e.g. Peach et al., 2004), as can comparisons of the environment between places where a species persists and those from which it has been lost. For example, by comparing sites where the endangered silver-spotted skipper Hesperia comma butterfly persisted with those from which it had become extinct, Thomas et al. (1986) found that persistence was associated with grazing regimes that provided the short, sparse fescue-grass swards that attracted egg-laying females, thus paving the way for a successful conservation intervention based on grazing management. Where possible, it is useful to extend this approach to compare changes over time in environmental variables. For example, Stowe et al. (1993) showed that factors linked to grassland management and mowing distinguished locations that had lost or retained nesting corncrakes Crex crex. Repeat surveys then showed that subsequent changes in corncrake numbers were correlated with changes in those same factors (Green and Stowe, 1993), a more powerful basis for conservation action.
Correlative studies like those above may be the only practical option available for most conservation practitioners. However, management interventions suggested by these approaches should ideally be followed up with replicated experimental tests at large sites or networks of sites. Both ‘before–after’ and ‘control–intervention’ designs can be used to manipulate a putative cause of the decline and monitor the response. For example, initial studies across an array of lakes showed that threatened common scoter Melanitta nigra populations persisted at lakes with shallow water, abundant large invertebrate prey, and few stocked brown trout Salmo trutta. These results led to a replicated experiment to test whether the reduction of fish populations through targeted angling can restore lake invertebrate populations as a potential scoter conservation measure (Hancock et al., 2020).
Where they are possible, experimental approaches have the advantage of allowing an iterative, adaptive approach so that a putative diagnosis can be modified — or abandoned in favour of another candidate cause — based on the evidence gathered. In cases where conservation urgency (e.g. due to drastic decline or highly threatened populations) is combined with a lack of a strong pre-existing evidence base, then merging diagnosis and the testing of conservation solutions in this way may be the best course of action, especially if the ideal, replicated experiment is impractical. For example, a simple experiment implementing predator control at one site for three years, with additional collection of reference data for four years before and three years afterwards offered important diagnostic insights in showing that capercaillie Tetrao urogallus productivity was highest when predator control coincided with dry weather during the nestling period (Summers et al., 2004). Experiments focused on measuring a demographic rate (e.g. fledgling rate) can be more practical for mobile taxa, where flows of individuals can make population responses (e.g. changes in numbers of adult individuals) difficult to measure and interpret.
Combining approaches
Most attempts at diagnosis ultimately combine approaches. As an example, Box 7.1 summarises the steps taken to diagnose rapid and severe recent declines of three Gyps vulture species in Asia. Other case studies are summarised by Sutherland (2000) for the Lord Howe woodhen Hypotaenidia sylvestris and Green (2002) for the wandering albatross Diomedea exulans.
7.4.3 Red team/blue team
The red team vs blue team practice is an approach for challenging systems to discover weaknesses, which may threaten the normal functioning of the system and provide opportunities for proactive intervention. The red team entails an individual or group attempting to challenge a system while the blue team seeks to prevent it. It can either be a real, but carefully planned and controlled physical attempt (e.g. attempting to get an imitation gun through security), or an exercise imagining how the challenge develops. This is widely used in cybersecurity and security, such as tests to challenge airport security. It could also be used to test systems for protecting rare species to identify areas of weakness, identify ways in which a predator could cross a fence protecting sensitive species, or identify ways in which sensitive conservation information could be acquired.
7.4.4 Horizon scanning to explore potential futures
With numerous current urgent problems, it may seem unnecessary or even irresponsible to suggest considering future potential issues. However, appropriate consideration of change is important, in particular for strategic planning. Horizon scanning is the process of identifying trends and phenomena that may gain prominence and that are likely to have a high magnitude of future impact, but for which there is little current awareness (UK Government Office for Science, 2017). This foresight technique helps anticipate unforeseen risks so as to respond efficiently and quickly whilst threats are still manageable as well as capitalise on opportunities by allocating resources to realise novel advances or innovations (Sutherland and Woodroof, 2009).
Horizon scanning is a useful means of identifying forthcoming issues. A review (Sutherland et al., 2019a) undertaken a decade after the first annual conservation scan in 2009 (Sutherland et al., 2010b) showed that a number of the top 15 identified issues subsequently appeared to have considerable salience and impacts (e.g. microplastic pollution, synthetic meat, artificial life, use of mobile-sensing technology for data collection, deoxygenation of the oceans, and invasive Indo-Pacific lionfish). Alongside the horizon scan, data were collected in 2010 as to whether senior representatives of 12 conservation organisations had heard of these issues (Sutherland et al., 2012a). This showed that these issues were on the horizon and not widely known. For instance, although use of mobile-sensing technology for data collection may seem obvious now, it was only known by 69% of these senior conservation leaders in 2010.
Horizon scans have also demonstrated value in informing funding priorities and strategic planning. The Antarctic Horizon Scan and follow-on Antarctic Roadmap Challenges have been used as justification for polar strategic planning efforts worldwide (Wintle et al., 2020) including recent major investment in Australian research.
One key consideration is the scope of the scan, as some horizon scan topics are broad while others are narrow (see Table 7.2 for examples). They also vary in geographical scale, with many global, but some local. For invasive species, for example, horizon scans have been carried out across different geographic areas (Great Britain: Parrott et al., 2009, Roy et al., 2014; western Europe: Gallardo et al., 2016; global: Ricciardi et al., 2016). Some scans have been designed for identifying emerging public policy issues (Parker et al. 2014). Many others have considered social issues, such as changes affecting forest-linked livelihoods (Oldekop et al., 2020).
Table 7.2 Examples of diversity within environmental horizon scanning.
Regular scanning can be adopted to ensure continuous monitoring of the landscape, as done for the global horizon scan of conservation that has been carried out annually since 2009 (Sutherland et al., 2010b, 2022), or by periodically updating, as done for bioengineering (Wintle et al., 2017; Kemp et al., 2020).
Horizon scans typically consider either challenges or both challenges and opportunities. Another aspect to determine at the planning stage is whether the scan will include issues that are well-known but not being adequately addressed, as in the scan for forest management in Myanmar (Prescott et al., 2017), or whether it aims to identify topics that most readers are unaware of, as in the global conservation scan (Sutherland et al., 2022). A different approach is to consider all issues that affect a specific subject. For example, Sutherland et al. (2012b) listed all the current and potential threats impacting migratory shorebird conservation. This has the advantage of setting out a reasonably complete set of challenges rather than just the most urgent or least well-known ones. Another advantage of this approach is that it deals with the criticism that horizon scans miss important issues when, in fact, these were just excluded because they are reasonably well known. Taylor et al. (2021) took a combined approach in their horizon scan for the conservation of Mediterranean wetlands, identifying both ‘critical’ issues (ignoring novelty to focus on the significance of impacts) and ‘overlooked’ issues (taking both novelty and impact into account).
There are numerous ways to conduct horizon scans across science and policy (Sutherland et al., 2011; Wintle et al., 2020). The core principles of the approach described in Box 7.2 are to consult a wide community and then use a form of structured transparent, anonymised expert elicitation, especially versions of the Delphi Technique (Section 5.5), to produce a final list (Sutherland et al., 2011).
Horizon scanning can consider a range of future types of problems. ‘Black swans’ was introduced by Taleb (2007) as a term for difficult-to-predict, low-probability events that might be high impact. Black swans (the birds Cygnus atratus) were once not considered by Europeans, who had only seen white species, until reported from Australia. Following the popularity of the term, we now have ‘black elephants’ as well-known subjects that attract insufficient attention (from the term ‘the elephant in the room’), such as the overuse of antibiotics and resulting drug resistance. ‘Black jellyfish’ are well-recognised events that could become more serious or are more complicated than expected (i.e. they could have a nasty sting). COVID-19, for instance, is a zoonotic disease originating from a well-known family of viruses, but which suddenly led to the global pandemic in 2020.
7.4.5 Scenario planning for potential futures
Scenario planning (also known as scenario building or scenario analysis) can be used alongside horizon scanning as a foresight tool that helps explore what the future might be like. It helps with thinking, in advance, about potential future change and uncertainty, providing the basis for strategic thinking about possible responses — both to avoid traps and make the most of opportunities — synthesising knowledge and advancing systems understanding (Peterson et al., 2003). Furthermore, it helps forewarn decision makers of undesirable future impacts of change, support decisions in developing adaptive governance strategies, and discover implications that may come from alternative social-ecological development pathways and policy options (IPBES, 2016). Different types of scenarios are useful in different phases of the policy cycle: exploratory scenarios looking at different possible directions are useful in agenda setting, target-seeking scenarios are useful when designing options, policy-screening scenarios are useful during implementation, and retrospective policy evaluation is useful during the review (Figure 7.1; IPBES, 2016).
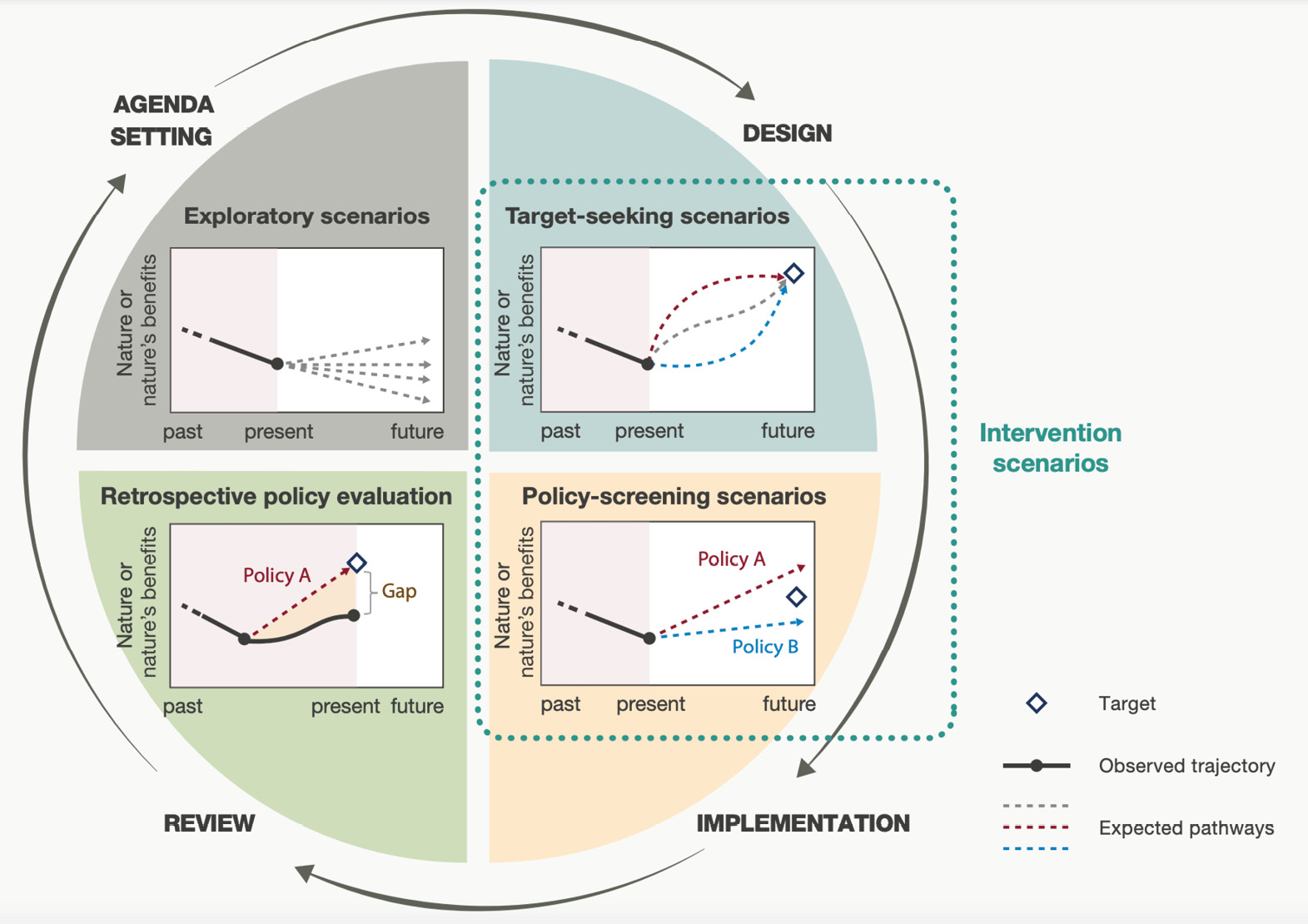
Figure 7.1 Scenarios to support decision making at different phases of the policy phase. (Source: © IPBES, 2016, CC-BY-NC-3.0)
There are multiple approaches to scenario planning, but it is generally developed through workshop discussions that allow groups (representing multiple stakeholders and experts) to identify a range of future visions (Box 7.3). Each of the identified scenarios is then detailed further, such as with a narrative or pathway exploring the consequences for existing or potential policy. This is then used as a basis for modelling, or to identify knowledge gaps.
The likely best-known example of scenarios are those created by the Intergovernmental Panel on Climate Change considering a range of societal responses. They then embed these various responses into their climatic models to predict the likely change over time under each scenario (IPCC, 2018). The model outputs have been widely used to explore the possible consequences of climate change, for example for biodiversity.
Scenarios developed for conservation applications range from envisioning a common desirable future for protected areas in Spain (Palomo et al., 2017), to assessing governance reforms for enhancing biodiversity outcomes in Australian landscapes (Mitchell et al., 2016), and developing alternative futures for global biological invasions (Roura-Pascual et al., 2021). Scenarios can provide the basis for deciding what to input into models; for example, models of the dynamics of saiga antelope Saiga tatarica parasite infection were underpinned by a range of current and plausible future scenarios of increasing livestock numbers, climate change and antiparasitic treatments (Khanyari et al., 2022).
7.5 Taking Stock
7.5.1 Summarising the problem
A situation model (or situation assessment diagram) is a tool that visually portrays the relationships among the different factors discussed so far, including the targets, direct and indirect threats, opportunities, biophysical stresses, and key actors related to a particular target or targets (Margoluis et al., 2009; CMP, 2020). It can greatly help in defining the project in a standardised and collaborative way. A good model illustrates the main cause-and-effect relationships that exist within the project area or theme. It should include the most important details, yet be as simple as possible. A situation model for an extensive project will need to be at a coarser grain than one for a smaller project. Models can be updated as a project progresses to reassess and re-strategise (Riley et al., 2003). New links can be added, or the specificity of existing links refined, as new evidence comes to light.
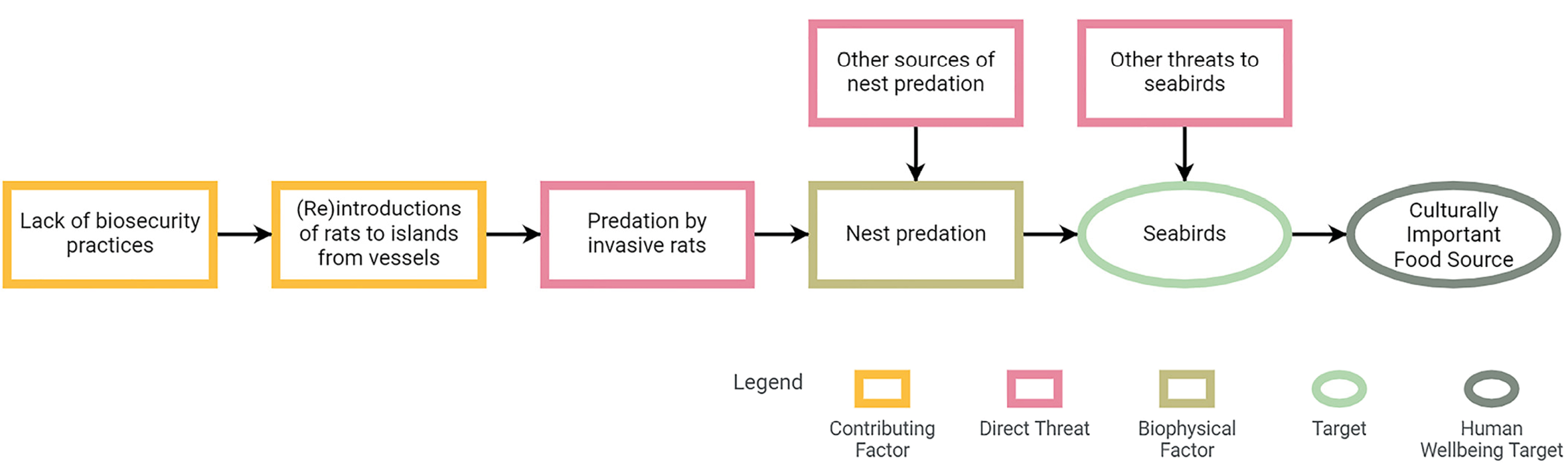
Figure 7.2 Excerpt from a situation model on protecting a seabird colony from invasive rodents. (Source: Salafsky et al., 2022, CC-BY-4.0). Note use of the term “Contributing Factor” to describe factors that could be considered indirect threats or opportunities for intervention.
7.5.2 Defining goals and objectives
With a clear understanding of the scope of a conservation project, and its situation, it is possible to determine the project’s goals and objectives. These outline the team’s desired future status for the features of interest, threats and opportunities (CMP 2020). Goals and objectives should be SMART: specific, measurable, achievable, results-oriented and time-bound. A sensible goal might therefore be: ‘Between 2020 and 2030, the available habitat for pond-breeding amphibians in Belize will be doubled through restoration and creation of new pond clusters’.
7.6 Identifying Potential Actions
7.6.1 Solution scanning
To achieve stated goals, conservationists will put in place different actions as part of their projects that aim to protect or restore biodiversity (or other features of interest). For example, in the UK it is common for infrastructure developments to erect bat boxes in an attempt to increase bat abundance in their project area, while farmers often create wildflower strips at the edge of fields to increase bird species richness and pollinator diversity. A reforestation programme may also aim to improve water quality or carbon storage, and reduce soil erosion; an alternative livelihoods programme may hope to improve incomes for local communities, whilst reducing unsustainable harvesting practices. Relevant actions will differ depending on the outcomes desired, the status of the features of interest, and the threatening processes present.
It is often sensible to start by considering all the possible (but reasonable) options for addressing the identified threats and exploiting the identified opportunities. Solution scanning is a more transparent and careful strategy than the traditional approach of selecting a subjective subset of policies using only the experience and beliefs of practitioners (Sutherland et al., 2014). While a complete evidence review for all options would nearly always be preferable, the time and effort required for such reviews mean they are often impractical in practice. The process of solution scanning entails bringing together a wide community to identify possible options for responding to a set of problems or opportunities. This is the initial stage, with no attempt to consider effectiveness. However, this stage is important; one study showed that practitioners had heard of just 57% of the possible 28 interventions (Walsh et al., 2015), suggesting decision makers may be blinkered in the range of available options. Two examples of how solution scanning has recently been applied are a list of possible actions for creating or enhancing ecosystem services (Sutherland et al., 2014) and a list of options for reducing the likelihood that future pandemics of zoonotic origin will emerge (Petrovan et al., 2021). Solution scanning can also be the first stage of subject-wide evidence synthesis, for example during collation, synthesis and assessments of the effectiveness of actions to protect biodiversity (Sutherland et al., 2019b).
The approach adopted for solution scanning (Box 7.4) is to create a list of the challenges, then compile a comprehensive list of possible solutions under each, consult more widely and make the scan available so it can be checked, used and updated. The issue as to whether an action is likely to work in practice is considered at a subsequent stage, when contextual information can be included. Depending on the topic, solution scanning can be carried out at different scales, ranging from quickly listing possible options to an extensive process aiming to be reasonably comprehensive and including structured searching of the literature and consulting a wide range of global experts.
For conservationists, conservationevidence.com and the latest edition of What Works in Conservation (Sutherland et al., 2021) provide a range of potential actions for a given threat. Doing nothing (or nothing new) is also an option; in most projects the costs and benefits of this should also be considered.
7.6.2 Innovating to devise novel solutions
Innovating, followed by testing, is critical for conservation, whether for novel research methods or techniques, or to improve and create new practical solutions. However, innovation in conservation practice is insufficient in comparison to other disciplines (Game et al., 2014).
Means for encouraging creativity include: experimentation; allocating time to creative side projects; tolerating obviously impractical ideas during initial discussions; embracing a degree of risk and failure; bringing together a group with different knowledge, perspectives and skills; meeting face-to-face; and setting ambitious goals (e.g. by adopting the 10x vs 10% mindset, as they do at Google, i.e. aiming to ambitiously improve something by 10 times, rather than just by 10%). The following paragraphs explore specific tools and approaches to stimulate innovation.
Continual improvement (kaizen)
Kaizen (‘doing better’ in Japanese) is the principle of continual innovation to improve. Much of the success of Toyota is attributed to kaizen. All departments from construction to accounts and management look at processes and consider means to improve. Similarly, in the 2000s, the British cycling team aimed to improve every component of competitive cycling — from the cleanliness of the maintenance workshop to the cyclists’ sleeping position. They suggested that even if each gain is just 1%, these improvements collectively result in large overall gains. Sutherland (2019) suggested that the widespread adoption of kaizen conservation, in which organisations and conservationists look for continual self improvement, would deliver conservation more effectively.
Reflection on the details of the problem
If exploring how to protect a particular species, consider its behavioural and habitat preferences and other requirements, and then what may be attractive and beneficial. For example, the attributes of nesting or hibernation sites give clues as to suitable designs for nest boxes or hibernacula. Equally, understanding the requirements of problematic species can generate innovative ideas for their control, for example by altering the habitat so that it no longer meets those requirements.
Brainstorming
Brainstorm by presenting a problem and encouraging the shouting out of possible solutions (which are documented e.g. on a flip chart) with the rule that no criticism is allowed; building on previous ideas is welcomed. It may be helpful to remove constraints like time, money and even laws of nature to encourage truly creative thinking! At the end of the process, the ideas are reviewed and the useful ones retained.
Physical models for inspiration
In this process materials are provided to make models, such as a hibernaculum or a nesting box that excludes predators, with the aim of generating novel practical design ideas. Building and testing prototypes, for example of wildlife tracking devices, can stimulate novel designs as practical problems are encountered.
Adopt and adapt existing solutions and technologies
Seek out and ask widely how others (including those from other disciplines) solve similar technical problems. In Mauritius, Tatayah et al. (2007) discovered that copper rings deterred African giant land-snails Achatina spp. from entering echo parakeet Psittacula eques nest cavities, thus preventing the birds from nesting. Newman and Showler (2007) were inspired by this to use copper rings to reduce losses of red helleborine Cephalanthera rubra to slug and snail herbivory in the Chiltern Hills, England. As another example, the algorithm used for astronomical pattern matching was used by Arzoumanian et al. (2005) to individually identify whale sharks Rhincodon typus by their spots, thus improving the understanding of populations and movements. Sometimes nature itself can inspire innovative design: remodelling the nose of the Japanese Shinkansen bullet train based on the streamlined beak of the kingfisher Alcedo atthis increased fuel efficiency and reduced the problem of sonic booms as the train exited tunnels. Bromeliads inspired the design of the Nucleário device (https://www.nucleario.com) that provides a reliable water supply to saplings planted for forest restoration.
Hackathons
These were created as a way for computer programmers to collaboratively explore software development with graphic and interface designers, product managers and others, but have since been adapted for conservation solution innovation. Crowdsourced participants are given problem statements and, with limited time and resources, are challenged to come up with practical, often technologically-driven solutions. Teams compete for prizes and prestige using the event to brainstorm ideas, develop prototypes and demonstrate designs. Examples of conservation hackathons are the Zoohackathon for wildlife trafficking (Zoohackathon, 2021) and the Grand Challenges for microfiber pollution, artisanal mining and climate-friendly cooling technologies (ConservationXLabs, 2022). ConservationXLabs winners are subsequently supported to develop and expand their solutions, catalysing innovation for the field and industry.
Gamifying conservation
Games can be used to identify novel possible actions by having teams seeking to solve challenges. In 2010, the World Bank Institute ran the online game EVOKE (https://www.urgentevoke.com/), which encouraged players to develop creative solutions to pressing social problems. Open source and open access platforms and databases lower barriers so multiple people can work on any one problem.
Prizes and awards
Prizes have been awarded to encourage solutions for specific problems; the most famous is the Longitude Prize offered in 1714, which delivered a solution for determining longitude. The annual Earthshot Prize, announced in 2020, has a similar objective of encouraging innovation for the world’s most extraordinary eco-solutions. Specific awards for innovative nature conservation include the Ulysses S. Seal Award for Innovation in Conservation, the Pathfinder Award for Innovation in Nature Conservation, and the annual Best Practice (Innovation) award given out by the UK Chartered Institute for Ecology and Environmental Management.
Incubators and accelerators
Incubation is a supportive process of innovators with an impactful idea to help meet investor requirements. Incubators work with innovators to ensure their ideas are relevant, scalable, and impactful. Acceleration is generally the last stage in growing promising ideas (often high-ambition and transformative) into initiatives with further development, validation and financing support. Incubators and accelerators for conservation are emerging and draw upon private-sector models for start-ups to create impactful, de-risked and exciting initiatives for investors and funders: the Luc Hoffmann Institute hosts an ideation–incubation–acceleration programme broadly across biodiversity conservation; the Conservation Finance Alliance Incubator identifies, supports and promotes innovative ideas and solutions to conservation finance challenges; IUCN and partners have set up the Nature+ Accelerator Fund.
7.6.3 Evaluating likely effects of actions
Designing a conservation project involves making claims or assumptions. These will often be about the effect of actions on biodiversity, for example will erecting bat boxes be effective at encouraging bats to roost? Will those bat boxes increase the population of bats in the area? Will the wildflower strips be effective at improving pollinator abundance and species richness? Sometimes assumptions will be about associated socioeconomic effects, for example is there evidence supporting the success of alternative livelihood programmes in increasing local incomes, or evidence showing that carbon storage is enhanced from reforestation programmes? Answering such questions requires evidence on the effectiveness of different actions — what works, and what does not.
It is vital to compile such evidence because sometimes actions presumed to be effective can be ineffective, or at worst harmful. For example, bat gantries have been frequently used in the UK to mitigate the impacts of road developments in areas where bats may frequently cross the infrastructure. Gantries were designed so that bats would use these structures to guide them safely above the road and the height of the incoming traffic. However, once evidence was compiled it showed these gantries were rarely used by bats (Berthinussen et al., 2021), meaning at least £1 million was wasted on ineffective mitigation (Sutherland and Wordley, 2017) and negative traffic threats and impacts for bats remained.
Evidence of the effectiveness of actions can take a range of forms. Effectiveness can be measured both quantitatively and qualitatively, and be from a range of sources (see Section 2.5). Effects can also comprise various measured outcomes, which can be difficult to compare. For example, the effectiveness of wildflower strips for biodiversity conservation is often measured using different metrics (e.g. nesting density, abundance, species richness), and with evidence focused on different species groups (e.g. butterflies, hoverflies, birds, small mammals). Noss (1990) provides a framework of indicators that can be used to measure effects on biodiversity at four organisational levels (genetic, population–species, community–ecosystem, and regional landscape) and including compositional, structural and functional components. A project may wish to compile evidence of the effectiveness of different actions for a range of different metrics. Chapters 2, 3 and 4 explain how to assess and draw conclusions from compiled evidence.
Ideally, evidence of effectiveness should be consulted during the design of a project, but such evidence can also be collected during pilot phases, trials or experiments before full implementation. This may be particularly important where there are large taxonomic or geographical gaps in the published evidence base for the effectiveness of actions (Christie et al., 2021; Junker et al., 2020). Even during implementation, monitoring of observed outcomes can help ensure project success and, if results are shared with the wider community, help build the global evidence base (see Chapter 10).
The effect of a conservation action can often be more complex than just directly impacting a feature of interest and the action may primarily be trying to influence threatening processes or behaviours. For example, a project may be hoping to improve the population outlook of a particular endangered species by reducing rates of hunting through an education programme. In this instance, evidence will also be needed on the impacts of education programmes on rates of hunting in the given context, rather than just evidence on whether reducing hunting benefits the endangered species.
Chapter 13 provides a list of resources which provide evidence of the effects of conservation actions, including some resources focused on biodiversity outcomes, and some focused on other environmental and social outcomes.
7.6.4 Evaluating actions based on other criteria
In practice, conservation actions will also need to be assessed on criteria other than effects on the features of interest or associated threats. The economic costs of actions may be an important consideration (see Section 2.4.4). The most effective action may exceed the budget of a conservation project. If two actions are expected to be similarly effective, one may be preferred because it is less expensive. Local attitudes, values, laws and politics might also restrict what actions can be used (see Section 2.4.5). Public opinion often favours non-lethal over lethal management of problematic species, for example, despite potentially lower effectiveness and higher economic costs (Roberts et al., 2018).
7.6.5 Theory of change
Theories of change are diagrams that illustrate how a given action, or set of actions, is expected to deliver a given outcome based on the available evidence (Figure 7.3). They are popular in many areas of conservation and development and are often expected as part of funding applications. Whereas a situation model (Figure 7.2) shows the system before taking action, the theory of change shows assumptions about how the system will respond after action (Margoluis et al., 2013; CMP, 2020). As such, they are complementary tools.
The example shown in Figure 7.3 illustrates how planned actions are intending to influence the situation expressed in the situation model of Figure 7.2, and which intermediate results and outcomes they are intending to achieve.
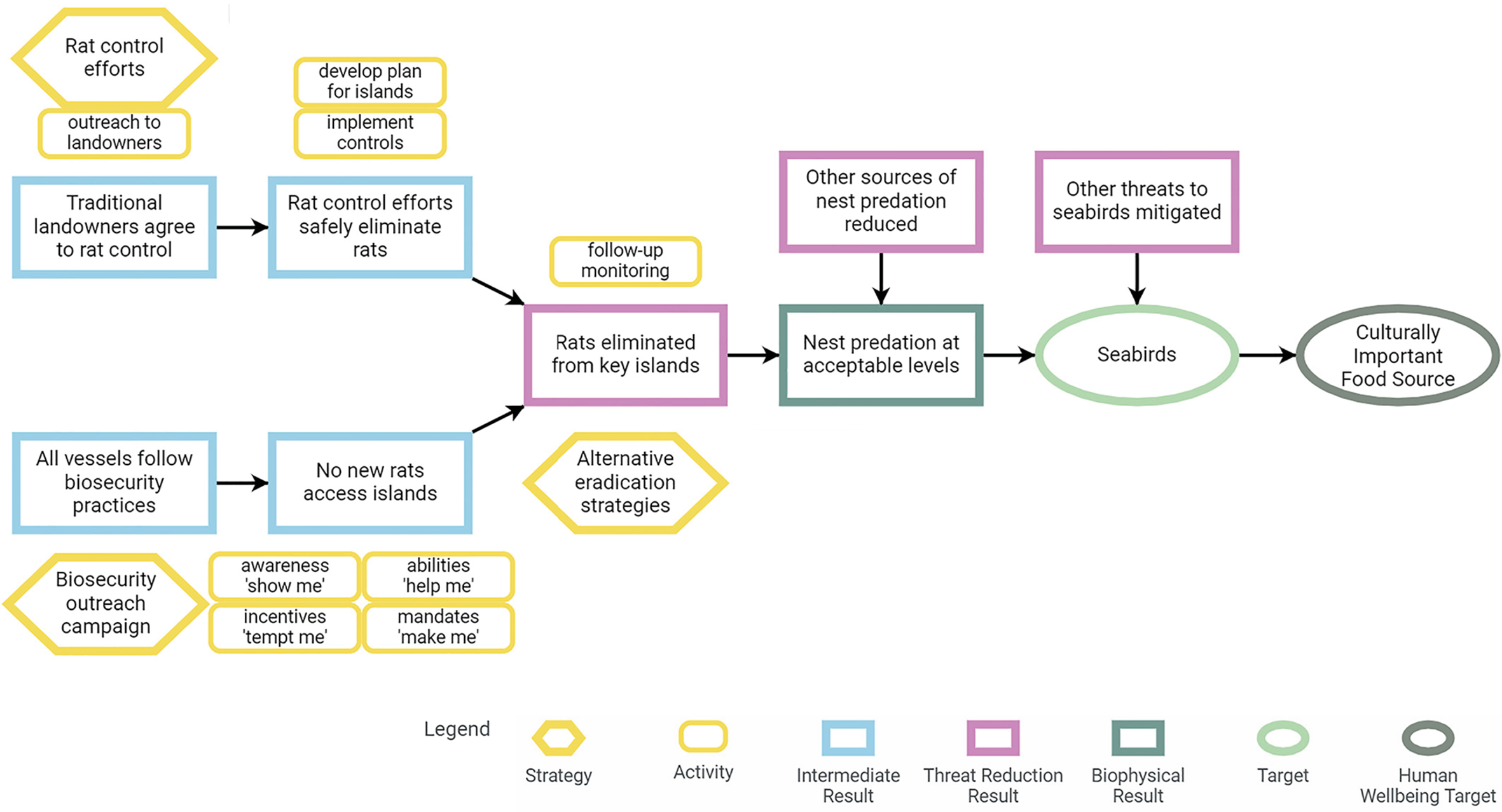
Figure 7.3 Example of a theory of change pathway diagram. (Source: Salafsky et al. 2022, CC-BY-4.0)
7.7 Developing Questions and Assumptions
7.7.1 Developing questions and assumptions in situation models and theories of change
There are many different types of questions and assumptions relevant to conservation policy and practice, each requiring different types of evidence. Some of these may be related to understanding the situation, such as the status of the target species (Section 7.3) or the cause of a threat (Section 7.4). Others are related to the effectiveness of an action or the conditions under which a given action might be effective (Section 7.6). A large part of the ‘art’ of evidence-based conservation thus involves understanding the system well enough to figure out the right set of assumptions to consider and the sequence in which these need to be assessed (USAID, 2018). For example, to help conserve seabirds on an island where rat predation might be an issue, the team may need to first confirm that rats are present on the island, then that they are at least a partial cause of seabird nest predation, and finally that poisoning might be an effective action to take to remove the rats given local rainfall patterns and the risks of accidental poisoning for non-target fauna.
The mental models created as part of situation models (Margoluis et al., 2009) (Figure 7.2) and the theory of change pathways (Margoluis et al., 2013) (Figure 7.3) become important tools to generate questions and assumptions. For example, five broad analytical questions need to be answered to justify the proposed rat eradication strategy (Figures 7.4 and 7.5):
- Do the seabird populations require conservation action?
- Are rats a major threat to the seabird populations?
- Is the proposed rat eradication strategy feasible and effective?
- Are alternative rat control strategies less feasible and/or effective?
- Can rats be prevented from re-invading the islands?
Questions are easier to answer if specific and well-formulated. For example, rather than the broad question ‘Are rats a major threat to our seabird populations?’, it is better to formulate a set of specific assumptions such as ‘nest predation is a major cause of seabird mortality’, ‘rats are the primary cause of seabird nest predation’, and ‘other sources of nest predation are less important’. To this end, it is usually helpful to break down each question into a set of component assumptions as shown in Figures 7.4 and 7.5.
Technically, every factor and every link between factors in situation models and theories of change represents an assumption. Whilst it is usually unrealistic to collect evidence for every assumption, instead, the need is to identify those that are critical as they are more uncertain or have higher risk consequences. The art lies in evaluating which assumptions will benefit from further scrutiny.
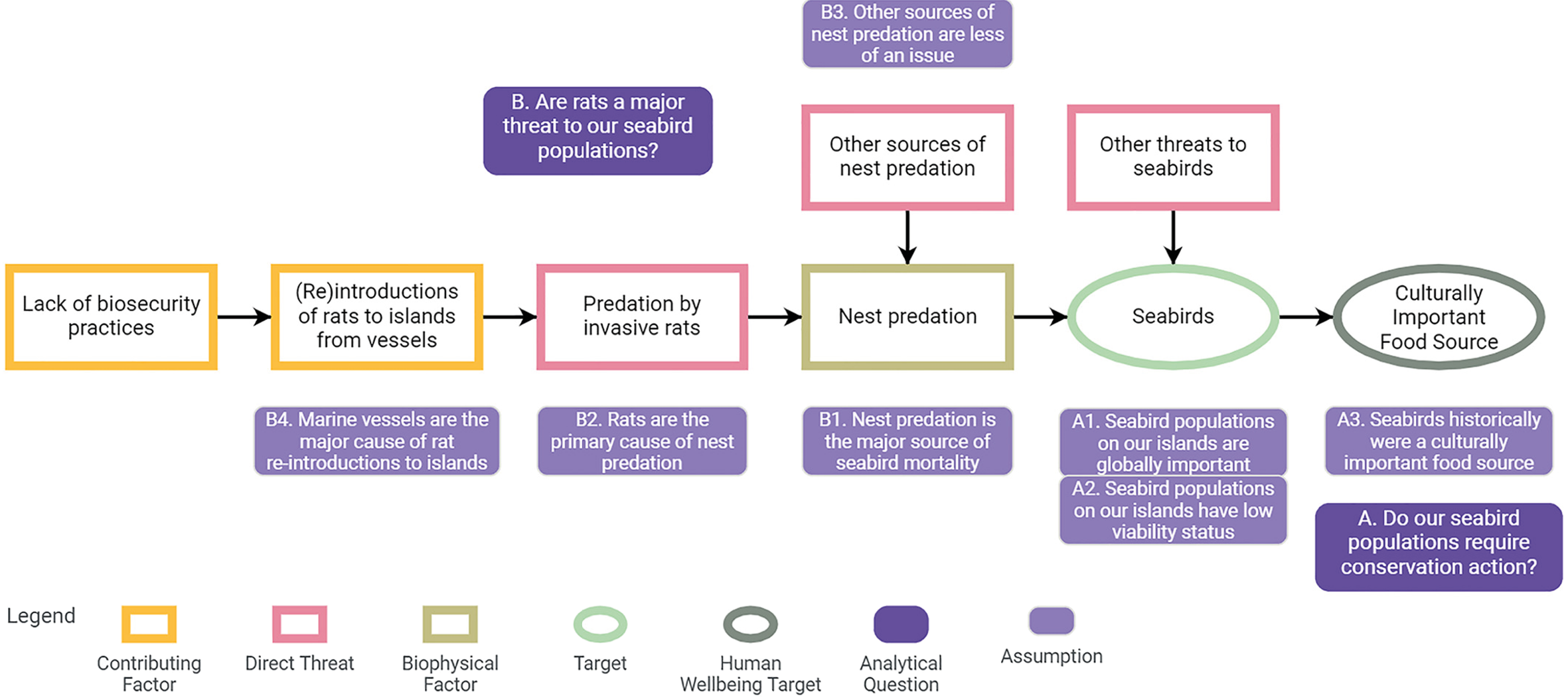
Figure 7.4 Examples of analytical questions and assumptions related to a situation model. (Source: Salafsky et al., 2022, CC-BY-4.0)
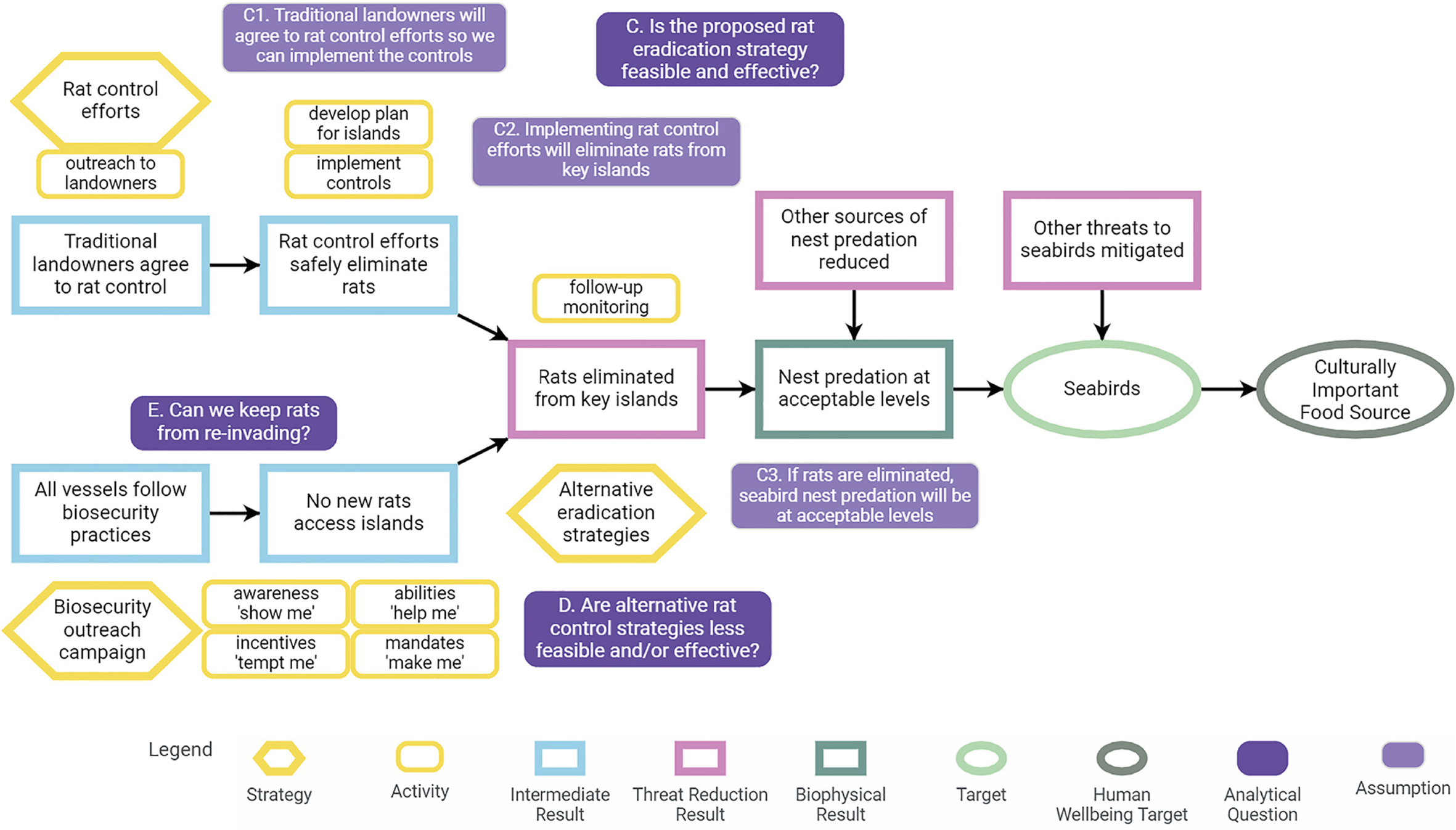
Figure 7.5 Examples of analytical questions and assumptions related to a theory of change. Note that assumptions for Questions D and E are not shown. (Source: Salafsky et al., 2022, CC-BY-4.0)
7.7.2 Identifying priority questions for policy and practice
Sutherland et al. (2006) created a process by which decision makers and researchers can work together to identify the questions that, if answered, would make the most difference in improving policy and practice. This has led to a wide range of such exercises focusing on subjects such as cetacean conservation (Parsons et al., 2015); habitat restoration (Ockendon et al., 2018); post-2015 development agenda (Oldekop et al., 2016); future of global agriculture (Pretty et al., 2010); microbial ecology (Antwis et al., 2017); Mediterranean wetland conservation (Taylor et al., 2021); Antarctic science (Kennicutt et al., 2015); UK poverty reduction (Sutherland et al., 2013); and UK biosecurity (Kemp et al., 2021). This process of creating research agendas is a promising way to identify what is needed to address societal challenges and risks, and can thus usefully inform policy and government strategies.
An analysis (Jucker et al., 2018) of the outputs of an exercise to identify priority questions for the conservation of global biological diversity a decade earlier (Sutherland, 2009) showed that, as of July 2016, seventy documents cited the exercise specifically to justify research on topics it highlighted. They also identified 21 questions that met their criteria for knowledge gaps and so needed further work. This shows such exercises are indeed used to generate new research and can be used to record progress in addressing priority questions.
Box 7.5 outlines how to adopt this approach. Our experience is that this process needs to combine the strengths of practitioners and policymakers, who know which knowledge gaps are important for practice and policy, and researchers, who can convert general interests into specific research questions.
7.7.3 Unpacking questions
The processes described above often identify gaps in knowledge across a range of subjects or topics, and questions that are critical to delivering action. Many questions asked as part of the process of decision making are general and need unpacking to make them more specific. For questions relating to actions or interventions, the PICO format is often used (Box 7.6).
An equivalent approach applies to other questions (EFSA, 2010; James et al., 2016). Questions about threats or impacts can be framed in a PECO format (population, exposure, comparison, outcome), for example ‘Do bumblebees with varroa mites have a lower reproductive output than bumblebees without varroa mites?’ Questions about the accuracy of tests or monitoring methods can be framed in a PIT format (population, index test, target condition), for example ‘Can eDNA sampling of flowers be used to detect the presence of endangered bees?’ Descriptive questions about prevalence, occurrence or incidence can be framed in a PO format (population, outcome), for example ‘What proportion of fish in UK rivers show signs of disease?’
As discussed in the previous section, it is often helpful to narrow down broad questions into specific ones, so that it is clear what data are needed to answer the question. So rather than asking ‘Are seabirds successfully nesting in Eastern Bay?’, the question could be ‘Are there at least 100 breeding pairs of ruby-crested puffins who have fledged an average of at least one chick during each of the last five breeding seasons in Eastern Bay?’
References
Antwis, R.E., Griffiths, S.M., Harrison, X.A. et al. 2017. Fifty important research questions in microbial ecology. FEMS Microbiology Ecology 93: fix044, https://doi.org/10.1093/femsec/fix044.
Arzoumanian, Z., Holmberg, J. and Norman, B. 2005., An astronomical pattern-matching algorithm for computer-aided identification of whale sharks Rhincodon typus. Journal of Applied Ecology 42: 999–1011, https://doi.org/10.1111/j.1365-2664.2005.01117.x.
Bennun, L., van Bochove, J., Ng, C., et al. 2021. Mitigating Biodiversity Impacts Associated with Solar and Wind Energy Development: Guidelines for Project Developers (Gland, Switzerland: IUCN; Cambridge, UK: The Biodiversity Consultancy), https://doi.org/10.2305/IUCN.CH.2021.04.en.
Berthinussen, A., Richardson, O.C. and Altringham, J.D. 2021. Bat Conservation: Global Evidence for the Effects of Interventions (2021 Edition) (Cambridge: University of Cambridge), https://www.conservationevidence.com/synopsis/pdf/32.
Brown, A., Gilbert, G. and Wotton, S. 2012. Bitterns and bittern conservation in the UK. British Birds 105: 58–87.
Christie, A.P., Amano, T., Martin, P.A., et al. 2021. The challenge of biased evidence in conservation. Conservation Biology 35: 249–62, https://doi.org/10.1111/cobi.13577.
CMP. 2020. Conservation Standards, https://conservationstandards.org/about/.
Community Conservation Horizon Scan Collaboration. 2022. 2021–2022 Global Horizon Scan for Community Conservation: informing future priorities and decision-making (Calgary, Canada: The Wilder Institute/Calgary Zoo), https://cchorizonscan.org/updates-outputs/.
ConservationXLabs. 2022. Grand Challenges for Conservation, https://conservationxlabs.com/grand-challenges.
Crockford, N. 2018. More game-changing good news on coastal wetland conservation — a policy perspective: from Yellow Sea to global conservation OR how an IWSG conference talk can catalyse conservation action… Wader Study 125: 158–61, https://doi.org/10.18194/ws.00128.
Cuthbert, R.J., Taggart, M.A., Prakash, V., et al. 2014. Avian scavengers and the threat from veterinary pharmaceuticals. Philosophical Transactions of the Royal Society B 369: 20130574, https://doi.org/10.1098/rstb.2013.0574.
Daszak, P., Cunningham, A.A. and Hyatt, A.D. 2003. Infectious disease and amphibian population declines. Diversity & Distributions 9: 141–50, https://doi.org/10.1046/j.1472-4642.2003.00016.x.
De Frenne, P., Van Langenhove, L., Van Driessche, A., et al. 2018. Using archived television video footage to quantify phenology responses to climate change. Methods in Ecology and Evolution 9: 1874–82, https://doi.org/10.1111/2041-210X.13024.
Donald, P.F., Green, R.E. and Heath, M.F. 2001. Agricultural intensification and the collapse of Europe’s farmland bird populations. Proceedings of the Royal Society of London B. 268: 25–29, https://doi.org/10.1098/rspb.2000.1325.
EFSA. 2010. Application of systematic review methodology to food and feed safety assessments to support decision making: EFSA guidance for those carrying out systematic reviews. EFSA Journal 8: 1637, https://doi.org/10.2903/j.efsa.2010.1637.
Esmail, N., Wintle, B.C., Sas‐Rolfes, M.T., et al. 2020. Emerging illegal wildlife trade issues: A global horizon scan. Conservation Letters 13: e12715, https://doi.org/10.1111/conl.12715.
Gallardo, B., Zieritz, A., Adriaens, T., et al. 2016. Trans-national horizon scanning for invasive non-native species: A case study in western Europe. Biological Invasions 18: 17–30, https://doi.org/10.1007/s10530-015-0986-0.
Galligan, T.H., Bhusal, K.P., Paudel, K., et al. 2020. Partial recovery of critically endangered Gyps vulture populations in Nepal. Bird Conservation International 30: 87–102, https://doi.org/10.1017/S0959270919000169.
Galligan, T.H., Mallord, J.W., Prakash, V.M., et al. 2021. Trends in the availability of the vulture-toxic drug, diclofenac, and other NSAIDs in South Asia, as revealed by covert pharmacy surveys. Bird Conservation International 31: 337–53, https://doi.org/10.1017/S0959270920000477.
Game, E.T., Meijaard, E., Sheil, D., et al. 2014. Conservation in a wicked complex world; challenges and solutions. Conservation Letters 7: 271–77, https://doi.org/10.1111/conl.12050.
Green, R.E. 1995. Diagnosing causes of bird declines. Ibis 137(Suppl. 1): 47–55, https://doi.org/10.1111/j.1474-919X.1995.tb08457.x.
Green, R.E. 2002. Diagnosing causes of population declines and selecting remedial actions. In: Conserving Bird Biodiversity: General Principles and their Application, Conservation Biology, ed. by K. Norris and D.J. Pain (Cambridge: Cambridge University Press), pp. 139–56, https://doi.org/10.1017/CBO9780511606304.008.
Green, R.E., Pain, D.J. and Krone, O. 2022. The impact of lead poisoning from ammunition sources on raptor populations in Europe. Science of the Total Environment 823: 154017, https://doi.org/10.1016/j.scitotenv.2022.154017.
Green, R.E. and Stowe, T.J. 1993. The decline of the corncrake Crex crex in Britain and Ireland in relation to habitat change. Journal of Applied Ecology 30: 689–95, https://doi.org/10.2307/2404247.
Green, R.E., Taggart, M.A., Senacha, K.R., et al. 2007. Rate of decline of the Oriental white-backed vulture population in India estimated from a survey of diclofenac residues in carcasses of ungulates. PLoS ONE 2: e686, https://doi.org/10.1371/journal.pone.0000686.
Hancock, M.H., Robson, H.J., Smith, T.D., et al. 2020. From a research study to a conservation partnership: Developing approaches for restoring common scoter populations. Aquatic Conservation: Marine and Freshwater Ecosystems 30: 1770–74, https://doi.org/10.1002/aqc.3414.
IPBES. 2016. Summary for Policymakers of the Methodological Assessment of Scenarios and Models of Biodiversity and Ecosystem Services of the Intergovernmental Science-Policy Platform on Biodiversity and Ecosystem Services (IPBES), ed. by S. Ferrier, et al. (Bonn: Secretariat of the Intergovernmental Science-Policy Platform on Biodiversity and Ecosystem Services), https://ipbes.net/sites/default/files/downloads/pdf/SPM_Deliverable_3c.pdf.
IPCC. 2018. Global Warming of 1.5°C. An IPCC Special Report on the impacts of global warming of 1.5°C above pre-industrial levels and related global greenhouse gas emission pathways, in the context of strengthening the global response to the threat of climate change, sustainable development, and efforts to eradicate poverty, ed. by V. Masson-Delmotte, et al. (Cambridge: Cambridge University Press), https://doi.org/10.1017/9781009157940.
IUCN. 2021. The IUCN Red List of Threatened Species. Version 2021–3, https://www.iucnredlist.org.
James, K.L., Randall, N.P. and Haddaway, N.R. 2016. A methodology for systematic mapping in environmental sciences. Environmental Evidence 5: Article 7, https://doi.org/10.1186/s13750-016-0059-6.
Jucker, T., Wintle, B., Shackelford, G., et al. 2018. Ten‐year assessment of the 100 priority questions for global biodiversity conservation. Conservation Biology 32: 1457–63, https://doi.org/10.1111/cobi.13159.
Junker, J., Petrovan, S.O., Arroyo-RodrÍguez, V., et al. 2020. A severe lack of evidence limits effective conservation of the world’s primates. BioScience 70: 794–803, https://doi.org/10.1093/biosci/biaa082.
Kark, S., Sutherland, W.J., Shanas, U., et al. 2016. Priority questions and horizon scanning for conservation: A comparative study. PLoS ONE 11(1): e0145978, https://doi.org/10.1371/journal.pone.0145978.
Kemp, L., Adam, L., Boehm, C.R., et al. 2020. Bioengineering horizon scan 2020. eLife 9: e54489, https://doi.org/10.7554/eLife.54489.
Kemp, L., Aldridge, D.C., Booy, O., et al. 2021. 80 questions for UK biological security. PLoS ONE 16: e.0241190, https://doi.org/10.1371/journal.pone.0241190.
Kennicutt, II, M.C., Chown, S.L., Cassano, J.J., et al. 2015. A roadmap for Antarctic and Southern Ocean science for the next two decades and beyond. Antarctic Science 27: 3–18, https://doi.org/10.1017/S0954102014000674.
Khanyari, M., Milner-Gulland, E.J., Oyanedel, R., et al. 2022. Investigating parasite dynamics of migratory ungulates for sustaining healthy populations: Application to critically-endangered saiga antelopes Saiga tatarica. Biological Conservation 266: 109465, https://doi.org/10.1016/j.biocon.2022.109465.
Kodikara, K.A.S., Mukherjee, N., Jayatissa, L.P., et al. 2017. Have mangrove restoration projects worked? An in‐depth study in Sri Lanka. Restoration Ecology 25: 705–16, https://doi.org/10.1111/rec.12492.
Macinnis-Ng, C., Mcintosh, A.R., Monks, J.M., et al. 2021. Climate-change impacts exacerbate conservation threats in island systems: New Zealand as a case study. Frontiers in Ecology and the Environment 19: 216–24, https://doi.org/10.1002/fee.2285.
MacKinnon, J., Verkuil, Y.I. and Murray, N. 2012. IUCN Situation Analysis on East and Southeast Asian Intertidal Habitats, with Particular Reference to the Yellow Sea (Including the Bohai Sea). Occasional Paper of the IUCN Species Survival Commission No. 047 (Gland, Switzerland; Cambridge: IUCN), https://portals.iucn.org/library/sites/library/files/documents/SSC-OP-047.pdf.
Margoluis, R., Stem, C., Salafsky, N., et al. 2009. Using conceptual models as a planning and evaluation tool in conservation. Evaluation and Program Planning 32: 138–47, https://doi.org/10.1016/j.evalprogplan.2008.09.007.
Margoluis, R., Stem, C., Swaminathan, V., et al. 2013. Results chains: A tool for conservation action design, management, and evaluation. Ecology and Society 18: Article 22, https://doi.org/10.5751/ES-05610-180322.
Mitchell, M., Lockwood, M., Moore, S.A., et al. 2016. Using scenario planning to assess governance reforms for enhancing biodiversity outcomes. Land Use Policy 50: 559–72, https://doi.org/10.1016/j.landusepol.2015.10.020.
Moussy, C., Burfield, I. J., Stephenson, P. J., et al. 2022. A quantitative global review of species population monitoring. Conservation Biology 36: e13721. https://doi.org/10.1111/cobi.13721.
Newman, R.D. and Showler, A.J. 2007. The use of copper rings to reduce losses of red helleborine Cephalanthera rubra to slug and snail herbivory in the Chiltern Hills, Buckinghamshire, England. Conservation Evidence 4: 66–68, https://conservationevidencejournal.com/reference/pdf/2263.
Noss, R.F., 1990. Indicators for monitoring biodiversity: a hierarchical approach. Conservation Biology 4: 355–64, https://doi.org/10.1111/j.1523-1739.1990.tb00309.x.
Oaks, J.L., Gilbert, M., Virani, M.Z., et al. 2004. Diclofenac residues as the cause of vulture population decline in Pakistan. Nature 427: 630–33, https://doi.org/10.1038/nature02317.
Ockendon, N., Thomas, D.H.L., Cortina, J., et al. 2018. One hundred priority questions for landscape restoration in Europe. Biological Conservation 221: 198–208, https://doi.org/10.1016/j.biocon.2018.03.002.
Oldekop, J.A., Fontana, L.B., Grugel, J., et al. 2016. 100 key research questions for the post-2015 development agenda. Development Policy Review 34: 55–82, https://doi.org/10.1111/dpr.12147.
Oldekop, J.A., Vang Rasmussen, L., Agrawal, A., et al. 2020. Forest-linked livelihoods in a globalized world. Nature Plants 6: 1400–07, https://doi.org/10.1038/s41477-020-00814-9.
Pain, D.J., Bowden, C.G.R., Cunningham, A.A., et al. 2008. The race to prevent the extinction of South Asian vultures. Bird Conservation International 18: S30–S48, https://doi.org/10.1017/S0959270908000324.
Palomo, I., Múgica, M., Piñeiro, C., et al. 2017. Envisioning protected areas through participatory scenario planning: Navigating coverage and effectiveness challenges ahead. Parks 23: 29–44, https://doi.org/10.2305/IUCN.CH.2017.PARKS-23-1IP.en.
Parker, M., Acland, A., Armstrong, H.J., et al. 2014. Identifying the science and technology dimensions of emerging public policy issues through horizon scanning. PLoS ONE 9 e96480, https://doi.org/10.1371/journal.pone.0096480.
Parrott, D., Roy, S., Baker, R., et al. 2009. Horizon Scanning for New Invasive Non-Native Animal Species in England. Natural England Commissioned Report NECR009 (Sheffield: Natural England), http://publications.naturalengland.org.uk/publication/43005.
Parsons, E.C.M., Baulch, S., Bechshoft, T., et al. 2015. Key research questions of global importance for cetacean conservation. Endangered Species Research 27: 113–18, https://doi.org/10.3354/esr00655.
Peach, W.J., Denny, M., Cotton, P.A., et al. 2004. Habitat selection by song thrushes in stable and declining farmland populations. Journal of Applied Ecology 41: 275–93, https://doi.org/10.1111/j.0021-8901.2004.00892.x.
Pérez-Jvostov, F., Sutherland, W.J., Barrett, R.D.H., et al. 2019. Horizon scan of conservation issues for inland waters in Canada. Canadian Journal of Fisheries and Aquatic Sciences 77: 869–81, https://doi.org/10.1139/cjfas-2019-0105.
Peterson, G.D., Cumming, G.S. and Carpenter, S.R. 2003. Scenario planning: A tool for conservation in an uncertain world. Conservation Biology 17: 358–66, https://doi.org/10.1046/j.1523-1739.2003.01491.x.
Petrovan, S.O., Aldridge, D.C., Bartlett, H., et al. 2021. Post COVID-19: A solution scan of options for preventing future zoonotic epidemics. Biological Reviews 96: 2694–715. https://doi.org/10.1111/brv.12774.
Pimm, S.L., Jenkins, C.N., Abell, R., et al. 2014. The biodiversity of species and their rates of extinction, distribution, and protection. Science 344: 1246752, https://doi.org/10.1126/science.1246752.
Prakash, V. 1999. Status of vultures in Keoladeo National Park, Bharatpur, Rajasthan, with special reference to population crash in Gyps species. Journal of the Bombay Natural History Society 96: 365–78.
Prescott, G.W., Sutherland, W.J., Aguirre, D., et al. 2017. Political transition and emergent forest-conservation issues in Myanmar. Conservation Biology 31: 1257–70, https://doi.org/10.1111/cobi.13021.
Pretty, J.J., Sutherland, W.J., Ashby, J. et al. 2010. The top 100 questions of importance to the future of global agriculture. International Journal of Agricultural Sustainability 8: 219–36, https://doi.org/10.3763/ijas.2010.0534.
Ricciardi, A., Blackburn, T.M., Carlton, J.T., et al. 2016. Invasion science: A horizon scan of emerging challenges and opportunities. Trends in Ecology and Evolution 32: 464–74, https://doi.org/10.1016/j.tree.2017.03.007.
Ricciardi, A. and MacIsaac, H.J. 2022. Vector control reduces the rate of species invasion in the world’s largest freshwater ecosystem. Conservation Letters 15: e12866, https://doi.org/10.1111/conl.12866.
Riley, S., Siemer, W., Decker, D., et al. 2003. Adaptive Impact Management: An integrative approach to wildlife management. Human Dimensions of Wildlife 8: 81–95, https://doi.org/10.1080/10871200304301.
Roberts, M., Cresswell, W. and Hanley, N. 2018. Prioritising invasive species control actions: Evaluating effectiveness, costs, willingness to pay and social acceptance. Ecological Economics 152: 1–8, https://doi.org/10.1016/j.ecolecon.2018.05.027.
Roura-Pascual, N., Leung, B., Rabitsch, W., et al. 2021. Alternative futures for global biological invasions. Sustainability Science 16: 1637–50, https://doi.org/10.1007/s11625-021-00963-6.
Roy, H., Peyton, J., Aldridge, D.C., et al. 2014. Horizon-scanning for invasive alien species with the potential to threaten biodiversity in Great Britain. Global Change Biology 20: 3859–71, https://doi.org/10.1111/gcb.12603.
Salafsky, N., Irvine, R., Boshoven, J., et al. 2022. A practical approach to assessing existing evidence for specific conservation strategies. Conservation Science and Practice 4: e12654, https://doi.org/10.1111/csp2.12654.
Schwartz, M.W., Cook, C.N., Pressey, R.L. et al. 2018. Decision Support Frameworks and Tools for Conservation. Conservation Letters 11: e12385. https://doi.org/10.1111/conl.12385.
Shultz, S., Baral, H.S., Charman, S., et al. 2004. Diclofenac poisoning is widespread in declining vulture populations across the Indian subcontinent. Proceedings of the Royal Society of London B. (Suppl.) 271: S458–S460, https://doi.org/10.1098/rsbl.2004.0223.
Siriwardena, G.M., Baillie, S.R. and Wilson, J.D. 1999. Temporal variation in annual survival rates of six granivorous birds with contrasting population trends. Ibis 141: 621–36, https://doi.org/10.1111/j.1474-919X.1999.tb07370.x.
Snyder, N. and Snyder, H. 2000. The California Condor: A Saga of Natural History and Conservation (San Diego: Academic Press).
Stephenson, P.J. and Stengel, C., 2020. An inventory of biodiversity data sources for conservation monitoring. PLoS ONE 15: e0242923, https://doi.org/10.1371/journal.pone.0242923.
Stowe, T.J., Newton, A.V., Green, R.E., et al. 1993. The decline of the corncrake Crex crex in Britain and Ireland in relation to habitat. Journal of Applied Ecology 30: 53–62, https://doi.org/10.2307/2404270.
Summers, R.W., Green, R.E., Proctor, D., et al. 2004. An experimental study of the effects of predation on the breeding productivity of capercaillie and black grouse. Journal of Applied Ecology 41: 513–25, https://doi.org/10.1111/j.0021-8901.2004.00891.x.
Sutherland, W.J. 2000. The Conservation Handbook. Research, Management and Policy (Oxford: Wiley-Blackwell).
Sutherland, W.J. 2019. Kaizen conservation? Oryx 53: 397–98, https://doi.org/10.1017/S0030605319000619.
Sutherland, W.J., Adams, W.M., Aronson, R.B., et al. 2009. One hundred questions of importance to the conservation of global biological diversity. Conservation Biology 23: 557–67, https://doi.org/10.1111/j.1523-1739.2009.01212.x.
Sutherland, W.J., Albon, S.D., Allison, H., et al. 2010a. The identification of priority opportunities for UK nature conservation policy. Journal of Applied Ecology 47: 955–65, https://doi.org/10.1111/j.1365-2664.2010.01863.x.
Sutherland, W.J., Allison, H., Aveling, R., et al. 2012a. Enhancing the value of horizon scanning through collaborative review. Oryx 46: 368–74, https://doi.org/10.1017/S0030605311001724.
Sutherland, W.J., Alves, J.A., Amano, T., et al. 2012b. A horizon scanning assessment of current and potential future threats to migratory shorebirds. Ibis 154: 663–79, https://doi.org/10.1111/j.1474-919X.2012.01261.x.
Sutherland, W.J., Armstrong-Brown, S., Armsworth, P.R., et al. 2006. The identification of 100 ecological questions of high policy relevance in the UK. Journal of Applied Ecology 43: 617–27, https://doi.org/10.1111/j.1365-2664.2006.01188.x.
Sutherland, W.J., Atkinson, P.W., Butchart, S.H.M., et al. 2022. A horizon scan of global biological conservation issues for 2022. Trends in Ecology and Evolution 37: 95–104, https://doi.org/10.1016/j.tree.2021.10.014.
Sutherland, W.J., Clout, M., Côté, I.M., et al. 2010b. A horizon scan of global conservation issues for 2010. Trends in Ecology and Evolution 25: 1–7, https://doi.org/10.1016/j.tree.2009.10.003.
Sutherland, W.J., Dicks, L.V., Petrovan, S.O., et al. (eds.). 2021. What Works in Conservation 2021 (Cambridge: Open Book Publishers), https://doi.org/10.11647/obp.0267.
Sutherland, W.J., Fleishman, E., Clout, M., et al. 2019a. Ten years on: A review of the first global conservation horizon scan. Trends in Ecology and Evolution 34: 139–53, https://doi.org/10.1016/j.tree.2018.12.003.
Sutherland, W.J., Fleishman, E., Mascia, M.B., et al. 2011. Methods for collaboratively identifying research priorities and emerging issues in science and policy. Methods in Ecology and Evolution 2: 238–47, https://doi.org/10.1111/j.2041-210X.2010.00083.x.
Sutherland, W.J., Gardner, T., Bogich, T.L., et al. 2014. Solution scanning as a key policy tool: Identifying management interventions to help maintain and enhance regulating ecosystem services. Ecology and Society 19: Article 3, https://doi.org/10.5751/ES-06082-190203.
Sutherland, W.J., Goulden, C., Bell, K., et al. 2013. 100 questions: identifying research priorities for poverty prevention and reduction. Journal of Poverty and Social Justice 21: 189–205, https://doi.org/10.1332/175982713X671210.
Sutherland, W.J., Taylor, N.G., MacFarlane, D., et al. 2019b. Building a tool to overcome barriers in research-implementation spaces: The Conservation Evidence database. Biological Conservation 238: 108199, https://doi.org/10.1016/j.biocon.2019.108199.
Sutherland, W.J. and Woodroof, H.J. 2009. The need for environmental horizon scanning. Trends in Ecology and Evolution 24: 523–27, https://doi.org/10.1016/j.tree.2009.04.008.
Sutherland, W.J. and Wordley, C.F. 2017. Evidence complacency hampers conservation. Nature Ecology and Evolution 1: 1215–16, https://doi.org/10.1038/s41559-017-0244-1.
Swan G.E., Cuthbert, R., Quevedo, M., et al. 2006. Toxicity of diclofenac to Gyps vultures. Biology Letters 2: 279–82, https://doi.org/10.1098/rsbl.2005.0425.
Taleb, N. 2007. The Black Swan: The Impact of the Highly Improbable (New York: Random House).
Tatayah, R.V.V., Malham, J. and Haverson, P. 2007. The use of copper strips to exclude invasive African giant land-snails Achatina spp. from echo parakeet Psittacula eques nest cavities, Black River Gorges National Park, Mauritius. Conservation Evidence 4: 6–8, https://conservationevidencejournal.com/reference/pdf/2245.
Taylor, N.G., Grillas, P., Al Hreisha, H., et al. 2021. The future for Mediterranean wetlands: 50 key issues and 50 important conservation research questions. Regional Environmental Change 21: Article 33, https://doi.org/10.1007/s10113-020-01743-1.
Thomas, J.A., Simcox, D.J. and Clarke, R.T. 2009. Successful conservation of a threatened Maculinea butterfly. Science 325: 80–83, https://doi.org/10.1126/science.1175726.
Thomas, J.A., Simcox, D.J. and Hovestadt, T. 2011. Evidence based conservation of butterflies. Journal of Insect Conservation 15: 241–48, https://doi.org/10.1007/s10841-010-9341-z.
Thomas, J.A., Thomas, C.D., Simcox, D.J., et al. 1986. Ecology and declining status of the silver-spotted skipper butterfly (Hesperia comma) in Britain. Journal of Applied Ecology 23: 365–80, https://doi.org/10.2307/2404023.
UK Government Office for Science. 2017. The Futures Toolkit: Tools for Futures Thinking and Foresight Across UK Government, Edition 1.0 (London: Government Office for Science), https://assets.publishing.service.gov.uk/government/uploads/system/uploads/attachment_data/file/674209/futures-toolkit-edition-1.pdf.
USAID. 2018. Evidence in Action: Using and Generating Evidence About Effectiveness in Biodiversity Programming. Unit 1: Understanding an Evidence-Based Approach (Washington DC: USAID Bureau for Economic Growth, Education, and the Environment Office of Forestry and Biodiversity), https://pdf.usaid.gov/pdf_docs/PA00SXVJ.pdf.
Walsh, J.C., Dicks, L.V. and Sutherland, W.J. 2015. The effect of scientific evidence on conservation practitioners’ management decisions. Conservation Biology 29: 88–98, https://doi.org/10.1111/cobi.12370.
Wintle, B., Boehm, C., Rhodes, C., et al. 2017. A transatlantic perspective on 20 emerging issues in biological engineering. e-Life 6: e30247, https://doi.org/10.7554/eLife.30247.
Wintle, B.C., Kennicutt, M.C. and Sutherland, W.J. 2020. Scanning horizons in research, policy and practice. In: Conservation Research, Policy and Practice, Ecological Reviews, ed. by W.J. Sutherland, et al. (Cambridge: Cambridge University Press), pp. 29–47, https://doi.org/10.1017/9781108638210.003.
Zoohackathon. 2021. Zoohackathon, https://www.state.gov/zoohackathon-2021/.